Revision Notes for CBSE Class 12 Chemistry Chapter 12 (Amines) - Free PDF Download
FAQs on Class 12 Chemistry Revision Notes for Chapter 12 - Amines - Free PDF Download
1. What is Diazotization?
Diazotization is a process where the Benzene diazonium chloride is prepared when there is a reaction of aniline with the nitrous acid. This occurs at 273 to 278 K. This conversion of primary aromatic amines into diazonium salt is known as Diazotization.
2. What are Amines Derived From?
Amines are derived from the ammonia acquired when the hydrogen is replaced with aryl or the alkyl groups. When one of the hydrogen atoms gets replaced, it forms a primary amine.
3. What are Simple Amines?
In case the aryl or the alkyl group of the tertiary and the secondary amines are the same, then this is known as the simple amines. If these are attached to various groups, then these are called mixed amines. Amines have one pair of unshared electron pair on the nitrogen atom. This then behaves like the Lewis bases. These get formed with amines, halides, imides, or nitro compounds.
4. What is the Gatterman Reaction?
Gatterman reaction is a reaction where the bromine or the chlorine gets introduced in the benzene ring. The diazonium salt solution treats this while it corresponds to the halogen acid in the presence of a copper powder.
5. What are Amines in Chemistry class 12?
Amines is an interesting and vital chapter in Chemistry in Class 12. This chapter gives an in-depth explanation of Amines. Amines are derivatives of the organic compound ammonia. Amines are classified into different types based on the replacement of hydrogen atoms. The chapters analyze everything related to amines, their preparation, chemical and physical properties, reactions of amines with other organic compounds. To understand the chapter better, you can refer to the revision notes of Class 12 Chemistry Chapter 12.
6. What are Amines? How are they classified?
Amines are organic compounds that are derived from another organic compound, ammonia. They are crucial for living organisms as it helps in bioregulation, neurotransmission, etc. Amines can be classified into three different categories which are as follows:
Primary Amines (1°) - When one hydrogen atom is replaced by an alkyl or aryl group.
Secondary Amines (2°) - When two hydrogen atoms are replaced by an alkyl or aryl.
Tertiary Amines (3°) - When three hydrogen atoms are replaced by an alkyl or aryl group.
7. What are the chemical properties of Amines?
Amines possess some really important chemical properties which are as follows:
Amines behave as Lewis base. The reason why they do so is that there is a pair of unshared electrons over the nitrogen atom.
Amines are basic in nature and when it is reacted with an acid, it gives salt as a product.
Amines show solubility with water whereas, in organic solvents, they are insoluble.
Because of the presence of the two pairs of unshared electrons, they also behave as nucleophiles.
8. How can I understand Amines in Class 12?
Chapter 12 ‘Amines’ of Chemistry of Class 12 is a very complex chapter. If you don't pay enough attention, it will be difficult for you to understand the chapters. You can expect a few questions from this chapter in your exams. This chapter holds an important place in competitive exams like JEE and NEET. Therefore, to understand the entire chapter, you should start with the NCERT textbook. The concepts explained in NCERT will make the whole chapter easy for you.
9. How can the revision notes of Amines help me in exams?
Amines is considered to be a significant chapter for Class 12. To be well-prepared, you can go through the revision notes provided on the website of Vedantu. These notes will clear your concepts and will thorough your knowledge on Amines. You can go through these notes to revise your concepts before exams. These notes are written in brief but to the point. So, you can use these points to fetch extra marks. You can also access the revision notes on the Vedantu app. All the resources are available free of cost.
