Class 12 Maths Chapter 7 Integrals NCERT Solutions - Free PDF Download
NCERT Solutions for Class 12 Mathematics, Chapter 7 Integrals were created by subject matter experts at Vedantu to assist students with their board exam preparation. Chapter 7 of the NCERT Maths Book for Class 12 covers the idea of integrals. This chapter of the NCERT Solutions for Class 12 Maths teaches students about integral calculus (definite and indefinite), its properties, and much more. The topic is crucial for both the CBSE board test and competitive exams. These NCERT Solutions for Class 12 Maths integrals are quite easy and can help students quickly grasp the problem-solving process. Students may access these NCERT Solutions for Class 12 Maths Chapter 7 and download them for free to practise offline as well. Access the latest Class 12 Maths Syllabus here.

- 4.1Exercise 7.1: This exercise introduces the concept of indefinite integrals and teaches students how to find them.Exercise 7.2: This exercise deals with the properties of indefinite integrals and helps students understand how to use these properties to solve problems.Exercise 7.3: This exercise covers the concept of definite integrals and teaches students how to evaluate them using different methods such as the limit definition of definite integrals and the properties of definite integrals.Exercise 7.4: This exercise teaches students how to evaluate definite integrals using substitution.Exercise 7.5: This exercise deals with integration by parts and teaches students how to solve problems using this method.Exercise 7.6: This exercise covers the concept of partial fractions and helps students understand how to use them to evaluate integrals.Exercise 7.7: This exercise teaches students how to evaluate integrals using trigonometric identities.Exercise 7.8: This exercise covers the concept of integration using trigonometric substitutions and helps students understand how to use this method to solve problems.Exercise 7.9: This exercise teaches students how to evaluate integrals using the method of differentiation under the integral sign.Exercise 7.10: This exercise deals with the concept of improper integrals and teaches students how to evaluate them.Miscellaneous Exercise: This exercise covers various miscellaneous integrals and teaches students how to solve problems using different methods. This exercise contains a collection of problems that require students to apply the concepts they have learned in the previous exercises.Access NCERT Solutions for Class 12 Mathematics Chapter 7- Integrals
- 5.1Exercise 7.1
- 5.2Exercise 7.2
- 5.3Exercise 7.3
- 5.4Exercise 7.4
- 5.5Exercise 7.5
- 5.6Exercise 7.6
- 5.7Exercise 7.7
- 5.8Exercise 7.8
- 5.9Exercise 7.9
- 5.10Exercise 7.10
- 5.11Miscellaneous Exercise
- 6.17.1 Introduction
- 6.27.2 Integration as a Reverse Process of Differentiation
- 6.37.3 Methods of Integration
- 6.47.4 Integrals of Some Particular Functions
- 6.57.5 Integration by Partial Functions
- 6.67.6 Integration by Parts
- 6.77.7 Definite Integral
- 6.87.8 Fundamental Theorem of Calculus
- 6.97.9 Evaluation of Definite Integrals by Substitution
- 6.107.10 Some Properties of Definite Integral
Glance on Integration for Class 12 Chapter 7 Maths
Integration helps you find the exact area under that curve.
This chapter covers various formulas and techniques to solve area problems.
You can find questions based on the general area (indefinite integrals) and the exact area (definite integrals).
Special methods are used to solve tricky integral problems like substitution or trigonometric identities.
This article contains chapter notes and important questions for Chapter 7 Integration.
There are exercise links provided. It has solutions for each question, from Integration as an Inverse Process of Differentiation, Methods of Integration, Integrals of Some Particular Functions, Definite Integrals, Application of Integrals, and Integration using Trigonometric Identities.
There are ten exercises and miscellaneous exercise (240 fully solved questions) in class 12 maths Ch 7 Integrals.
Access Exercise wise NCERT Solutions for Chapter 7 Maths Class 12
S.No. | Current Syllabus Exercises of Class 12 Maths Chapter 7 | |
1 | ||
2 | ||
3 | ||
4 | ||
5 | ||
6 | ||
7 | ||
8 | ||
9 | ||
10 | ||
11 | Class 12 Maths NCERT Solutions of Integrals Miscellaneous Exercise |
Exercises Under NCERT Class 12 Maths Chapter 7 – Integrals
Exercise 7.1: This exercise introduces the concept of indefinite integrals and teaches students how to find them.
Exercise 7.2: This exercise deals with the properties of indefinite integrals and helps students understand how to use these properties to solve problems.
Exercise 7.3: This exercise covers the concept of definite integrals and teaches students how to evaluate them using different methods such as the limit definition of definite integrals and the properties of definite integrals.
Exercise 7.4: This exercise teaches students how to evaluate definite integrals using substitution.
Exercise 7.5: This exercise deals with integration by parts and teaches students how to solve problems using this method.
Exercise 7.6: This exercise covers the concept of partial fractions and helps students understand how to use them to evaluate integrals.
Exercise 7.7: This exercise teaches students how to evaluate integrals using trigonometric identities.
Exercise 7.8: This exercise covers the concept of integration using trigonometric substitutions and helps students understand how to use this method to solve problems.
Exercise 7.9: This exercise teaches students how to evaluate integrals using the method of differentiation under the integral sign.
Exercise 7.10: This exercise deals with the concept of improper integrals and teaches students how to evaluate them.
Miscellaneous Exercise: This exercise covers various miscellaneous integrals and teaches students how to solve problems using different methods. This exercise contains a collection of problems that require students to apply the concepts they have learned in the previous exercises.
Access NCERT Solutions for Class 12 Mathematics Chapter 7- Integrals
Exercise 7.1: This exercise introduces the concept of indefinite integrals and teaches students how to find them.
Exercise 7.2: This exercise deals with the properties of indefinite integrals and helps students understand how to use these properties to solve problems.
Exercise 7.3: This exercise covers the concept of definite integrals and teaches students how to evaluate them using different methods such as the limit definition of definite integrals and the properties of definite integrals.
Exercise 7.4: This exercise teaches students how to evaluate definite integrals using substitution.
Exercise 7.5: This exercise deals with integration by parts and teaches students how to solve problems using this method.
Exercise 7.6: This exercise covers the concept of partial fractions and helps students understand how to use them to evaluate integrals.
Exercise 7.7: This exercise teaches students how to evaluate integrals using trigonometric identities.
Exercise 7.8: This exercise covers the concept of integration using trigonometric substitutions and helps students understand how to use this method to solve problems.
Exercise 7.9: This exercise teaches students how to evaluate integrals using the method of differentiation under the integral sign.
Exercise 7.10: This exercise deals with the concept of improper integrals and teaches students how to evaluate them.
Miscellaneous Exercise: This exercise covers various miscellaneous integrals and teaches students how to solve problems using different methods. This exercise contains a collection of problems that require students to apply the concepts they have learned in the previous exercises.
Exercise 7.1
1. Find an antiderivative (or integral) of the following functions by the method of inspection. sin 2x
Ans: We use the method of inspection as follows:
$ \dfrac{d}{dx}\left( \cos 2x \right)=-2\sin 2x\Rightarrow -\dfrac{1}{2}\dfrac{d}{dx}\left( \cos 2x \right) $
$\therefore \sin 2x=\dfrac{d}{dx}\left( -\dfrac{1}{2}\cos 2x \right)$
Thus, the anti-derivative of sin $2x$is $-\dfrac{1}{2}\cos 2x$.
2. Find an antiderivative (or integral) of the following functions by the method of inspection. cos 3x
Ans: We use the method of inspection as follows:
$\dfrac{d}{dx}\left( \sin 3x \right)=3\cos 3x\Rightarrow \dfrac{1}{3}\dfrac{d}{dx}\left( \sin 3x \right)$
$ \therefore \cos 3x=\dfrac{d}{dx}\left( \dfrac{1}{3}\sin 3x \right)$
Thus, the anti - derivative of cos $3x$is $\dfrac{1}{3}\sin 3x$.
3. Find an antiderivative (or integral) of the following functions by the method of inspection. $\mathbf{{{e}^{2x}}}$
Ans: We use the method of inspection as follows:
$ \dfrac{d}{dx}\left( {{e}^{2x}} \right)\Rightarrow 2{{e}^{2x}}=\dfrac{1}{2}\dfrac{d}{dx}\left( {{e}^{2x}} \right) $
$ \therefore {{e}^{2x}}=\dfrac{d}{dx}\left( \dfrac{1}{2}{{e}^{2x}} \right) $
Thus, the anti-derivative of ${{e}^{2x}}$is $\dfrac{1}{2}{{e}^{2x}}$.
4. Find an antiderivative (or integral) of the following functions by the method of inspection.$\mathbf{{{\left( ax+b \right)}^{2}}}$
Ans: We use the method of inspection as follows:
$ \dfrac{d}{dx}{{\left( ax+b \right)}^{3}}=3a{{\left( ax+b \right)}^{2}} $
$ \Rightarrow {{\left( ax+b \right)}^{2}}=\dfrac{1}{3a}\dfrac{d}{dx}{{\left( ax+b \right)}^{3}} $
$ \therefore {{\left( ax+b \right)}^{2}}=\dfrac{d}{dx}\left( \dfrac{1}{3a}{{\left( ax+b \right)}^{3}} \right)$
Thus, the anti-derivative of ${{\left( ax+b \right)}^{2}}$ is $\dfrac{1}{3a}{{\left( ax+b \right)}^{3}}$.
5. Find an antiderivative (or integral) of the following functions by the method of inspection. sin $\mathbf{2x-4{{e}^{3x}}}$
Ans: We use the method of inspection as follows:
$\dfrac{d}{dx}\left( -\dfrac{1}{2}\cos 2x-\dfrac{4}{3}{{e}^{3x}} \right)=\left( \sin 2x-4{{e}^{3x}} \right)$
Thus, the anti-derivative of $\left( \sin 2x-4{{e}^{3x}} \right)$ is $\left( -\dfrac{1}{2}\cos 2x-\dfrac{4}{3}{{e}^{3x}} \right)$.