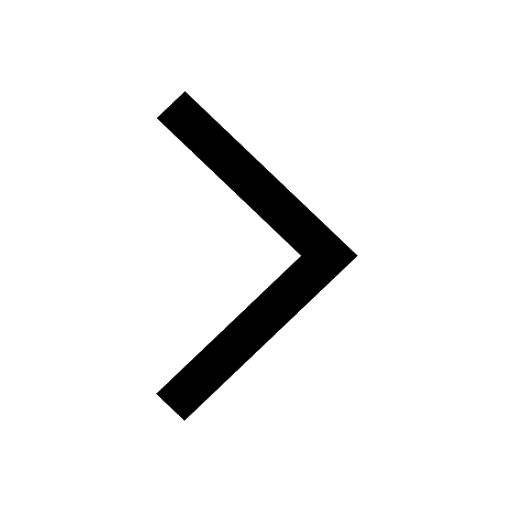
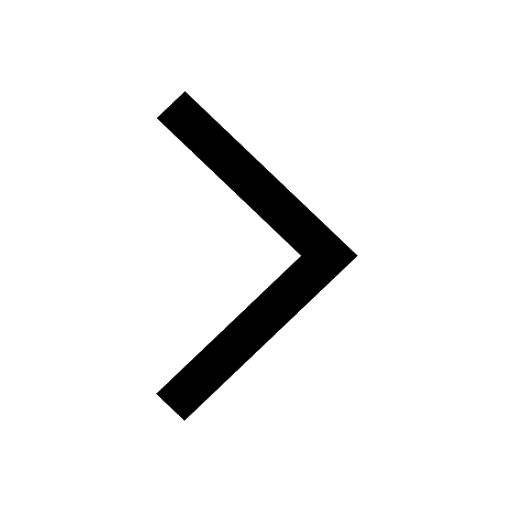
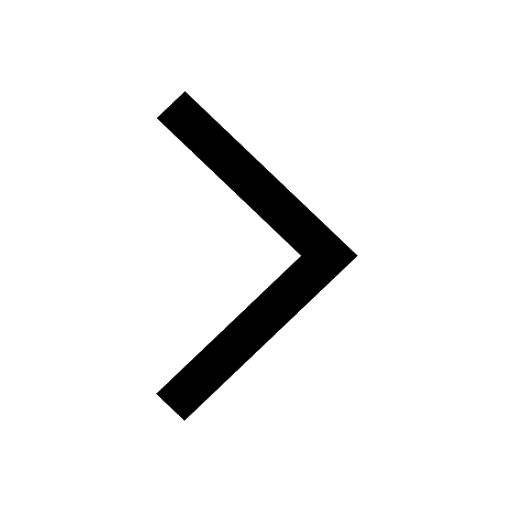
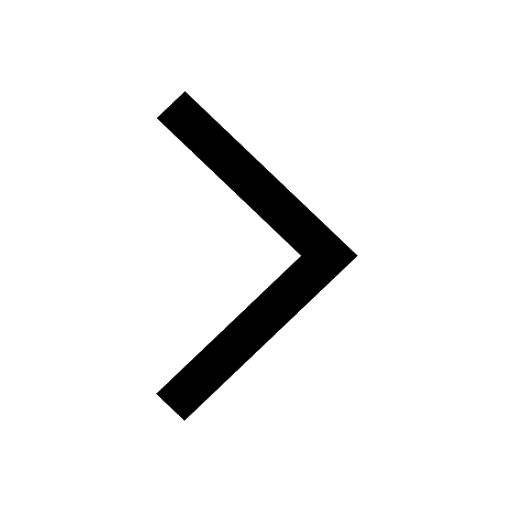
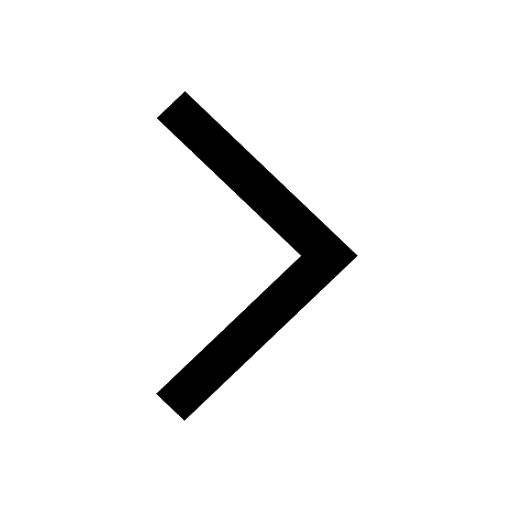
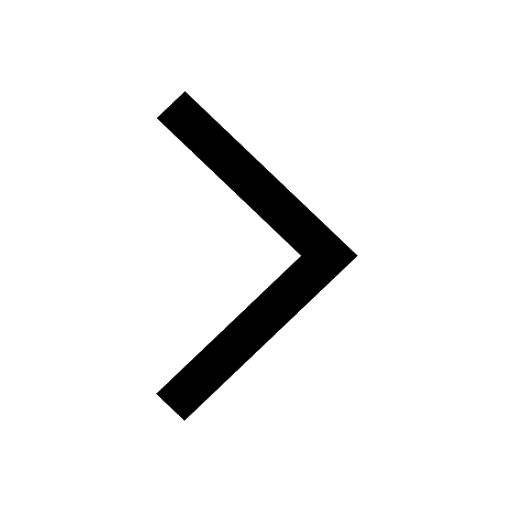
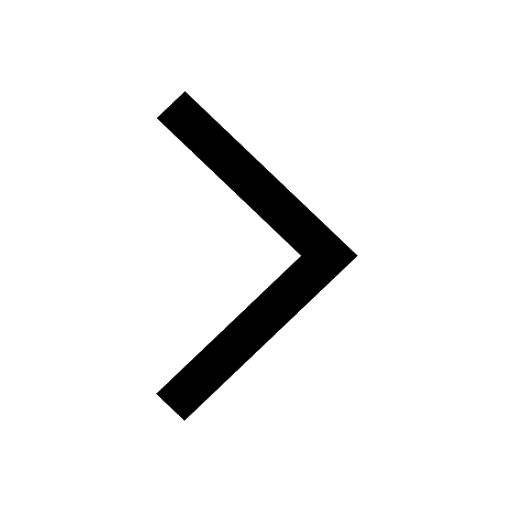
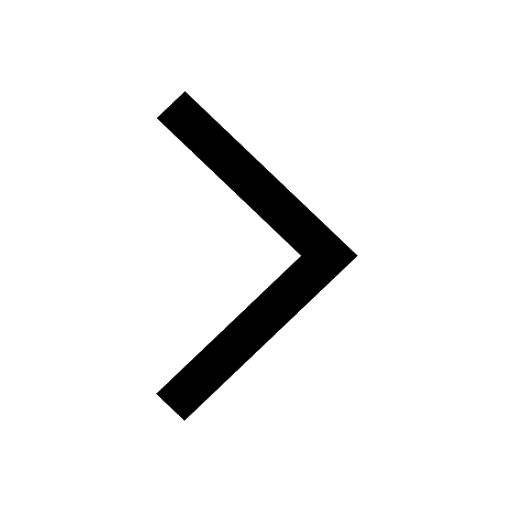
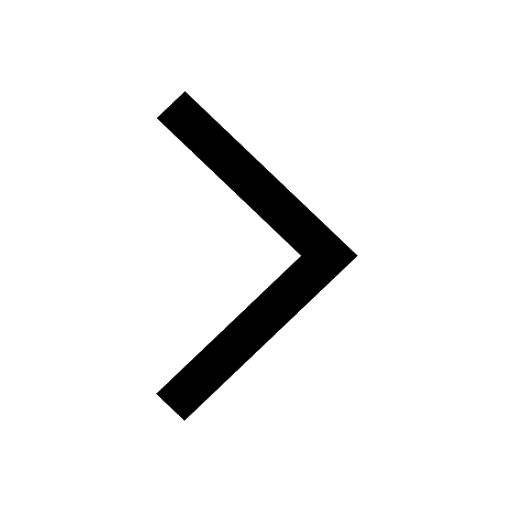
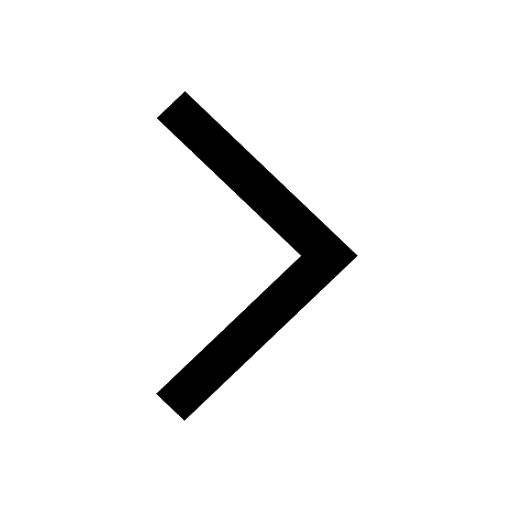