6. $\int{\left( 4{{e}^{3x}}+1 \right)dx}$
Ans:
$ \int{\left( 4{{e}^{3x}}+1 \right)dx} $
$ =4\int{{{e}^{3x}}dx}+\int{1}dx $
$ =4\left( \dfrac{{{e}^{3x}}}{3} \right)+x+C $
$=\dfrac{4}{3}{{e}^{3x}}+x+C$
7. $\int{{{x}^{2}}\left( 1-\dfrac{1}{{{x}^{2}}} \right)dx}$
Ans: $ \int{{{x}^{2}}\left( 1-\dfrac{1}{{{x}^{2}}} \right)dx} $
$=\int{\left( {{x}^{2}}-1 \right)dx} $
$ =\dfrac{{{x}^{3}}}{3}-x+C$
8. $\int{\left( a{{x}^{2}}+bx+c \right)dx}$
Ans:$ \int{\left( a{{x}^{2}}+bx+c \right)}dx $
$ =a\int{{{x}^{2}}dx+b\int{xdx+c\int{1.dx}}}$
$=a\left( \dfrac{{{x}^{3}}}{3} \right)+b\left( \dfrac{{{x}^{2}}}{2} \right)+cx+C $
9. $\int{\left( 2{{x}^{2}}+{{e}^{x}} \right)dx}$
Ans: $\int{\left( 2{{x}^{2}}+{{e}^{x}} \right)dx} $
$ =2\int{{{x}^{2}}dx+\int{{{e}^{x}}dx}} $
$ =2\left( \dfrac{{{x}^{3}}}{3} \right)+{{e}^{x}}+C$
$=\dfrac{2}{3}{{x}^{3}}+{{e}^{x}}+C $
10. $\int{{{\left( \sqrt{x}-\dfrac{1}{\sqrt{x}} \right)}^{2}}dx}$
Ans:$ \int{{{\left( \sqrt{x}-\dfrac{1}{\sqrt{x}} \right)}^{2}}}dx $
$ =\int{\left( x+\dfrac{1}{x}-2 \right)dx} $
$ =\int{xdx}+\int{\dfrac{1}{x}dx}-2\int{1.dx} $
$=\dfrac{{{x}^{2}}}{2}+\log \left| x \right|-2x+C $
11. $\int{\dfrac{{{x}^{3}}+5{{x}^{2}}-4}{{{x}^{2}}}dx}$
Ans: $\int{\dfrac{{{x}^{3}}+5{{x}^{2}}-4}{{{x}^{2}}}dx}$
\[ \int{\left( x+5-4{{x}^{-2}} \right)dx} \]
\[ =\int{xdx}+5\int{1.dx}-4\int{{{x}^{-2}}dx}\]
\[=\dfrac{{{x}^{2}}}{2}+5x+\dfrac{4}{x}+C \]
12. $\int{\dfrac{{{x}^{3}}+3x+4}{\sqrt{x}}dx}$
Ans:$\int{\dfrac{{{x}^{3}}+3x+4}{\sqrt{x}}dx}$
$ =\int{\left( {{x}^{\dfrac{5}{2}}}+3{{x}^{\dfrac{1}{2}}}+4{{x}^{-\dfrac{1}{2}}} \right)dx} $
$ =\dfrac{{{x}^{\dfrac{7}{2}}}}{\dfrac{7}{2}}+\dfrac{3\left( {{x}^{\dfrac{3}{2}}} \right)}{\dfrac{3}{2}}+\dfrac{4\left( {{x}^{\dfrac{1}{2}}} \right)}{\dfrac{1}{2}}+C$
$ =\dfrac{2}{7}{{x}^{\dfrac{7}{2}}}+2{{x}^{\dfrac{3}{2}}}+8\sqrt{x}+C$
13. $\int{\dfrac{{{x}^{3}}-{{x}^{2}}+x-1}{x-1}dx}$
Ans: $\int{\dfrac{{{x}^{3}}-{{x}^{2}}+x-1}{x-1}dx}$
We obtain, on dividing:
$ =\int{\left( {{x}^{2}}+1 \right)dx} $
$ =\int{{{x}^{2}}dx}+\int{1.dx} $
$ =\dfrac{{{x}^{3}}}{3}+x+C$
14. $\int{\left( 1-x \right)}\sqrt{x}dx$
Ans: $\int{\left( 1-x \right)}\sqrt{x}dx$
$=\int{\left( \sqrt{x}-{{x}^{\dfrac{3}{2}}} \right)dx} $
$ =\int{{{x}^{\dfrac{1}{2}}}dx-\int{{{x}^{\dfrac{3}{2}}}dx}} $
$=\dfrac{2}{3}{{x}^{\dfrac{3}{2}}}-\dfrac{2}{5}{{x}^{\dfrac{5}{2}}}+C $
15. $\int{\sqrt{x}\left( 3{{x}^{2}}+2x+3 \right)dx}$
Ans: $\int{\sqrt{x}\left( 3{{x}^{2}}+2x+3 \right)dx}$
$ =3\int{\left( 2{{x}^{\dfrac{5}{2}}}+2{{x}^{\dfrac{3}{2}}}+3{{x}^{\dfrac{1}{2}}} \right)} $
$=3\int{{{x}^{\dfrac{5}{2}}}dx+2\int{{{x}^{\dfrac{3}{2}}}dx+3\int{{{x}^{\dfrac{1}{2}}}}dx}} $
$=\dfrac{6}{7}{{x}^{\dfrac{7}{2}}}+\dfrac{4}{5}{{x}^{\dfrac{5}{2}}}+2{{x}^{\dfrac{3}{2}}}+C $
16. $\int{\left( 2x-3\cos x+{{e}^{x}} \right)dx}$
Ans: $\int{\left( 2x-3\cos x+{{e}^{x}} \right)dx}$
\[ =2\int{xdx-3\int{\cos xdx}+\int{{{e}^{x}}dx}} \]
\[=\dfrac{2{{x}^{2}}}{2}-3\left( \sin x \right)+{{e}^{x}}+C \]
\[ ={{x}^{2}}-3\sin x+{{e}^{x}}+C\]
17. $\int{\left( 2{{x}^{2}}-3\sin x+5\sqrt{x} \right)}dx$
Ans: $\int{\left( 2{{x}^{2}}-3\sin x+5\sqrt{x} \right)}dx$
=\[ 2\int{{{x}^{2}}dx-3\int{\sin xdx+5\int{{{x}^{\dfrac{1}{2}}}}dx}}\]
= \[ \dfrac{2{{x}^{3}}}{3}-3\left( -\cos x \right)+5\left(\dfrac{{{x}^{\dfrac{3}{2}}}}{\dfrac{3}{2}} \right)+C\]
=$ \dfrac{2}{3}{{x}^{3}}+3\cos x+\dfrac{10}{3}{{x}^{\dfrac{3}{2}}}+C $
18. $\int{\sec x\left( \sec x+\tan x \right)dx}$
Ans: $\int{\sec x\left( \sec x+\tan x \right)dx}$
$ =\int{\left( {{\sec }^{2}}x+\sec x\tan x \right)dx}$
$ =\int{{{\sec }^{2}}xdx+\int{\sec x\tan xdx}}$
$ =\tan x+\sec x+C $
19. $\int{\dfrac{{{\sec }^{2}}x}{\cos e{{c}^{2}}x}dx}$
Ans: $\int{\dfrac{{{\sec }^{2}}x}{\cos e{{c}^{2}}x}dx}$
$ =\int{\dfrac{\dfrac{1}{{{\cos }^{2}}x}}{\dfrac{1}{{{\sin }^{2}}x}}dx} $
$=\int{\dfrac{{{\sin }^{2}}x}{{{\cos }^{2}}x}dx}$
$=\int{{{\tan }^{2}}xdx}$
$ =\int{{{\sec }^{2}}xdx}-\int{1dx} $
$=\tan x-x+C $
20. $\int{\dfrac{2-3\sin x}{{{\cos }^{2}}x}dx}$
Ans: $\int{\dfrac{2-3\sin x}{{{\cos }^{2}}x}dx}$
$ =\int{\left( \dfrac{2}{{{\cos }^{2}}x}-\dfrac{3\sin x}{{{\cos }^{2}}x} \right)dx} $
$ =\int{2{{\sec }^{2}}xdx}-3\int{\tan x\sec xdx} $
$=2\tan x-3\sec x+C$
21. The anti – derivative of $\left( \sqrt{x}+\dfrac{1}{\sqrt{x}} \right)$ equals
- $\dfrac{1}{3}{{x}^{\dfrac{1}{3}}}+2{{x}^{\dfrac{1}{2}}}+C$
- $\dfrac{2}{3}{{x}^{\dfrac{2}{3}}}+\dfrac{1}{2}{{x}^{2}}+C$
- $\dfrac{2}{3}{{x}^{\dfrac{3}{2}}}+2{{x}^{\dfrac{1}{2}}}+C$
- $\dfrac{3}{2}{{x}^{\dfrac{3}{2}}}+\dfrac{1}{2}{{x}^{\dfrac{1}{2}}}+C$
Ans:
$ \left( \sqrt{x}+\dfrac{1}{\sqrt{x}} \right) $
$=\int{{{x}^{\dfrac{1}{2}}}dx}+\int{{{x}^{-\dfrac{1}{2}}}}dx=\dfrac{{{x}^{\dfrac{3}{2}}}}{\dfrac{3}{2}}+\dfrac{{{x}^{\dfrac{1}{2}}}}{\dfrac{1}{2}}+C $
$=\dfrac{2}{3}{{x}^{\dfrac{3}{2}}}+2{{x}^{\dfrac{1}{2}}}+C $
Thus, the correct answer is C.
22. If $\dfrac{d}{dx}f\left( x \right)=4{{x}^{3}}-\dfrac{3}{{{x}^{4}}}$ such that $f\left( 2 \right)=0$ then $f\left( x \right)$ is
${{x}^{4}}+\dfrac{1}{{{x}^{3}}}-\dfrac{129}{8}$
${{x}^{3}}+\dfrac{1}{{{x}^{4}}}+\dfrac{129}{8}$
${{x}^{4}}+\dfrac{1}{{{x}^{3}}}+\dfrac{129}{8}$
${{x}^{3}}+\dfrac{1}{{{x}^{4}}}-\dfrac{129}{8}$
Ans: Given, $\dfrac{d}{dx}f\left( x \right)=4{{x}^{3}}-\dfrac{3}{{{x}^{4}}}$
Anti-derivative of $4{{x}^{3}}-\dfrac{3}{{{x}^{4}}}=f\left( x \right)$
\[ \therefore f\left( x \right)=\int{4{{x}^{3}}-\dfrac{3}{{{x}^{4}}}=f\left( x \right)} \]
$ f\left( x \right)=4\int{{{x}^{3}}dx-3\int{\left( {{x}^{-4}} \right)}dx} $
$f\left( x \right)=4\left( \dfrac{{{x}^{4}}}{4} \right)-3\left( \dfrac{{{x}^{-3}}}{-3} \right)+C $
$ f\left( x \right)={{x}^{4}}+\dfrac{1}{{{x}^{3}}}+C$
Also,
$f\left( 2 \right)=0 $
$\therefore f\left( 2 \right)={{\left( 2 \right)}^{4}}+\dfrac{1}{{{\left( 2 \right)}^{3}}}+C=0 $
$ \Rightarrow 16+\dfrac{1}{8}+C=0 $
$ \Rightarrow C=\dfrac{-129}{8} $
$\therefore f\left( x \right)={{x}^{4}}+\dfrac{1}{{{x}^{3}}}-\dfrac{129}{8} $
Thus, the correct answer is A.
Exercise 7.2
1. $\dfrac{2x}{1+{{x}^{2}}}$
Ans: Substitute $1+{{x}^{2}}=t$
$\therefore 2xdx=dt $
$\Rightarrow \int{\dfrac{2x}{1+{{x}^{2}}}dx}=\int{\dfrac{1}{t}}dt$
$ =\log \left| t \right|+C$
$ =\log \left| 1+{{x}^{2}} \right|+C$
$ =\log \left( 1+{{x}^{2}} \right)+C $
2. $\dfrac{{{\left( \log x \right)}^{2}}}{x}$
Ans: Substitute $\log \left| x \right|=t$
$ \therefore \dfrac{1}{x}dx=dt $
$\Rightarrow \int{\dfrac{{{\left( \log \left| x \right| \right)}^{2}}}{x}}dx=\int{{{t}^{2}}dt} $
$=\dfrac{{{t}^{3}}}{3}+C$
$ =\dfrac{{{\left( \log \left| x \right| \right)}^{3}}}{3}+C $
3. ${\dfrac{1}{x+x\log x}}$
Ans: $\dfrac{1}{x+x\log x}=\dfrac{1}{x\left( 1+\log x \right)}$
Substitute $1+\log x=t$
$ \therefore \dfrac{1}{x}dx=dt$
$\Rightarrow \int{\dfrac{1}{x\left( 1+\log x \right)}dx}=\int{\dfrac{1}{t}dt} $
$ =\log \left| t \right|+C$
$=\log \left| 1+\log x \right|+C $
4. ${Sinx.\sin \left( \cos x \right)}$
Ans: $\operatorname{Sin}x.\sin \left( \cos x \right)$
$ Put,\cos x=t $
$ \therefore -\sin xdx=dt $
$ \Rightarrow \int{\sin x.\sin \left( \cos x \right)dx}=-\int{\sin tdt} $
$ =-\left[ -\cos t \right]+C $
$ =\cos t+C $
$ =\cos \left( \cos x \right)+C $
5. $\operatorname{sin}\left( ax+b \right)\cos \left( ax+b \right)$
Ans: $\operatorname{sin}\left( ax+b \right)\cos \left( ax+b \right)=\dfrac{2\sin \left( ax+b \right)\cos \left( ax+b \right)}{2}=\dfrac{\sin 2\left( ax+b \right)}{2}$
Substitute $2\left( ax+b \right)=t$
$ \therefore 2adx=dt $
$ \Rightarrow \int{\dfrac{\sin 2\left( ax+b \right)}{2}}dx=\dfrac{1}{2}\int{\dfrac{\sin t}{2a}}dt $
$ =\dfrac{1}{4a}\left[ -\cos t \right]+C $
$ =\dfrac{-1}{4a}\cos 2\left( ax+b \right)+C$
6. $\sqrt{ax+b}$
Ans: Substitute $ax+b=t$
$ \Rightarrow adx=dt $
$ \therefore dx=\dfrac{1}{a}dt $
$ \Rightarrow \int{{{\left( ax+b \right)}^{\dfrac{1}{2}}}dx}=\dfrac{1}{a}\int{{{t}^{\dfrac{1}{2}}}}dt $
$=\dfrac{1}{a}\left( \dfrac{{{t}^{\dfrac{1}{2}}}}{\dfrac{3}{2}} \right)+C=\dfrac{2}{3a}{{\left( ax+b \right)}^{\dfrac{3}{2}}}+C $
7. $x\sqrt{x+2}$
Ans: Substitute $x+2=t$
$\therefore dx=dt $
$ \Rightarrow \int{x\sqrt{x+2}}=\int{\left( t-2 \right)\sqrt{t}}dt$
$ =\int{\left( {{t}^{\dfrac{3}{2}}}-2{{t}^{\dfrac{1}{2}}} \right)}dt $
$ =\int{{{t}^{\dfrac{3}{2}}}dt-2\int{{{t}^{\dfrac{1}{2}}}}dt} $
$ =\dfrac{2}{5}{{t}^{\dfrac{5}{2}}}-\dfrac{4}{3}{{t}^{\dfrac{3}{2}}}+C $
8. $x\sqrt{1+2{{x}^{2}}}$
Ans: Substitute $1+2{{x}^{2}}=t$
$ \therefore 4xdx=dt $
$\Rightarrow \int{x\sqrt{1+2{{x}^{2}}}dx}=\int{\dfrac{\sqrt{t}dt}{4}} $
$ =\dfrac{1}{4}\int{{{t}^{\dfrac{1}{2}}}}dt $
$ =\dfrac{1}{4}\left( \dfrac{{{t}^{\dfrac{3}{2}}}}{\dfrac{3}{2}} \right)+C $
$=\dfrac{1}{6}{{\left( 1+2{{x}^{2}} \right)}^{\dfrac{3}{2}}}+C$
9. $\left( 4x+2 \right)\sqrt{{{x}^{2}}+x+1}$
Ans: Substitute ${{x}^{2}}+x+1=t$
$ \therefore \left( 2x+1 \right)dx=dt $
$ \int{\left( 4x+2 \right)\sqrt{{{x}^{2}}+x+1}}dx$
$ =\int{2\sqrt{t}dt}$
$=2\int{\sqrt{t}}dt $
$ =2\left( \dfrac{{{t}^{\dfrac{3}{2}}}}{\dfrac{3}{2}} \right)+C=\dfrac{4}{3}{{\left( {{x}^{2}}+x+1 \right)}^{\dfrac{3}{2}}}+C $
10. $\dfrac{1}{x-\sqrt{x}}$
Ans: $\dfrac{1}{x-\sqrt{x}}=\dfrac{1}{\sqrt{x}\left( \sqrt{x}-1 \right)}$
Substitute $\left( \sqrt{x-1} \right)=t$
$\Rightarrow \int{\dfrac{1}{\sqrt{x}\left( \sqrt{x}-1 \right)}dx=\int{\dfrac{2}{t}dt}} $
$ =2\log \left| t \right|+C$
$=2\log \left| \sqrt{x}-1 \right|+C$
11. $\dfrac{x}{\sqrt{x+4}},x>0$
Ans: Substitute $x+4=t$
$ \therefore dx=dt $
$ \int{\dfrac{x}{\sqrt{x+4}}dx}=\int{\dfrac{\left( t-4 \right)}{\sqrt{t}}dt=\int{\left( \sqrt{t}-\dfrac{4}{\sqrt{t}} \right)}}dt $
$ =\dfrac{{{t}^{\dfrac{3}{2}}}}{\dfrac{3}{2}}-4\left( \dfrac{{{t}^{\dfrac{1}{2}}}}{\dfrac{1}{2}} \right)+C=\dfrac{2}{3}{{\left( t \right)}^{\dfrac{3}{2}}}-8{{\left( t \right)}^{\dfrac{1}{2}}}+C $
$ =\dfrac{2}{3}t.{{t}^{\dfrac{1}{2}}}-8{{t}^{\dfrac{1}{2}}}+C $
$ =\dfrac{2}{3}{{t}^{\dfrac{1}{2}}}\left( t-12 \right)+C $
$=\dfrac{2}{3}\sqrt{x+4}\left( x-8 \right)+C $
12. ${{\left( {{x}^{3}}-1 \right)}^{\dfrac{1}{3}}}{{x}^{5}}$
Ans: Substitute ${{x}^{3}}-1=t$
$ \therefore 3{{x}^{2}}dx=dt $
$ \Rightarrow \int{{{\left( {{x}^{3}}-1 \right)}^{\dfrac{1}{3}}}{{x}^{5}}dx=\int{{{\left( {{x}^{3}}-1 \right)}^{\dfrac{1}{3}}}{{x}^{3}}.{{x}^{2}}dx}} $
$=\int{{{t}^{\dfrac{1}{3}}}\left( t+1 \right)\dfrac{dt}{3}=\dfrac{1}{3}\int{\left( {{t}^{\dfrac{4}{3}}}+{{t}^{\dfrac{1}{3}}} \right)dt}} $
$ =\dfrac{1}{3}\left[ \dfrac{3}{7}{{t}^{\dfrac{7}{3}}}+\dfrac{3}{4}{{t}^{\dfrac{4}{3}}} \right]+C $
$=\dfrac{1}{7}{{\left( {{x}^{3}}-1 \right)}^{\dfrac{7}{3}}}+\dfrac{1}{4}{{\left( {{x}^{3}}-1 \right)}^{\dfrac{4}{3}}}+C $
13. $\dfrac{{{x}^{2}}}{{{\left( 2+3{{x}^{3}} \right)}^{3}}}$
Ans: Substitute $2+3{{x}^{3}}=t$
$ \therefore 9{{x}^{2}}dx=dt $
$ \Rightarrow \int{\dfrac{{{x}^{2}}}{{{\left( 2+3{{x}^{3}} \right)}^{3}}}dx}=\dfrac{1}{9}\int{\dfrac{dt}{{{\left( t \right)}^{3}}}} $
$ =\dfrac{1}{9}\left[ \dfrac{{{t}^{-2}}}{-2} \right]+C $
$=\dfrac{-1}{18{{\left( 2+3{{x}^{3}} \right)}^{2}}}+C $
14. $\dfrac{1}{x{{\left( \log x \right)}^{m}}},x>0$
Ans: Substitute $\log x=t$
$ \dfrac{1}{x}dx=dt$
$\Rightarrow \int{\dfrac{1}{x{{\left( \log x \right)}^{m}}}dx}=\int{\dfrac{dt}{{{\left( t \right)}^{m}}}=\left( \dfrac{{{t}^{-m-1}}}{1-m} \right)}+C $
$ =\dfrac{{{\left( \log x \right)}^{1-m}}}{\left( 1-m \right)}+C $
15. $\dfrac{x}{9-4{{x}^{2}}}$
Ans: Substitute $9-4{{x}^{2}}=t$
$ \therefore -8xdx=dt $
$ \Rightarrow \int{\dfrac{x}{9-4{{x}^{2}}}dx=\dfrac{-1}{8}\int{\dfrac{1}{t}dt}} $
$=\dfrac{-1}{8}\log \left| t \right|+C $
$=\dfrac{-1}{8}\log \left| 9-4{{x}^{2}} \right|+C$
16. ${{e}^{2x+3}}$
Ans: Substitute $2x+3=t$
$\therefore 2dx=dt $
$ \Rightarrow \int{{{e}^{2x+3}}dx}=\dfrac{1}{2}\int{{{e}^{t}}dt} $
$ =\dfrac{1}{2}\left( {{e}^{t}} \right)+C $
$ =\dfrac{1}{2}{{e}^{\left( 2x+3 \right)}}+C $
17. $\dfrac{x}{{{e}^{{{x}^{2}}}}}$
Ans: Substitute ${{x}^{2}}=t$
$ \therefore 2xdx=dt$
$ \Rightarrow \int{\dfrac{x}{{{e}^{{{x}^{2}}}}}dx=\dfrac{1}{2}\int{\dfrac{1}{{{e}^{t}}}dt}} $
$=\dfrac{1}{2}\int{{{e}^{-t}}dt} $
$ =\dfrac{1}{2}\left( \dfrac{{{e}^{-t}}}{-1} \right)+C$
$=-\dfrac{1}{2}{{e}^{-{{x}^{2}}}}+C $
$ =\dfrac{-1}{2{{e}^{{{x}^{2}}}}}+C $
18. $\dfrac{{{e}^{{{\tan }^{-1}}x}}}{1+{{x}^{2}}}$
Ans: Substitute ${{\tan }^{-1}}x=t$
$ \therefore \dfrac{1}{1+{{x}^{2}}}dx=dt $
$ \Rightarrow \int{\dfrac{{{e}^{{{\tan }^{-1}}x}}}{1+{{x}^{2}}}dx=dt} $
$ ={{e}^{t}}+C $
$ ={{e}^{{{\tan }^{-1}}x}}+C$
19. Integrate \[\dfrac{{{e}^{2x}}-1}{{{e}^{2x}}+1}\]
Ans:
Dividing the given function’s numerator and denominator by $ {{e}^{x}} $ , we obtain,
\[\dfrac{\dfrac{\left( {{e}^{2x}}-1 \right)}{{{e}^{x}}}}{\dfrac{\left( {{e}^{2x}}+1 \right)}{{{e}^{x}}}}=\dfrac{{{e}^{x}}-{{e}^{-x}}}{{{e}^{x}}+{{e}^{-x}}}\]
Let \[{{e}^{x}}+{{e}^{-x}}=t\]
\[\left( {{e}^{x}}-{{e}^{-x}} \right)dx=dt\]
\[\Rightarrow \int{\dfrac{{{e}^{2x}}-1}{{{e}^{2x}}+1}}dx=\int{\dfrac{{{e}^{x}}-{{e}^{-x}}}{{{e}^{x}}+{{e}^{-x}}}}dx\]
\[=\int{\dfrac{dt}{t}}\]
\[=\log |t|+C\]
\[=\log |{{e}^{x}}-{{e}^{-x}}|+C\]
where C is an arbitrary constant.
20. Solve the following: $\dfrac{{{e}^{2x}}-{{e}^{-2x}}}{{{e}^{2x}}+{{e}^{-2x}}}$.
Ans: Given expression $\dfrac{{{e}^{2x}}-{{e}^{-2x}}}{{{e}^{2x}}+{{e}^{-2x}}}$.
Let us substitute ${{e}^{2x}}+{{e}^{-2x}}=t$, we get
$\left( 2{{e}^{2x}}+2{{e}^{-2x}} \right)dx=dt$
$\Rightarrow 2\left( {{e}^{2x}}-{{e}^{-2x}} \right)dx=dt$
Integration of given expression is
$\Rightarrow \int{\dfrac{{{e}^{2x}}-{{e}^{-2x}}}{{{e}^{2x}}+{{e}^{-2x}}}}=\int{\dfrac{dt}{2t}}$
$\Rightarrow \int{\dfrac{{{e}^{2x}}-{{e}^{-2x}}}{{{e}^{2x}}+{{e}^{-2x}}}}=\dfrac{1}{2}\int{\dfrac{1}{t}dt}$
$\Rightarrow \int{\dfrac{{{e}^{2x}}-{{e}^{-2x}}}{{{e}^{2x}}+{{e}^{-2x}}}}=\dfrac{1}{2}\log \left| t \right|+C$
Again substitute $t={{e}^{2x}}+{{e}^{-2x}}$, we get
$\therefore\int{\dfrac{{{e}^{2x}}-{{e}^{-2x}}}{{{e}^{2x}}+{{e}^{-2x}}}}=\dfrac{1}{2}\log \left| {{e}^{2x}}+{{e}^{-2x}} \right|+C$
21. Solve the following: ${{\tan }^{2}}\left( 2x-3 \right)$.
Ans: Given expression ${{\tan }^{2}}\left( 2x-3 \right)$.
We can apply the identity ${{\tan }^{2}}x={{\sec }^{2}}x-1$, we get
${{\tan }^{2}}\left( 2x-3 \right)={{\sec }^{2}}\left( 2x-3 \right)-1$
Substitute $2x-3=t$, we get
$2dx=dt$
Integration of given expression is
$\Rightarrow \int{{{\tan }^{2}}\left( 2x-3 \right)dx}=\int{{{\sec }^{2}}\left( 2x-3 \right)-1dx}$
$\Rightarrow \int{{{\tan }^{2}}\left( 2x-3 \right)dx}=\dfrac{1}{2}\int{{{\sec }^{2}}tdt-\int{1dx}}$
$\Rightarrow \int{{{\tan }^{2}}\left( 2x-3 \right)dx}=\dfrac{1}{2}\tan t-x+C$
Substitute $2x-3=t$
$\therefore \int{{{\tan }^{2}}\left( 2x-3 \right)dx}=\dfrac{1}{2}\tan \left( 2x-3 \right)-x+C$
22. Solve the following: ${{\sec }^{2}}\left( 7-4x \right)$.
Ans: Given expression ${{\sec }^{2}}\left( 7-4x \right)$.
Put $7-4x=t$, we get
$\therefore -4dx=dt$
Integration of given expression is
$\Rightarrow \int{{{\sec }^{2}}\left( 7-4x \right)dx=-\dfrac{1}{4}\int{{{\sec }^{2}}tdt}}$
$\Rightarrow \int{{{\sec }^{2}}\left( 7-4x \right)dx=-\dfrac{1}{4}\tan t+C}$
Substitute $7-4x=t$, we get
$\therefore \int{{{\sec }^{2}}\left( 7-4x \right)dx=-\dfrac{1}{4}\tan \left( 7-4x \right)+C}$
23. Solve the following: $\dfrac{{{\sin }^{-1}}x}{\sqrt{1-{{x}^{2}}}}$.
Ans: Given expression $\dfrac{{{\sin }^{-1}}x}{\sqrt{1-{{x}^{2}}}}$.
Put ${{\sin }^{-1}}x=t$, we get
$\Rightarrow \dfrac{1}{\sqrt{1-{{x}^{2}}}}dx=dt$
Integration of given expression is
$\Rightarrow \int{\dfrac{{{\sin }^{-1}}x}{\sqrt{1-{{x}^{2}}}}dx=\int{tdt}}$
$\Rightarrow \int{\dfrac{{{\sin }^{-1}}x}{\sqrt{1-{{x}^{2}}}}dx=\dfrac{{{t}^{2}}}{2}+C}$
Substitute ${{\sin }^{-1}}x=t$, we get
$\therefore \int{\dfrac{{{\sin }^{-1}}x}{\sqrt{1-{{x}^{2}}}}dx=\dfrac{{{\left( {{\sin }^{-1}}x \right)}^{2}}}{2}+C}$
24. Solve the following: $\dfrac{2\cos x-3\sin x}{6\cos x+4\sin x}$.
Ans: Given expression is $\dfrac{2\cos x-3\sin x}{6\cos x+4\sin x}$.
Given expression can be written as
$\dfrac{2\cos x-3\sin x}{6\cos x+4\sin x}=\dfrac{2\cos x-3\sin x}{2\left( 3\cos x+2\sin x \right)}$
Let $3\cos x+2\sin x=t$, we get
$\left( -3\sin x+2\cos x \right)dx=dt$
Integration of given expression is
$\Rightarrow \int{\dfrac{2\cos x-3\sin x}{6\cos x+4\sin x}}dx=\int{\dfrac{2\cos x-3\sin x}{2\left( 3\cos x+2\sin x \right)}}dx$
$\Rightarrow \int{\dfrac{2\cos x-3\sin x}{6\cos x+4\sin x}}dx=\int{\dfrac{dt}{2t}}$
$\Rightarrow \int{\dfrac{2\cos x-3\sin x}{6\cos x+4\sin x}}dx=\dfrac{1}{2}\int{\dfrac{1}{t}dt}$
$\Rightarrow \int{\dfrac{2\cos x-3\sin x}{6\cos x+4\sin x}}dx=\dfrac{1}{2}\log \left| t \right|+C$
Substitute $3\cos x+2\sin x=t$
$\therefore \int{\dfrac{2\cos x-3\sin x}{6\cos x+4\sin x}}dx=\dfrac{1}{2}\log \left| 2\sin x+3\cos x \right|+C$
25. Solve the following: $\dfrac{1}{{{\cos }^{2}}x{{\left( 1-\tan x \right)}^{2}}}$.
Ans: Given expression $\dfrac{1}{{{\cos }^{2}}x{{\left( 1-\tan x \right)}^{2}}}$.