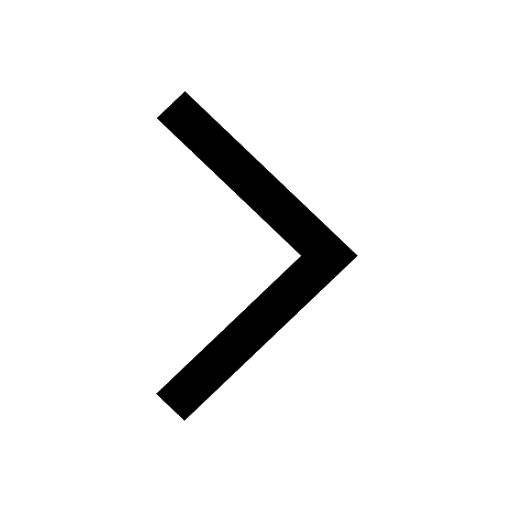
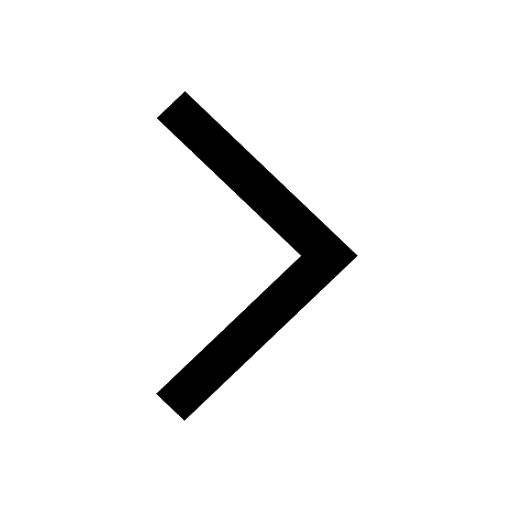
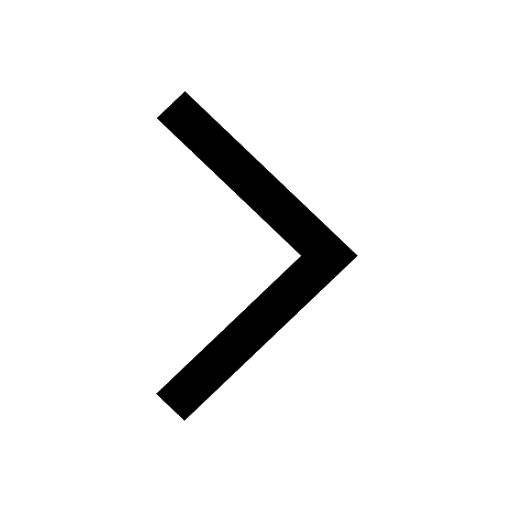
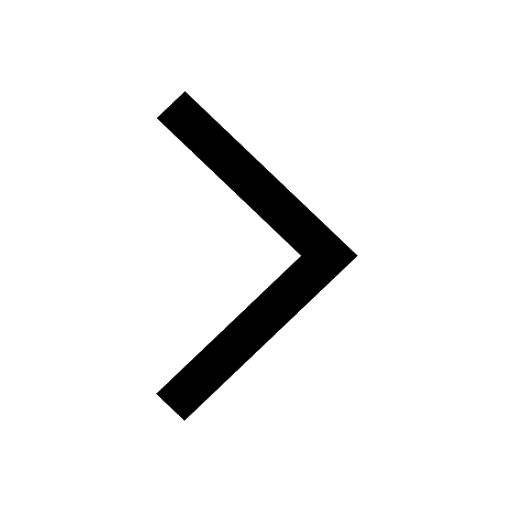
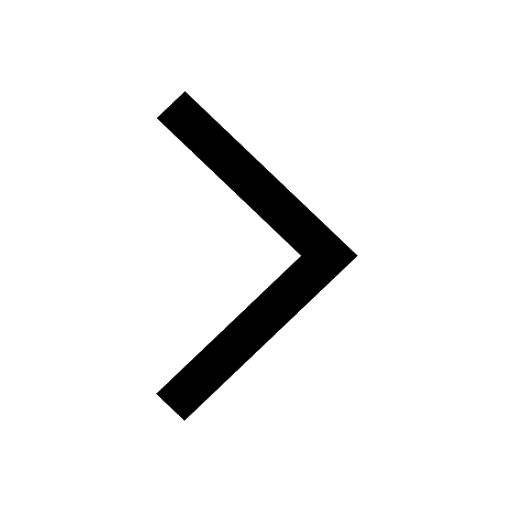
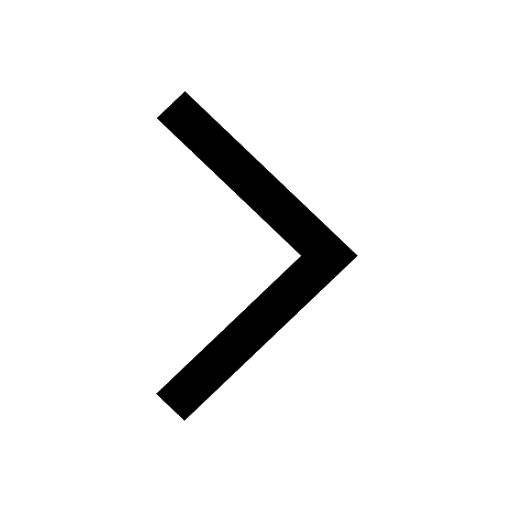