Given expression can be written as
$\dfrac{1}{{{\cos }^{2}}x{{\left( 1-\tan x \right)}^{2}}}=\dfrac{{{\sec }^{2}}x}{{{\left( 1-\tan x \right)}^{2}}}$
Let $\left( 1-\tan x \right)=t$, we get
$-{{\sec }^{2}}xdx=dt$
Integration of given expression is
$\Rightarrow \int{\dfrac{1}{{{\cos }^{2}}x{{\left( 1-\tan x \right)}^{2}}}}dx=\int{\dfrac{{{\sec }^{2}}x}{{{\left( 1-\tan x \right)}^{2}}}}dx$
$\Rightarrow \int{\dfrac{1}{{{\cos }^{2}}x{{\left( 1-\tan x \right)}^{2}}}}dx=\int{\dfrac{-dt}{{{t}^{2}}}}$
$\Rightarrow \int{\dfrac{1}{{{\cos }^{2}}x{{\left( 1-\tan x \right)}^{2}}}}dx=-\int{{{t}^{-2}}dt}$
$\Rightarrow \int{\dfrac{1}{{{\cos }^{2}}x{{\left( 1-\tan x \right)}^{2}}}}dx=\dfrac{1}{t}+C$
Substitute $\left( 1-\tan x \right)=t$,
$\therefore \int{\dfrac{1}{{{\cos }^{2}}x{{\left( 1-\tan x \right)}^{2}}}}dx=\dfrac{1}{\left( 1-\tan x \right)}+C$
26. Solve the following: $\dfrac{\cos \sqrt{x}}{\sqrt{x}}$.
Ans: Given expression is $\dfrac{\cos \sqrt{x}}{\sqrt{x}}$.
Let $\sqrt{x}=t$, we get
$\dfrac{1}{2\sqrt{x}}dx=dt$
Integration of given expression is
$\Rightarrow \int{\dfrac{\cos \sqrt{x}}{\sqrt{x}}dx}=2\int{\cos tdt}$
$\Rightarrow \int{\dfrac{\cos \sqrt{x}}{\sqrt{x}}dx}=2\sin t+C$
Substitute $\sqrt{x}=t$
$\therefore \int{\dfrac{\cos \sqrt{x}}{\sqrt{x}}dx}=2\sin \sqrt{x}+C$
27. Solve the following: $\sqrt{\sin 2x}\cos 2x$.
Ans: Given expression is $\sqrt{\sin 2x}\cos 2x$.
Let $\sin 2x=t$, we get
$2\cos 2xdx=dt$
Integration of given expression is
$\Rightarrow \int{\sqrt{\sin 2x}\cos 2xdx=\dfrac{1}{2}\int{\sqrt{t}dt}}$
$\Rightarrow \int{\sqrt{\sin 2x}\cos 2xdx=\dfrac{1}{2}\left( \dfrac{{{t}^{\dfrac{3}{2}}}}{\dfrac{3}{2}} \right)+C}$
$\Rightarrow \int{\sqrt{\sin 2x}\cos 2xdx=\dfrac{1}{3}{{t}^{\dfrac{3}{2}}}+C}$
Substitute $\sin 2x=t$
$\therefore \int{\sqrt{\sin 2x}\cos 2xdx=\dfrac{1}{3}{{\left( \sin 2x \right)}^{\dfrac{3}{2}}}+C}$
28. Solve the following: $\dfrac{\cos x}{\sqrt{1+\sin x}}$.
Ans: Given expression $\dfrac{\cos x}{\sqrt{1+\sin x}}$.
Let $1+\sin x=t$
$\therefore \cos xdx=dt$
Integration of given expression is
$\Rightarrow \int{\dfrac{\cos x}{\sqrt{1+\sin x}}dx}=\int{\dfrac{dt}{\sqrt{t}}}$
$\Rightarrow \int{\dfrac{\cos x}{\sqrt{1+\sin x}}dx}=\dfrac{{{t}^{\dfrac{1}{2}}}}{\dfrac{1}{2}}+C$
$\Rightarrow \int{\dfrac{\cos x}{\sqrt{1+\sin x}}dx}=2\sqrt{t}+C$
Substitute $1+\sin x=t$,
$\therefore \int{\dfrac{\cos x}{\sqrt{1+\sin x}}dx}=2\sqrt{1+\sin x}+C$
29. Solve the following: $\cot x\log \sin x$.
Ans: Given expression $\cot x\log \sin x$.
Let $\log \sin x=t$, we get
$\dfrac{1}{\sin x}\cos xdx=dt$
$\Rightarrow \cot xdx=dt$
Integration of given expression is
$\int{\cot x\log \sin xdx=\int{tdt}}$
$\Rightarrow \int{\cot x\log \sin xdx=\dfrac{{{t}^{2}}}{2}+C}$
Substitute $\log \sin x=t$,
$\therefore \int{\cot x\log \sin xdx=\dfrac{1}{2}{{\left( \log \sin x \right)}^{2}}+C}$
30. Solve the following: $\dfrac{\sin x}{1+\cos x}$.
Ans: Given expression $\dfrac{\sin x}{1+\cos x}$.
Let $1+\cos x=t$
$\therefore -\sin xdx=dt$
Integration of given expression is
$\Rightarrow \int{\dfrac{\sin x}{1+\cos x}dx=\int{-\dfrac{dt}{t}}}$
$\Rightarrow \int{\dfrac{\sin x}{1+\cos x}dx=-\log \left| t \right|+C}$
Substitute $1+\cos x=t$,
$\therefore \int{\dfrac{\sin x}{1+\cos x}dx=-\log \left| 1+\cos x \right|+C}$
31. Solve the following: $\dfrac{\sin x}{{{\left( 1+\cos x \right)}^{2}}}$.
Ans: Given expression $\dfrac{\sin x}{{{\left( 1+\cos x \right)}^{2}}}$.
Let $1+\cos x=t$
$\therefore -\sin xdx=dt$
Integration of given expression is
$\Rightarrow \int{\dfrac{\sin x}{{{\left( 1+\cos x \right)}^{2}}}dx=\int{-\dfrac{dt}{{{t}^{2}}}}}$
$\Rightarrow \int{\dfrac{\sin x}{{{\left( 1+\cos x \right)}^{2}}}dx=-\int{{{t}^{-2}}dt}}$
$\Rightarrow \int{\dfrac{\sin x}{{{\left( 1+\cos x \right)}^{2}}}dx=\dfrac{1}{t}+C}$
Substitute $1+\cos x=t$,
$\therefore \int{\dfrac{\sin x}{{{\left( 1+\cos x \right)}^{2}}}dx=\dfrac{1}{1+\cos x}+C}$
.
32.Solve the following: $\dfrac{1}{1+\cot x}$.
Ans: Given expression $\dfrac{1}{1+\cot x}$.
Let $I=\int{\dfrac{1}{1+\cot x}}dx$
Integration of given expression is
$\Rightarrow I=\int{\dfrac{1}{1+\cot x}}dx$
$\Rightarrow I=\int{\dfrac{1}{1+\dfrac{\cos x}{\sin x}}}dx$
$\Rightarrow I=\int{\dfrac{\sin x}{\sin x+\cos x}}dx$
$\Rightarrow I=\dfrac{1}{2}\int{\dfrac{2\sin x}{\sin x+\cos x}}dx$
$\Rightarrow I=\dfrac{1}{2}\int{\dfrac{\left( \sin x+\cos x \right)+\left( \sin x-\cos x \right)}{\sin x+\cos x}}dx$
$\Rightarrow I=\dfrac{1}{2}\int{\dfrac{\left( \sin x+\cos x \right)}{\sin x+\cos x}+\dfrac{\left( \sin x-\cos x \right)}{\sin x+\cos x}}dx$
$\Rightarrow I=\dfrac{1}{2}\int{1dx+\dfrac{1}{2}\int{\dfrac{\left( \sin x-\cos x \right)}{\sin x+\cos x}}}dx$
Let $\sin x+\cos x=t$
$\therefore \left( \cos x-\sin x \right)dx=dt$
Substitute in above obtained equation, we get
$\Rightarrow I=\dfrac{1}{2}x+\dfrac{1}{2}\int{-\dfrac{dt}{t}}$
$\Rightarrow I=\dfrac{x}{2}-\dfrac{1}{2}\log \left| t \right|+C$
Substitute $\sin x+\cos x=t$,
$\therefore I=\dfrac{x}{2}-\dfrac{1}{2}\log \left| \sin x+\cos x \right|+C$
33. Solve the following: $\dfrac{1}{1-\tan x}$.
Ans: Given expression $\dfrac{1}{1-\tan x}$.
Let $I=\int{\dfrac{1}{1-\tan x}}dx$
Integration of given expression is
$\Rightarrow I=\int{\dfrac{1}{1-\tan x}}dx$
$\Rightarrow I=\int{\dfrac{1}{1-\dfrac{\sin x}{\cos x}}}dx$
$\Rightarrow I=\int{\dfrac{\cos x}{\cos x-\sin x}}dx$
$\Rightarrow I=\dfrac{1}{2}\int{\dfrac{2\cos x}{\cos x-\sin x}}dx$
$\Rightarrow I=\dfrac{1}{2}\int{\dfrac{\left( \cos x-\sin x \right)+\left( \cos x+\sin x \right)}{\cos x-\sin x}}dx$
$\Rightarrow I=\dfrac{1}{2}\int{\dfrac{\left( \cos x-\sin x \right)}{\cos x-\sin x}+\dfrac{\left( \cos x+\sin x \right)}{\cos x-\sin x}}dx$
$\Rightarrow I=\dfrac{1}{2}\int{1dx+\dfrac{1}{2}\int{\dfrac{\left( \cos x+\sin x \right)}{\cos x-\sin x}}}dx$
Let $\cos x-\sin x=t$
$\therefore \left( -\sin x-\cos x \right)dx=dt$
Substitute in above obtained equation, we get
$\Rightarrow I=\dfrac{1}{2}x+\dfrac{1}{2}\int{-\dfrac{dt}{t}}$
$\Rightarrow I=\dfrac{x}{2}-\dfrac{1}{2}\log \left| t \right|+C$
Substitute $\cos x-\sin x=t$,
$\therefore I=\dfrac{x}{2}-\dfrac{1}{2}\log \left| \cos x-\sin x \right|+C$
34. Solve the following: $\dfrac{\sqrt{\tan x}}{\sin x\cos x}$.
Ans: Given expression $\dfrac{\sqrt{\tan x}}{\sin x\cos x}$.
Let $I=\int{\dfrac{\sqrt{\tan x}}{\sin x\cos x}dx}$
Multiply and divide by $\cos x$, we get
$\Rightarrow I=\int{\dfrac{\sqrt{\tan x}\times \cos x}{\sin x\cos x\times \cos x}dx}$
$\Rightarrow I=\int{\dfrac{\sqrt{\tan x}\times \cos x}{\tan x\times {{\cos }^{2}}x}dx}$
$\Rightarrow I=\int{\dfrac{{{\sec }^{2}}x}{\sqrt{\tan x}}dx}$
Let $\tan x=t$
$\therefore {{\sec }^{2}}xdx=dt$
Substitute in above obtained equation, we get
$\Rightarrow I=\int{\dfrac{dt}{\sqrt{t}}dx}$
$\Rightarrow I=2\sqrt{t}+C$
Substitute $\tan x=t$,
$\therefore I=2\sqrt{\tan x}+C$
35. Solve the following: $\dfrac{{{\left( 1+\log x \right)}^{2}}}{x}$.
Ans: Given expression $\dfrac{{{\left( 1+\log x \right)}^{2}}}{x}$.
Let $1+\log x=t$
$\therefore \dfrac{1}{x}dx=dt$
Integration of given expression is
$\int{\dfrac{{{\left( 1+\log x \right)}^{2}}}{x}dx=\int{{{t}^{2}}dt}}$
$\Rightarrow \int{\dfrac{{{\left( 1+\log x \right)}^{2}}}{x}dx=\dfrac{{{t}^{3}}}{3}+C}$
Substitute $1+\log x=t$
$\therefore \int{\dfrac{{{\left( 1+\log x \right)}^{2}}}{x}dx=\dfrac{{{\left( 1+\log x \right)}^{3}}}{3}+C}$
36. Solve the following: $\dfrac{\left( x+1 \right){{\left( x+\log x \right)}^{2}}}{x}$.
Ans: Given expression $\dfrac{\left( x+1 \right){{\left( x+\log x \right)}^{2}}}{x}$.
Given expression can be written as
$\dfrac{\left( x+1 \right){{\left( x+\log x \right)}^{2}}}{x}=\left( \dfrac{x+1}{x} \right){{\left( x+\log x \right)}^{2}}$
$\Rightarrow \dfrac{\left( x+1 \right){{\left( x+\log x \right)}^{2}}}{x}=\left( 1+\dfrac{1}{x} \right){{\left( x+\log x \right)}^{2}}$
Let $x+\log x=t$
$\therefore \left( 1+\dfrac{1}{x} \right)dx=dt$
Integration of given expression is
$\Rightarrow \int{\dfrac{\left( x+1 \right){{\left( x+\log x \right)}^{2}}}{x}dx=\int{{{t}^{2}}dt}}$
$\Rightarrow \int{\dfrac{\left( x+1 \right){{\left( x+\log x \right)}^{2}}}{x}dx=\dfrac{{{t}^{3}}}{3}+C}$
Substitute $x+\log x=t$
$\therefore \int{\dfrac{\left( x+1 \right){{\left( x+\log x \right)}^{2}}}{x}dx=\dfrac{1}{3}{{\left( x+\log x \right)}^{3}}+C}$
37. Solve the following: $\dfrac{{{x}^{3}}\sin \left( {{\tan }^{-1}}{{x}^{4}} \right)}{1+{{x}^{8}}}$.
Ans: Given expression $\dfrac{{{x}^{3}}\sin \left( {{\tan }^{-1}}{{x}^{4}} \right)}{1+{{x}^{8}}}$.
Let ${{x}^{4}}=t$,
$\therefore 4{{x}^{3}}dx=dt$
Integration of given expression is
$\Rightarrow \int{\dfrac{{{x}^{3}}\sin \left( {{\tan }^{-1}}{{x}^{4}} \right)}{1+{{x}^{8}}}dx=\dfrac{1}{4}\int{\dfrac{\sin \left( {{\tan }^{-1}}t \right)}{1+{{t}^{2}}}dt}}$ ………(1)
Let ${{\tan }^{-1}}t=u$
$\therefore \dfrac{1}{1+{{t}^{2}}}dt=du$
Substitute in eq. (1), we get
$\Rightarrow \int{\dfrac{{{x}^{3}}\sin \left( {{\tan }^{-1}}{{x}^{4}} \right)}{1+{{x}^{8}}}dx=\dfrac{1}{4}\int{\sin udu}}$
$\Rightarrow \int{\dfrac{{{x}^{3}}\sin \left( {{\tan }^{-1}}{{x}^{4}} \right)}{1+{{x}^{8}}}dx=\dfrac{1}{4}\left( -\cos u \right)+C}$
Substitute ${{\tan }^{-1}}t=u$,
$\Rightarrow \int{\dfrac{{{x}^{3}}\sin \left( {{\tan }^{-1}}{{x}^{4}} \right)}{1+{{x}^{8}}}dx=-\dfrac{1}{4}\cos \left( {{\tan }^{-1}}t \right)+C}$
Substitute ${{x}^{4}}=t$,
$\therefore \int{\dfrac{{{x}^{3}}\sin \left( {{\tan }^{-1}}{{x}^{4}} \right)}{1+{{x}^{8}}}dx=-\dfrac{1}{4}\cos \left( {{\tan }^{-1}}{{x}^{4}} \right)+C}$
38. Choose the correct answer in Question 38 and 39.
38. $\int{\dfrac{10{{x}^{9}}+{{10}^{x}}{{\log }_{e}}10}{{{x}^{10}}+{{10}^{x}}}}dx$ equals
${{10}^{x}}-{{x}^{10}}+C$
${{10}^{x}}+{{x}^{10}}+C$
${{\left( {{10}^{x}}-{{x}^{10}} \right)}^{-1}}+C$
$\log \left( {{10}^{x}}+{{x}^{10}} \right)+C$
Ans: Given expression $\int{\dfrac{10{{x}^{9}}+{{10}^{x}}{{\log }_{e}}10}{{{x}^{10}}+{{10}^{x}}}dx}$.
Let ${{x}^{10}}+{{10}^{x}}=t$,
$\therefore \left( 10{{x}^{9}}+{{10}^{x}}{{\log }_{e}}10 \right)dx=dt$
Integration of given expression is
$\Rightarrow \int{\dfrac{10{{x}^{9}}+{{10}^{x}}{{\log }_{e}}10}{{{x}^{10}}+{{10}^{x}}}dx}=\int{\dfrac{dt}{t}}$
$\Rightarrow \int{\dfrac{10{{x}^{9}}+{{10}^{x}}{{\log }_{e}}10}{{{x}^{10}}+{{10}^{x}}}dx}=\log t+C$
Substitute ${{x}^{10}}+{{10}^{x}}=t$,
$\therefore \int{\dfrac{10{{x}^{9}}+{{10}^{x}}{{\log }_{e}}10}{{{x}^{10}}+{{10}^{x}}}dx}=\log \left( {{10}^{x}}+{{x}^{10}} \right)+C$
Therefore, option D is the correct answer.
39. $\int{\dfrac{dx}{{{\sin }^{2}}{{\cos }^{2}}x}}$ equals
$\tan x+\cot x+C$
$\tan x-\cot x+C$
$\tan x\cot x+C$
$\tan x-\cot 2x+C$
Ans: Given expression $\int{\dfrac{dx}{{{\sin }^{2}}x{{\cos }^{2}}x}}$.
Let $I=\int{\dfrac{dx}{{{\sin }^{2}}x{{\cos }^{2}}x}}$
$I=\int{\dfrac{1}{{{\sin }^{2}}x{{\cos }^{2}}x}dx}$
We know that ${{\sin }^{2}}x+{{\cos }^{2}}x=1$, we get
$\Rightarrow I=\int{\dfrac{{{\sin }^{2}}x+{{\cos }^{2}}x}{{{\sin }^{2}}x{{\cos }^{2}}x}dx}$
$\Rightarrow I=\int{\dfrac{{{\sin }^{2}}x}{{{\sin }^{2}}x{{\cos }^{2}}x}dx}+\int{\dfrac{{{\cos }^{2}}x}{{{\sin }^{2}}x{{\cos }^{2}}x}dx}$
$\Rightarrow I=\int{\dfrac{1}{{{\cos }^{2}}x}dx}+\int{\dfrac{1}{{{\sin }^{2}}x}dx}$
$\Rightarrow I=\int{{{\sec }^{2}}xdx}+\int{cose{{c}^{2}}xdx}$
$\Rightarrow I=\tan x-\cot x+C$
Therefore, option B is the correct answer.
Exercise 7.3
1. Solve the following: ${{\sin }^{2}}\left( 2x+5 \right)$.
Ans: Given expression ${{\sin }^{2}}\left( 2x+5 \right)$.
Given expression can be written as
${{\sin }^{2}}\left( 2x+5 \right)=\dfrac{1-\cos 2\left( 2x+5 \right)}{2}$
$\Rightarrow {{\sin }^{2}}\left( 2x+5 \right)=\dfrac{1-\cos \left( 4x+10 \right)}{2}$
Integration of given expression is
$\Rightarrow \int{{{\sin }^{2}}\left( 2x+5 \right)dx}=\int{\dfrac{1-\cos \left( 4x+10 \right)}{2}dx}$
$\Rightarrow \int{{{\sin }^{2}}\left( 2x+5 \right)dx}=\dfrac{1}{2}\int{1dx-\dfrac{1}{2}\int{\cos \left( 4x+10 \right)dx}}$
$\Rightarrow \int{{{\sin }^{2}}\left( 2x+5 \right)dx}=\dfrac{1}{2}x-\dfrac{1}{2}\left( \dfrac{\sin \left( 4x+10 \right)}{4} \right)+C$
$\therefore \int{{{\sin }^{2}}\left( 2x+5 \right)dx}=\dfrac{1}{2}x-\dfrac{1}{8}\sin \left( 4x+10 \right)+C$
2. Solve the following: $\sin 3x\cos 4x$.
Ans: Given expression $\sin 3x\cos 4x$.
Using the identity $\sin A\cos B=\dfrac{1}{2}\left\{ \sin \left( A+B \right)+\sin \left( A-B \right) \right\}$ given expression can be written as
$\sin 3x\cos 4x=\dfrac{1}{2}\left\{ \sin \left( 3x+4x \right)+\sin \left( 3x-4x \right) \right\}$
Integration of above expression is
$\Rightarrow \int{\sin 3x\cos 4x}dx=\int{\dfrac{1}{2}\left\{ \sin 7x+\sin \left( -x \right) \right\}dx}$
$\Rightarrow \int{\sin 3x\cos 4x}dx=\dfrac{1}{2}\int{\left\{ \sin 7x+\sin x \right\}dx}$
$\Rightarrow \int{\sin 3x\cos 4x}dx=\dfrac{1}{2}\int{\sin 7xdx+\dfrac{1}{2}\int{\sin x}dx}$
$\Rightarrow \int{\sin 3x\cos 4x}dx=\dfrac{1}{2}\left( \dfrac{-\cos 7x}{7} \right)-\dfrac{1}{2}\left( -\cos x \right)+C$
$\therefore \int{\sin 3x\cos 4x}dx=\dfrac{-\cos 7x}{14}+\dfrac{\cos x}{2}+C$
3. Solve the following: $\cos 2x\cos 4x\cos 6x$.
Ans: Given expression $\cos 2x\cos 4x\cos 6x$.
Using the identity $\cos A\cos B=\dfrac{1}{2}\left\{ \cos \left( A+B \right)+\cos \left( A-B \right) \right\}$ given expression can be written as
$\cos 2x\left( \cos 4x\cos 6x \right)=\cos 2x\dfrac{1}{2}\left\{ \cos \left( 4x+6x \right)+\cos \left( 4x-6x \right) \right\}$
Integration of the above expression is
$\Rightarrow \int{\cos 2x\left( \cos 4x\cos 6x \right)}=\int{\cos 2x}\left[ \dfrac{1}{2}\left\{ \cos 10x+\cos \left( -2x \right) \right\} \right]dx$
$\Rightarrow \int{\cos 2x\left( \cos 4x\cos 6x \right)}=\int{\left[ \dfrac{1}{2}\left\{ \cos 2x\cos 10x+\cos 2x\cos \left( -2x \right) \right\} \right]}dx$
$\Rightarrow \int{\cos 2x\left( \cos 4x\cos 6x \right)}=\dfrac{1}{2}\int{\left[ \left\{ \cos 2x\cos 10x+{{\cos }^{2}}2x \right\} \right]}dx$
Again applying the identity $\cos A\cos B=\dfrac{1}{2}\left\{ \cos \left( A+B \right)+\cos \left( A-B \right) \right\}$, we get
$\Rightarrow \int{\cos 2x\left( \cos 4x\cos 6x \right)}=\dfrac{1}{2}\int{\left[ \left\{ \dfrac{1}{2}\cos \left( 2x+10x \right)+\cos \left( 2x-10x \right)+\left( \dfrac{1+\cos 4x}{2} \right) \right\} \right]}dx$$\Rightarrow \int{\cos 2x\left( \cos 4x\cos 6x \right)}=\dfrac{1}{4}\int{\left[ \cos 12x+\cos 8x+\cos 4x \right]}dx$
$\therefore \int{\cos 2x\cos 4x\cos 6x}=\dfrac{1}{4}\left[ \dfrac{\sin 12x}{12}+\dfrac{\sin 8x}{8}+\dfrac{\sin 4x}{4} \right]+C$
4. Solve the following: ${{\sin }^{3}}\left( 2x+1 \right)$.
Ans: Given expression ${{\sin }^{3}}\left( 2x+1 \right)$.
Let $I=\int{{{\sin }^{3}}\left( 2x+1 \right)dx}$
$\Rightarrow I=\int{{{\sin }^{2}}\left( 2x+1 \right)\sin \left( 2x+1 \right)dx}$
$\Rightarrow I=\int{\left( 1-{{\cos }^{2}}\left( 2x+1 \right) \right)\sin \left( 2x+1 \right)dx}$
Let $\cos \left( 2x+1 \right)=t$
$\therefore -2\sin \left( 2x+1 \right)dx=dt$
Integration becomes
$\Rightarrow I=-\dfrac{1}{2}\int{\left( 1-{{t}^{2}} \right)dt}$
$\Rightarrow I=-\dfrac{1}{2}\left( t-\dfrac{{{t}^{3}}}{3} \right)+C$
Substitute $\cos \left( 2x+1 \right)=t$,
$\Rightarrow I=-\dfrac{1}{2}\left( \cos \left( 2x+1 \right)-\dfrac{{{\cos }^{3}}\left( 2x+1 \right)}{3} \right)+C$
$\therefore \int{{{\sin }^{3}}\left( 2x+1 \right)dx}=\dfrac{-\cos \left( 2x+1 \right)}{2}+\dfrac{{{\cos }^{3}}\left( 2x+1 \right)}{3}+C$
5. Solve the following: ${{\sin }^{3}}x{{\cos }^{3}}x$.
Ans: Given expression ${{\sin }^{3}}x{{\cos }^{3}}x$.
Let $I=\int{{{\sin }^{3}}x{{\cos }^{3}}x}dx$
$\Rightarrow I=\int{{{\sin }^{2}}x\sin x{{\cos }^{3}}x}dx$
$\Rightarrow I=\int{{{\cos }^{3}}x\left( 1-{{\cos }^{2}}x \right)\sin x}dx$
Let $\cos x=t$
$\therefore -\sin dx=dt$
Integration becomes
$\Rightarrow I=-\int{{{t}^{3}}\left( 1-{{t}^{2}} \right)}dt$
$\Rightarrow I=-\int{\left( {{t}^{3}}-{{t}^{5}} \right)}dt$
$\Rightarrow I=-\left[ \dfrac{{{t}^{4}}}{4}-\dfrac{{{t}^{6}}}{6} \right]+C$
Substitute $\cos x=t$,
$\Rightarrow I=-\left[ \dfrac{{{\cos }^{4}}x}{4}-\dfrac{{{\cos }^{6}}x}{6} \right]+C$
$\therefore \int{{{\sin }^{3}}x{{\cos }^{3}}x}dx=\dfrac{{{\cos }^{6}}x}{6}-\dfrac{{{\cos }^{4}}x}{4}+C$
6. Solve the following: $\sin x\sin 2x\sin 3x$.
Ans: Given expression $\sin x\sin 2x\sin 3x$.