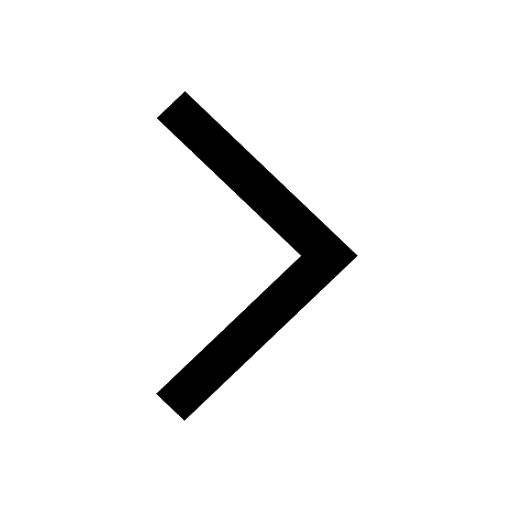
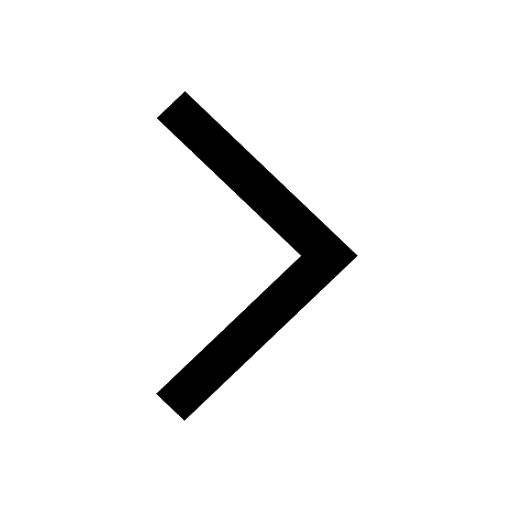
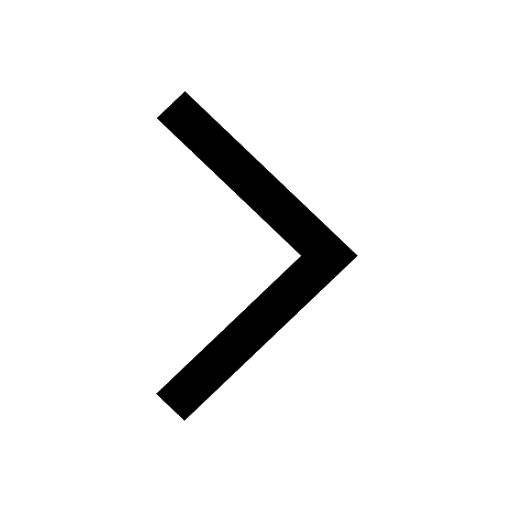
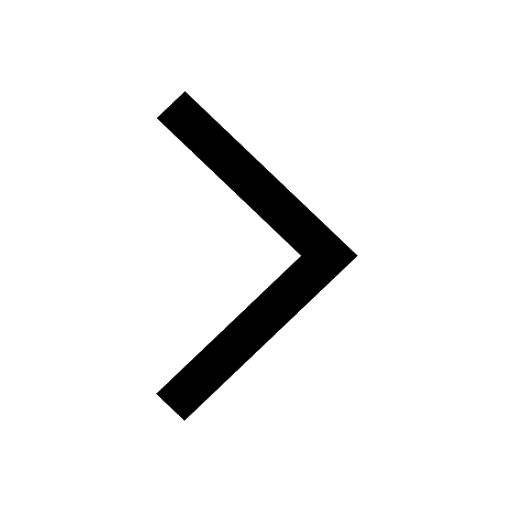
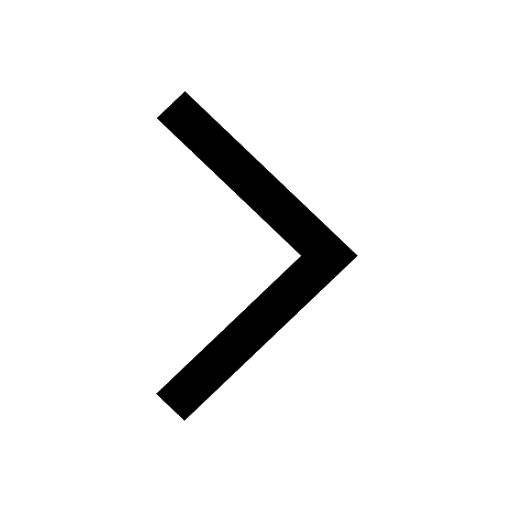