Using the identity $\sin A\sin B=\dfrac{1}{2}\cos \left( A-B \right)-\cos \left( A+B \right)$, given expression can be written as
$\Rightarrow \sin x\sin 2x\sin 3x=\sin x.\dfrac{1}{2}\cos \left( 2x-3x \right)-\cos \left( 2x+3x \right)$
Integration of given expression is
$\Rightarrow \int{\sin x\sin 2x\sin 3x}dx=\dfrac{1}{2}\int{\left( \sin x\cos \left( -x \right)-\sin x\cos \left( 5x \right) \right)dx}$
$\Rightarrow \int{\sin x\sin 2x\sin 3x}dx=\dfrac{1}{2}\int{\left( \sin x\cos x-\sin x\cos 5x \right)dx}$
$\Rightarrow \int{\sin x\sin 2x\sin 3x}dx=\dfrac{1}{2}\int{\dfrac{\sin 2x}{2}dx-\dfrac{1}{2}\int{\left( \sin x\cos 5x \right)}dx}$
$\Rightarrow \int{\sin x\sin 2x\sin 3x}dx=\dfrac{1}{4}\left( \dfrac{-\cos 2x}{2} \right)-\dfrac{1}{2}\int{\left\{ \dfrac{1}{2}\left( \sin \left( x+5x \right)+\sin \left( x-5x \right) \right) \right\}dx}$
$\Rightarrow \int{\sin x\sin 2x\sin 3x}dx=\dfrac{-\cos 2x}{8}-\dfrac{1}{4}\int{\left\{ \left( \sin 6x+\sin \left( -4x \right) \right) \right\}dx}$
$\Rightarrow \int{\sin x\sin 2x\sin 3x}dx=\dfrac{-\cos 2x}{8}-\dfrac{1}{4}\int{\left\{ \left( \sin 6x+\sin 4x \right) \right\}dx}$
$\Rightarrow \int{\sin x\sin 2x\sin 3x}dx=\dfrac{-\cos 2x}{8}-\dfrac{1}{8}\left[ \dfrac{-\cos 6x}{3}+\dfrac{\cos 4x}{4} \right]+C$
$\therefore \int{\sin x\sin 2x\sin 3x}dx=\dfrac{1}{8}\left[ \dfrac{\cos 6x}{3}-\dfrac{\cos 4x}{4}-\cos 2x \right]+C$
7. Solve the following: $\sin 4x\sin 8x$.
Ans: Given expression $\sin 4x\sin 8x$.
Using the identity $\sin A\sin B=\dfrac{1}{2}\cos \left( A-B \right)-\cos \left( A+B \right)$, given expression can be written as
$\Rightarrow \sin 4x\sin 8x=\dfrac{1}{2}\cos \left( 4x-8x \right)-\cos \left( 4x+8x \right)$
Integration of given expression is
$\Rightarrow \int{\sin 4x\sin 8x}dx=\dfrac{1}{2}\int{\left( \cos \left( -4x \right)-\cos \left( 12x \right) \right)dx}$
$\Rightarrow \int{\sin 4x\sin 8x}dx=\dfrac{1}{2}\int{\left( \cos 4x-\cos 12x \right)dx}$
$\therefore \int{\sin 4x\sin 8x}dx=\dfrac{1}{2}\left[ \dfrac{\sin 4x}{4}-\dfrac{\sin 12x}{12} \right]+C$
8. Solve the following: $\dfrac{1-\cos x}{1+\cos x}$.
Ans: Given expression $\dfrac{1-\cos x}{1+\cos x}$.
Using the identities $2{{\sin }^{2}}\dfrac{x}{2}=1-\cos x$ and $\cos x=2{{\cos }^{2}}\dfrac{x}{2}-1$ given expression can be written as
$\Rightarrow \dfrac{1-\cos x}{1+\cos x}=\dfrac{2{{\sin }^{2}}\dfrac{x}{2}}{2{{\cos }^{2}}\dfrac{x}{2}}$
$\Rightarrow \dfrac{1-\cos x}{1+\cos x}={{\tan }^{2}}\dfrac{x}{2}$
Integration of given expression is
$\Rightarrow \int{\dfrac{1-\cos x}{1+\cos x}dx}=\int{\left[ {{\tan }^{2}}\dfrac{x}{2} \right]dx}$
$\Rightarrow \int{\dfrac{1-\cos x}{1+\cos x}dx}=\int{\left[ {{\sec }^{2}}\dfrac{x}{2}-1 \right]dx}$
$\Rightarrow \int{\dfrac{1-\cos x}{1+\cos x}dx}=\left[ \dfrac{\tan \dfrac{x}{2}}{\dfrac{1}{2}}-x \right]+C$
$\therefore \int{\dfrac{1-\cos x}{1+\cos x}dx}=2\tan \dfrac{x}{2}-x+C$
9. Solve the following: $\dfrac{\cos x}{1+\cos x}$.
Ans: Given expression $\dfrac{\cos x}{1+\cos x}$.
Using the identity $\cos x={{\cos }^{2}}\dfrac{x}{2}-{{\sin }^{2}}\dfrac{x}{2}$ and $\cos x=2{{\cos }^{2}}\dfrac{x}{2}-1$ given expression can be written as
$\Rightarrow \dfrac{\cos x}{1+\cos x}=\dfrac{{{\cos }^{2}}\dfrac{x}{2}-{{\sin }^{2}}\dfrac{x}{2}}{2{{\cos }^{2}}\dfrac{x}{2}}$
$\Rightarrow \dfrac{\cos x}{1+\cos x}=\dfrac{1}{2}\left[ 1-\dfrac{{{\sin }^{2}}\dfrac{x}{2}}{{{\cos }^{2}}\dfrac{x}{2}} \right]$
$\Rightarrow \dfrac{\cos x}{1+\cos x}=\dfrac{1}{2}\left[ 1-{{\tan }^{2}}\dfrac{x}{2} \right]$
Integration of given expression is
$\Rightarrow \int{\dfrac{\cos x}{1+\cos x}dx}=\dfrac{1}{2}\int{\left[ 1-{{\tan }^{2}}\dfrac{x}{2} \right]dx}$
$\Rightarrow \int{\dfrac{\cos x}{1+\cos x}dx}=\dfrac{1}{2}\int{\left[ 1-{{\sec }^{2}}\dfrac{x}{2}+1 \right]dx}$
$\Rightarrow \int{\dfrac{\cos x}{1+\cos x}dx}=\dfrac{1}{2}\int{\left[ 2-{{\sec }^{2}}\dfrac{x}{2} \right]dx}$
$\Rightarrow \int{\dfrac{\cos x}{1+\cos x}dx}=\dfrac{1}{2}\left[ 2x-\dfrac{\tan \dfrac{x}{2}}{\dfrac{1}{2}} \right]+C$
$\therefore \int{\dfrac{\cos x}{1+\cos x}dx}=x-\tan \dfrac{x}{2}+C$
10. Solve the following: ${{\sin }^{4}}x$.
Ans: Given expression ${{\sin }^{4}}x$.
Given expression can be written as ${{\sin }^{4}}x={{\sin }^{2}}x{{\sin }^{2}}x$
$\Rightarrow {{\sin }^{4}}x=\left( \dfrac{1-\cos 2x}{2} \right)\left( \dfrac{1-\cos 2x}{2} \right)$
$\Rightarrow {{\sin }^{4}}x=\dfrac{1}{4}{{\left( 1-\cos 2x \right)}^{2}}$
$\Rightarrow {{\sin }^{4}}x=\dfrac{1}{4}\left( 1+{{\cos }^{2}}2x-2\cos 2x \right)$
$\Rightarrow {{\sin }^{4}}x=\dfrac{1}{4}\left( 1+\dfrac{1+\cos 4x}{2}-2\cos 2x \right)$
$\Rightarrow {{\sin }^{4}}x=\dfrac{1}{4}\left( 1+\dfrac{1}{2}+\dfrac{\cos 4x}{2}-2\cos 2x \right)$
$\Rightarrow {{\sin }^{4}}x=\dfrac{1}{4}\left( \dfrac{3}{2}+\dfrac{1}{2}\cos 4x-2\cos 2x \right)$
Integration of given expression is
$\Rightarrow \int{{{\sin }^{4}}x}dx=\dfrac{1}{4}\int{\left( \dfrac{3}{2}+\dfrac{1}{2}\cos 4x-2\cos 2x \right)}dx$
$\Rightarrow \int{{{\sin }^{4}}x}dx=\dfrac{1}{4}\left[ \dfrac{3}{2}x+\dfrac{\sin 4x}{8}-\sin 2x \right]+C$
$\therefore \int{{{\sin }^{4}}x}dx=\dfrac{3x}{8}+\dfrac{\sin 4x}{32}-\dfrac{1}{4}\sin 2x+C$
11. Solve the following: ${{\cos }^{4}}2x$.
Ans: Given expression ${{\cos }^{4}}2x$.
Given expression can be written as
${{\cos }^{4}}2x={{\left( {{\cos }^{2}}2x \right)}^{2}}$
$\Rightarrow {{\cos }^{4}}2x={{\left( \dfrac{1+\cos 4x}{2} \right)}^{2}}$
$\Rightarrow {{\cos }^{4}}2x=\dfrac{1}{4}\left( 1+{{\cos }^{2}}4x+2\cos 4x \right)$
$\Rightarrow {{\cos }^{4}}2x=\dfrac{1}{4}\left( 1+\dfrac{1+\cos 8x}{2}+2\cos 4x \right)$
$\Rightarrow {{\cos }^{4}}2x=\dfrac{1}{4}\left( 1+\dfrac{1}{2}+\dfrac{\cos 8x}{2}+2\cos 4x \right)$
$\Rightarrow {{\cos }^{4}}2x=\dfrac{1}{4}\left( \dfrac{3}{2}+\dfrac{\cos 8x}{2}+2\cos 4x \right)$
Integration of given expression is
$\Rightarrow \int{{{\cos }^{4}}2xdx}=\int{\left( \dfrac{3}{8}+\dfrac{\cos 8x}{8}+\dfrac{\cos 4x}{2} \right)dx}$
$\therefore \int{{{\cos }^{4}}2xdx}=\dfrac{3}{8}x+\dfrac{\sin 8x}{64}+\dfrac{\sin 4x}{8}+C$
12. Solve the following: $\dfrac{{{\sin }^{2}}x}{1+\cos x}$.
Ans: Given expression $\dfrac{{{\sin }^{2}}x}{1+\cos x}$.
By applying the identity $\sin x=2\sin \dfrac{x}{2}\cos \dfrac{x}{2}$ and $\cos x=2{{\cos }^{2}}\dfrac{x}{2}-1$, given expression can be written as
$\Rightarrow \dfrac{{{\sin }^{2}}x}{1+\cos x}=\dfrac{{{\left( 2\sin \dfrac{x}{2}\cos \dfrac{x}{2} \right)}^{2}}}{2{{\cos }^{2}}\dfrac{x}{2}}$
$\Rightarrow \dfrac{{{\sin }^{2}}x}{1+\cos x}=\dfrac{4{{\sin }^{2}}\dfrac{x}{2}{{\cos }^{2}}\dfrac{x}{2}}{2{{\cos }^{2}}\dfrac{x}{2}}$
$\Rightarrow \dfrac{{{\sin }^{2}}x}{1+\cos x}=2{{\sin }^{2}}\dfrac{x}{2}$
$\Rightarrow \dfrac{{{\sin }^{2}}x}{1+\cos x}=1-\cos x$
Integration of given expression is
$\Rightarrow \int{\dfrac{{{\sin }^{2}}x}{1+\cos x}dx}=\int{1dx-\int{\cos xdx}}$
$\therefore \int{\dfrac{{{\sin }^{2}}x}{1+\cos x}dx}=x-\sin x+C$
13. Solve the following: $\dfrac{\cos 2x-\cos 2\alpha }{\cos x-\cos \alpha }$.
Ans: Given expression $\dfrac{\cos 2x-\cos 2\alpha }{\cos x-\cos \alpha }$.
We can apply the identity $\cos C-\cos D=-2\sin \dfrac{C+D}{2}\sin \dfrac{C-D}{2}$ , we get
$\Rightarrow \dfrac{\cos 2x-\cos 2\alpha }{\cos x-\cos \alpha }=\dfrac{-2\sin \dfrac{2x+2\alpha }{2}\sin \dfrac{2x-2\alpha }{2}}{-2\sin \dfrac{x+\alpha }{2}\sin \dfrac{x-\alpha }{2}}$
$\Rightarrow \dfrac{\cos 2x-\cos 2\alpha }{\cos x-\cos \alpha }=\dfrac{\sin \dfrac{2\left( x+\alpha \right)}{2}\sin \dfrac{2\left( x-\alpha \right)}{2}}{\sin \dfrac{x+\alpha }{2}\sin \dfrac{x-\alpha }{2}}$
$\Rightarrow \dfrac{\cos 2x-\cos 2\alpha }{\cos x-\cos \alpha }=\dfrac{\sin \left( x+\alpha \right)\sin \left( x-\alpha \right)}{\sin \dfrac{x+\alpha }{2}\sin \dfrac{x-\alpha }{2}}$
We can apply the identity $\sin 2x=2\sin x\cos x$, we get
$\Rightarrow \dfrac{\cos 2x-\cos 2\alpha }{\cos x-\cos \alpha }=\dfrac{\left[ 2\sin \dfrac{x+\alpha }{2}\cos \dfrac{x+\alpha }{2} \right]\left[ 2\sin \dfrac{x-\alpha }{2}\cos \dfrac{x-\alpha }{2} \right]}{\sin \dfrac{x+\alpha }{2}\sin \dfrac{x-\alpha }{2}}$
$\Rightarrow \dfrac{\cos 2x-\cos 2\alpha }{\cos x-\cos \alpha }=4\cos \dfrac{x+\alpha }{2}\cos \dfrac{x-\alpha }{2}$
$\Rightarrow \dfrac{\cos 2x-\cos 2\alpha }{\cos x-\cos \alpha }=2\left[ \cos \dfrac{x+\alpha }{2}+\dfrac{x-\alpha }{2}+\cos \dfrac{x+\alpha }{2}-\dfrac{x-\alpha }{2} \right]$
$\Rightarrow \dfrac{\cos 2x-\cos 2\alpha }{\cos x-\cos \alpha }=2\left[ \cos x+\cos \alpha \right]$
Integration of given expression is
$\Rightarrow \int{\dfrac{\cos 2x-\cos 2\alpha }{\cos x-\cos \alpha }dx}=2\int{\left[ \cos x+\cos \alpha \right]}dx$
$\therefore \int{\dfrac{\cos 2x-\cos 2\alpha }{\cos x-\cos \alpha }dx}=2\left[ \sin x+x\cos \alpha \right]+C$
14. Solve the following: $\dfrac{\cos x-\sin x}{1+\sin 2x}$.
Ans: Given expression $\dfrac{\cos x-\sin x}{1+\sin 2x}$.
We know that ${{\sin }^{2}}x+{{\cos }^{2}}x=1$.
Given expression can be written as
$\Rightarrow \dfrac{\cos x-\sin x}{1+\sin 2x}=\dfrac{\cos x-\sin x}{{{\sin }^{2}}x+{{\cos }^{2}}x+\sin 2x}$ .
We can apply the identity $\sin 2x=2\sin x\cos x$, we get
$\Rightarrow \dfrac{\cos x-\sin x}{1+\sin 2x}=\dfrac{\cos x-\sin x}{{{\sin }^{2}}x+{{\cos }^{2}}x+2\sin x\cos x}$
$\Rightarrow \dfrac{\cos x-\sin x}{1+\sin 2x}=\dfrac{\cos x-\sin x}{{{\left( \sin x+\cos x \right)}^{2}}}$
Let $\sin x+\cos x=t$
$\therefore \left( \cos x-\sin x \right)dx=dt$
Integration of given expression is
$\Rightarrow \int{\dfrac{\cos x-\sin x}{1+\sin 2x}dx=\int{\dfrac{\cos x-\sin x}{{{\left( \sin x+\cos x \right)}^{2}}}dx}}$
$\Rightarrow \int{\dfrac{\cos x-\sin x}{1+\sin 2x}dx=\int{\dfrac{dt}{{{t}^{2}}}}}$
$\Rightarrow \int{\dfrac{\cos x-\sin x}{1+\sin 2x}dx=\int{{{t}^{-2}}dt}}$
$\Rightarrow \int{\dfrac{\cos x-\sin x}{1+\sin 2x}dx=-{{t}^{-1}}+C}$
$\Rightarrow \int{\dfrac{\cos x-\sin x}{1+\sin 2x}dx=-\dfrac{1}{t}+C}$
Substitute $\sin x+\cos x=t$,
$\therefore \int{\dfrac{\cos x-\sin x}{1+\sin 2x}dx=-\dfrac{1}{\sin x+\cos x}+C}$
15. Solve the following: ${{\tan }^{3}}2x\sec 2x$.
Ans: Given expression ${{\tan }^{3}}2x\sec 2x$.
Given expression can be written as
${{\tan }^{3}}2x\sec 2x={{\tan }^{2}}2x\tan 2x\sec 2x$
$\Rightarrow {{\tan }^{3}}2x\sec 2x=\left( {{\sec }^{2}}2x-1 \right)\tan 2x\sec 2x$
$\Rightarrow {{\tan }^{3}}2x\sec 2x={{\sec }^{2}}2x\tan 2x\sec 2x-\tan 2x\sec 2x$
Integration of given expression is
$\Rightarrow \int{{{\tan }^{3}}2x\sec 2xdx}=\int{{{\sec }^{2}}2x\tan 2x\sec 2xdx}-\int{\tan 2x\sec 2xdx}$
$\Rightarrow \int{{{\tan }^{3}}2x\sec 2xdx}=\int{{{\sec }^{2}}2x\tan 2x\sec 2xdx}-\dfrac{\sec 2x}{2}+C$
Let $\sec 2x=t$
$\therefore 2\sec 2x\tan 2xdx=dt$
Above integral becomes
$\Rightarrow \int{{{\tan }^{3}}2x\sec 2xdx}=\dfrac{1}{2}\int{{{t}^{2}}dt}-\dfrac{\sec 2x}{2}+C$
$\Rightarrow \int{{{\tan }^{3}}2x\sec 2xdx}=\dfrac{{{t}^{3}}}{6}-\dfrac{\sec 2x}{2}+C$
Substitute $\sec 2x=t$,
$\therefore \int{{{\tan }^{3}}2x\sec 2xdx}=\dfrac{{{\left( \sec 2x \right)}^{3}}}{6}-\dfrac{\sec 2x}{2}+C$
16. Solve the following: ${{\tan }^{4}}x$.
Ans: Given expression ${{\tan }^{4}}x$.
Given expression can be written as
$\Rightarrow {{\tan }^{4}}x={{\tan }^{2}}x{{\tan }^{2}}x$
$\Rightarrow {{\tan }^{4}}x=\left( {{\sec }^{2}}x-1 \right){{\tan }^{2}}x$
$\Rightarrow {{\tan }^{4}}x={{\sec }^{2}}x{{\tan }^{2}}x-{{\tan }^{2}}x$
$\Rightarrow {{\tan }^{4}}x={{\sec }^{2}}x{{\tan }^{2}}x-\left( {{\sec }^{2}}x-1 \right)$
$\Rightarrow {{\tan }^{4}}x={{\sec }^{2}}x{{\tan }^{2}}x-{{\sec }^{2}}x+1$
Integration of given expression is
$\Rightarrow \int{{{\tan }^{4}}xdx}=\int{\left( {{\sec }^{2}}x{{\tan }^{2}}x-{{\sec }^{2}}x+1 \right)}dx$
$\Rightarrow \int{{{\tan }^{4}}xdx}=\int{\left( {{\sec }^{2}}x{{\tan }^{2}}x \right)}dx-\int{{{\sec }^{2}}xdx+\int{1dx}}$
$\Rightarrow \int{{{\tan }^{4}}xdx}=\int{{{\sec }^{2}}x{{\tan }^{2}}x}dx-\tan x+x+C$
Let $\tan x=t$
$\therefore {{\sec }^{2}}xdx=dt$
$\Rightarrow \int{{{\tan }^{4}}xdx}=\int{{{t}^{2}}}dt-\tan x+x+C$
$\Rightarrow \int{{{\tan }^{4}}xdx}=\dfrac{{{t}^{3}}}{3}-\tan x+x+C$
Substitute $\tan x=t$,
$\therefore \int{{{\tan }^{4}}xdx}=\dfrac{1}{3}{{\tan }^{3}}x-\tan x+x+C$
17. Solve the following: $\dfrac{{{\sin }^{3}}x+{{\cos }^{3}}x}{{{\sin }^{2}}x{{\cos }^{2}}x}$.
Ans: Given expression $\dfrac{{{\sin }^{3}}x+{{\cos }^{3}}x}{{{\sin }^{2}}x{{\cos }^{2}}x}$.
Given expression can be written as
$\Rightarrow \dfrac{{{\sin }^{3}}x+{{\cos }^{3}}x}{{{\sin }^{2}}x{{\cos }^{2}}x}=\dfrac{{{\sin }^{3}}x}{{{\sin }^{2}}x{{\cos }^{2}}x}+\dfrac{{{\cos }^{3}}x}{{{\sin }^{2}}x{{\cos }^{2}}x}$
$\Rightarrow \dfrac{{{\sin }^{3}}x+{{\cos }^{3}}x}{{{\sin }^{2}}x{{\cos }^{2}}x}=\dfrac{\sin x}{{{\cos }^{2}}x}+\dfrac{\cos x}{{{\sin }^{2}}x}$
$\Rightarrow \dfrac{{{\sin }^{3}}x+{{\cos }^{3}}x}{{{\sin }^{2}}x{{\cos }^{2}}x}=\tan x\sec x+\cot xcosecx$
Integration of given expression is
$\Rightarrow \int{\dfrac{{{\sin }^{3}}x+{{\cos }^{3}}x}{{{\sin }^{2}}x{{\cos }^{2}}x}dx}=\int{\tan x\sec xdx}+\int{\cot x\operatorname{cosecx}dx}$
$\therefore \int{\dfrac{{{\sin }^{3}}x+{{\cos }^{3}}x}{{{\sin }^{2}}x{{\cos }^{2}}x}dx}=\sec x-cosecx+C$
18. Solve the following: $\dfrac{\cos 2x+2{{\sin }^{2}}x}{{{\cos }^{2}}x}$.
Ans: Given expression $\dfrac{\cos 2x+2{{\sin }^{2}}x}{{{\cos }^{2}}x}$.
By applying the identity $\cos 2x=1-2{{\sin }^{2}}x$, we get
$\Rightarrow \dfrac{\cos 2x+2{{\sin }^{2}}x}{{{\cos }^{2}}x}=\dfrac{\cos 2x+1-\cos 2x}{{{\cos }^{2}}x}$
$\Rightarrow \dfrac{\cos 2x+2{{\sin }^{2}}x}{{{\cos }^{2}}x}=\dfrac{1}{{{\cos }^{2}}x}$
$\Rightarrow \dfrac{\cos 2x+2{{\sin }^{2}}x}{{{\cos }^{2}}x}={{\sec }^{2}}x$
Integration of given expression is
$\Rightarrow \int{\dfrac{\cos 2x+2{{\sin }^{2}}x}{{{\cos }^{2}}x}}dx=\int{{{\sec }^{2}}xdx}$
$\therefore \int{\dfrac{\cos 2x+2{{\sin }^{2}}x}{{{\cos }^{2}}x}}dx=\tan x+C$
19. Solve the following: $\dfrac{1}{\sin x{{\cos }^{3}}x}$.
Ans: Given expression $\dfrac{1}{\sin x{{\cos }^{3}}x}$.
We can apply the identity ${{\sin }^{2}}x+{{\cos }^{2}}x=1$, we get
$\Rightarrow \dfrac{1}{\sin x{{\cos }^{3}}x}=\dfrac{{{\sin }^{2}}x+{{\cos }^{2}}x}{\sin x{{\cos }^{3}}x}$
$\Rightarrow \dfrac{1}{\sin x{{\cos }^{3}}x}=\dfrac{{{\sin }^{2}}x}{\sin x{{\cos }^{3}}x}+\dfrac{{{\cos }^{2}}x}{\sin x{{\cos }^{3}}x}$
$\Rightarrow \dfrac{1}{\sin x{{\cos }^{3}}x}=\dfrac{\sin x}{{{\cos }^{3}}x}+\dfrac{1}{\sin x\cos x}$
$\Rightarrow \dfrac{1}{\sin x{{\cos }^{3}}x}=\tan x{{\sec }^{2}}x+\dfrac{{{\cos }^{2}}x}{\dfrac{\sin x\cos x}{{{\cos }^{2}}x}}$
$\Rightarrow \dfrac{1}{\sin x{{\cos }^{3}}x}=\tan x{{\sec }^{2}}x+\dfrac{{{\sec }^{2}}x}{\tan x}$
Integration of given expression is
$\Rightarrow \int{\dfrac{1}{\sin x{{\cos }^{3}}x}}dx=\int{\tan x{{\sec }^{2}}x}dx+\int{\dfrac{{{\sec }^{2}}x}{\tan x}}dx$
Let $\tan x=t$
$\therefore {{\sec }^{2}}xdx=dt$
$\Rightarrow \int{\dfrac{1}{\sin x{{\cos }^{3}}x}}dx=\int{t}dt+\int{\dfrac{1}{\operatorname{t}}}dt$
$\Rightarrow \int{\dfrac{1}{\sin x{{\cos }^{3}}x}}dx=\dfrac{{{t}^{2}}}{2}+\log \left| t \right|+C$
Substitute $\tan x=t$,
$\therefore \int{\dfrac{1}{\sin x{{\cos }^{3}}x}}dx=\dfrac{1}{2}{{\tan }^{2}}x+\log \left| \tan x \right|+C$
20. Solve the following: $\dfrac{\cos 2x}{{{\left( \cos x+\sin x \right)}^{2}}}$.
Ans: Given expression $\dfrac{\cos 2x}{{{\left( \cos x+\sin x \right)}^{2}}}$.
Given expression can be written as
$\Rightarrow \dfrac{\cos 2x}{{{\left( \cos x+\sin x \right)}^{2}}}=\dfrac{\cos 2x}{{{\cos }^{2}}x+{{\sin }^{2}}x+2\sin x\cos x}$
We know that ${{\sin }^{2}}x+{{\cos }^{2}}x=1$ and $2\sin x\cos x=\sin 2x$, we get
$\Rightarrow \dfrac{\cos 2x}{{{\left( \cos x+\sin x \right)}^{2}}}=\dfrac{\cos 2x}{1+\sin 2x}$
Integration of given expression is
$\Rightarrow \int{\dfrac{\cos 2x}{{{\left( \cos x+\sin x \right)}^{2}}}dx}=\int{\dfrac{\cos 2x}{1+\sin 2x}dx}$
Let $1+\sin 2x=t$
$\therefore 2\cos 2xdx=dt$
Integration becomes
$\Rightarrow \int{\dfrac{\cos 2x}{{{\left( \cos x+\sin x \right)}^{2}}}dx}=\dfrac{1}{2}\int{\dfrac{1}{t}dt}$
$\Rightarrow \int{\dfrac{\cos 2x}{{{\left( \cos x+\sin x \right)}^{2}}}dx}=\dfrac{1}{2}\log \left| t \right|+C$
Substitute $1+\sin 2x=t$
$\Rightarrow \int{\dfrac{\cos 2x}{{{\left( \cos x+\sin x \right)}^{2}}}dx}=\dfrac{1}{2}\log \left| 1+\sin 2x \right|+C$
$\Rightarrow \int{\dfrac{\cos 2x}{{{\left( \cos x+\sin x \right)}^{2}}}dx}=\dfrac{1}{2}\log \left| {{\left( \cos x+\sin x \right)}^{2}} \right|+C$
$\therefore \int{\dfrac{\cos 2x}{{{\left( \cos x+\sin x \right)}^{2}}}dx}=\log \left| \left( \cos x+\sin x \right) \right|+C$
21. Solve the following: ${{\sin }^{-1}}\left( \cos x \right)$.
Ans: Given expression ${{\sin }^{-1}}\left( \cos x \right)$.
Let $\cos x=t$
$\therefore \sin x=\sqrt{1-{{t}^{2}}}$
$\Rightarrow -\sin xdx=dt$
$\Rightarrow dx=-\dfrac{dt}{\sin x}$
$\Rightarrow dx=-\dfrac{dt}{\sqrt{1-{{t}^{2}}}}$
Integration of given expression is
$\Rightarrow \int{{{\sin }^{-1}}\left( \cos x \right)dx}=\int{{{\sin }^{-1}}t\left( \dfrac{-dt}{\sqrt{1-{{t}^{2}}}} \right)}$
$\Rightarrow \int{{{\sin }^{-1}}\left( \cos x \right)dx}=-\int{\left( \dfrac{{{\sin }^{-1}}t}{\sqrt{1-{{t}^{2}}}} \right)dt}$
Let ${{\sin }^{-1}}t=u$
$\Rightarrow \dfrac{1}{\sqrt{1-{{t}^{2}}}}dt=du$
Integration becomes
$\Rightarrow \int{{{\sin }^{-1}}\left( \cos x \right)dx}=\int{4du}$
$\Rightarrow \int{{{\sin }^{-1}}\left( \cos x \right)dx}=-\dfrac{{{u}^{2}}}{2}+C$
Substitute ${{\sin }^{-1}}t=u$
$\Rightarrow \int{{{\sin }^{-1}}\left( \cos x \right)dx}=-\dfrac{{{\left( {{\sin }^{-1}}t \right)}^{2}}}{2}+C$
Substitute $\cos x=t$
$\Rightarrow \int{{{\sin }^{-1}}\left( \cos x \right)dx}=-\dfrac{{{\left[ {{\sin }^{-1}}\left( \cos x \right) \right]}^{2}}}{2}+C$ ……..(1)
We know that ${{\sin }^{-1}}x+{{\cos }^{-1}}x=\dfrac{\pi }{2}$
$\therefore {{\sin }^{-1}}\left( \cos x \right)=\dfrac{\pi }{2}-{{\cos }^{-1}}\left( \cos x \right)=\left( \dfrac{\pi }{2}-x \right)$
Substitute in eq. (1), we get
$\Rightarrow \int{{{\sin }^{-1}}\left( \cos x \right)dx}=\dfrac{-{{\left( \dfrac{\pi }{2}-x \right)}^{2}}}{2}+C$
$\Rightarrow \int{{{\sin }^{-1}}\left( \cos x \right)dx}=\dfrac{1}{2}\left( \dfrac{{{\pi }^{2}}}{2}+{{x}^{2}}-\pi x \right)+C$
$\Rightarrow \int{{{\sin }^{-1}}\left( \cos x \right)dx}=-\dfrac{{{\pi }^{2}}}{4}-\dfrac{{{x}^{2}}}{2}+\dfrac{1}{2}\pi x+C$
$\Rightarrow \int{{{\sin }^{-1}}\left( \cos x \right)dx}=\dfrac{\pi x}{2}-\dfrac{{{x}^{2}}}{2}+\left( C-\dfrac{{{\pi }^{2}}}{4} \right)$
$\therefore \int{{{\sin }^{-1}}\left( \cos x \right)dx}=\dfrac{\pi x}{2}-\dfrac{{{x}^{2}}}{2}+{{C}_{1}}$
22. Solve the following: $\dfrac{1}{\cos \left( x-a \right)\cos \left( x-b \right)}$.
Ans: Given expression $\dfrac{1}{\cos \left( x-a \right)\cos \left( x-b \right)}$.
Given expression can be written as
$\Rightarrow \dfrac{1}{\cos \left( x-a \right)\cos \left( x-b \right)}=\dfrac{1}{\sin \left( a-b \right)}\left[ \dfrac{\sin \left( a-b \right)}{\cos \left( x-a \right)\cos \left( x-b \right)} \right]$
$\Rightarrow \dfrac{1}{\cos \left( x-a \right)\cos \left( x-b \right)}=\dfrac{1}{\sin \left( a-b \right)}\left[ \dfrac{\sin \left[ \left( x-b \right)-\left( x-a \right) \right]}{\cos \left( x-a \right)\cos \left( x-b \right)} \right]$
$\Rightarrow \dfrac{1}{\cos \left( x-a \right)\cos \left( x-b \right)}=\dfrac{1}{\sin \left( a-b \right)}\left[ \dfrac{\sin \left( x-b \right)\cos \left( x-a \right)-\cos \left( x-b \right)\sin \left( x-a \right)}{\cos \left( x-a \right)\cos \left( x-b \right)} \right]$
$\Rightarrow \dfrac{1}{\cos \left( x-a \right)\cos \left( x-b \right)}=\dfrac{1}{\sin \left( a-b \right)}\left[ \tan \left( x-b \right)-\tan \left( x-b \right) \right]$
Integration of given expression is
$\Rightarrow \int{\dfrac{1}{\cos \left( x-a \right)\cos \left( x-b \right)}dx}=\int{\dfrac{1}{\sin \left( a-b \right)}\left[ \tan \left( x-b \right)-\tan \left( x-b \right) \right]dx}$
$\Rightarrow \int{\dfrac{1}{\cos \left( x-a \right)\cos \left( x-b \right)}dx}=\int{\dfrac{1}{\sin \left( a-b \right)}\left[ -\log \left| \cos \left( x-b \right) \right|+\log \cos \left( x-a \right) \right]}$
$\Rightarrow \int{\dfrac{1}{\cos \left( x-a \right)\cos \left( x-b \right)}dx}=\int{\dfrac{1}{\sin \left( a-b \right)}\left[ \log \left| \dfrac{\cos \left( x-a \right)}{\cos \left( x-b \right)} \right| \right]}+C$
23. Solve the following: $\int{\dfrac{{{\sin }^{2}}x-{{\cos }^{2}}x}{{{\sin }^{2}}x{{\cos }^{2}}x}dx}$ is equal to
$\tan x+\cot x+C$
$\tan x+cosecx+C$
$-\tan x+\cot x+C$
$\tan x+\sec x+C$
Ans: Given expression $\int{\dfrac{{{\sin }^{2}}x-{{\cos }^{2}}x}{{{\sin }^{2}}x{{\cos }^{2}}x}dx}$.
Given expression can be written as
$\Rightarrow \int{\dfrac{{{\sin }^{2}}x-{{\cos }^{2}}x}{{{\sin }^{2}}x{{\cos }^{2}}x}dx}=\int{\dfrac{{{\sin }^{2}}x}{{{\sin }^{2}}x{{\cos }^{2}}x}dx}-\int{\dfrac{{{\cos }^{2}}x}{{{\sin }^{2}}x{{\cos }^{2}}x}dx}$
$\Rightarrow \int{\dfrac{{{\sin }^{2}}x-{{\cos }^{2}}x}{{{\sin }^{2}}x{{\cos }^{2}}x}dx}=\int{{{\sec }^{2}}xdx}-\int{cose{{c}^{2}}xdx}$
$\therefore \int{\dfrac{{{\sin }^{2}}x-{{\cos }^{2}}x}{{{\sin }^{2}}x{{\cos }^{2}}x}dx}=\tan x+\cot x+C$
Therefore, option A is the correct answer.
24. Solve the following: $\int{\dfrac{{{e}^{x}}\left( 1+x \right)}{{{\cos }^{2}}\left( {{e}^{x}}x \right)}dx}$ equals
$-\cot \left( e{{x}^{x}} \right)+C$
$\tan \left( x{{e}^{x}} \right)+C$
$\tan \left( {{e}^{x}} \right)+C$
$\cot \left( {{e}^{x}} \right)+C$
Ans: Given expression $\int{\dfrac{{{e}^{x}}\left( 1+x \right)}{{{\cos }^{2}}\left( {{e}^{x}}x \right)}dx}$.
Let ${{e}^{x}}x=t$
$\therefore \left( {{e}^{x}}.x+{{e}^{x}}.1 \right)dx=dt$
$\Rightarrow {{e}^{x}}\left( x+1 \right)dx=dt$
Integration of given expression is
$\Rightarrow \int{\dfrac{{{e}^{x}}\left( 1+x \right)}{{{\cos }^{2}}\left( {{e}^{x}}x \right)}dx}=\int{\dfrac{dt}{{{\cos }^{2}}t}}$
$\Rightarrow \int{\dfrac{{{e}^{x}}\left( 1+x \right)}{{{\cos }^{2}}\left( {{e}^{x}}x \right)}dx}=\int{{{\sec }^{2}}t}dt$
$\Rightarrow \int{\dfrac{{{e}^{x}}\left( 1+x \right)}{{{\cos }^{2}}\left( {{e}^{x}}x \right)}dx}=\tan t+C$
Substitute ${{e}^{x}}x=t$,
$\therefore \int{\dfrac{{{e}^{x}}\left( 1+x \right)}{{{\cos }^{2}}\left( {{e}^{x}}x \right)}dx}=\tan \left( {{e}^{x}}x \right)+C$
Therefore, option B is the correct answer.
Exercise 7.4
1. Solve the following: $\dfrac{3{{x}^{2}}}{{{x}^{6}}+1}$.
Ans: Given expression $\dfrac{3{{x}^{2}}}{{{x}^{6}}+1}$.
Let ${{x}^{3}}=t$
$\therefore 3{{x}^{2}}dx=dt$
Integration of given expression is
$\Rightarrow \int{\dfrac{3{{x}^{2}}}{{{x}^{6}}+1}dx=\int{\dfrac{dt}{{{t}^{2}}+1}}}$
We know that $\int{\dfrac{1}{1+{{x}^{2}}}={{\tan }^{-1}}x}$
$\Rightarrow \int{\dfrac{3{{x}^{2}}}{{{x}^{6}}+1}dx={{\tan }^{-1}}t+C}$
Substitute ${{x}^{3}}=t$,
$\therefore \int{\dfrac{3{{x}^{2}}}{{{x}^{6}}+1}dx={{\tan }^{-1}}\left( {{x}^{3}} \right)+C}$
2. Solve the following: $\dfrac{1}{\sqrt{1+4{{x}^{2}}}}$.
Ans: Given expression $\dfrac{1}{\sqrt{1+4{{x}^{2}}}}$.
Let $2x=t$
$\therefore 2dx=dt$
Integration of given expression is
$\Rightarrow \int{\dfrac{1}{\sqrt{1+4{{x}^{2}}}}dx=\dfrac{1}{2}\int{\dfrac{dt}{\sqrt{1+{{t}^{2}}}}}}$
We know that $\int{\dfrac{1}{\sqrt{{{x}^{2}}+{{a}^{2}}}}}dt=\log \left| x+\sqrt{{{x}^{2}}+{{a}^{2}}} \right|$
$\Rightarrow \int{\dfrac{1}{\sqrt{1+4{{x}^{2}}}}dx=\dfrac{1}{2}\log \left| t+\sqrt{{{t}^{2}}+1} \right|}+C$
Substitute $2x=t$,
$\therefore \int{\dfrac{1}{\sqrt{1+4{{x}^{2}}}}dx=\dfrac{1}{2}\log \left| 2x+\sqrt{4{{x}^{2}}+1} \right|}+C$
3. Solve the following: $\dfrac{1}{\sqrt{{{\left( 2-x \right)}^{2}}+1}}$.
Ans: Given expression $\dfrac{1}{\sqrt{{{\left( 2-x \right)}^{2}}+1}}$.
Let $2-x=t$
$\therefore -dx=dt$
Integration of given expression is
$\Rightarrow \int{\dfrac{1}{\sqrt{{{\left( 2-x \right)}^{2}}+1}}dx=-\int{\dfrac{1}{\sqrt{{{t}^{2}}+1}}dt}}$
We know that $\int{\dfrac{1}{\sqrt{{{x}^{2}}+{{a}^{2}}}}}dt=\log \left| x+\sqrt{{{x}^{2}}+{{a}^{2}}} \right|$
$\Rightarrow \int{\dfrac{1}{\sqrt{{{\left( 2-x \right)}^{2}}+1}}dx=-\log \left| t+\sqrt{{{t}^{2}}+1} \right|+C}$
Substitute $2-x=t$,
$\Rightarrow \int{\dfrac{1}{\sqrt{{{\left( 2-x \right)}^{2}}+1}}dx=-\log \left| \left( 2-x \right)+\sqrt{{{\left( 2-x \right)}^{2}}+1} \right|+C}$
$\therefore \int{\dfrac{1}{\sqrt{{{\left( 2-x \right)}^{2}}+1}}dx=\log \left| \dfrac{1}{\left( 2-x \right)+\sqrt{{{x}^{2}}-4x+5}} \right|+C}$
4. Solve the following: $\dfrac{1}{\sqrt{9-25{{x}^{2}}}}$.
Ans: Given expression $\dfrac{1}{\sqrt{9-25{{x}^{2}}}}$.
Let $5x=t$
$\therefore 5dx=dt$
Integration of given expression is
$\Rightarrow \int{\dfrac{1}{\sqrt{9-25{{x}^{2}}}}dx=\dfrac{1}{5}\int{\dfrac{1}{\sqrt{9-{{t}^{2}}}}dt}}$
$\Rightarrow \int{\dfrac{1}{\sqrt{9-25{{x}^{2}}}}dx=\dfrac{1}{5}\int{\dfrac{1}{\sqrt{{{3}^{2}}-{{t}^{2}}}}dt}}$
$\Rightarrow \int{\dfrac{1}{\sqrt{9-25{{x}^{2}}}}dx=\dfrac{1}{5}{{\sin }^{-1}}\left( \dfrac{t}{3} \right)+C}$
Substitute $5x=t$,
$\therefore \int{\dfrac{1}{\sqrt{9-25{{x}^{2}}}}dx=\dfrac{1}{5}{{\sin }^{-1}}\left( \dfrac{5x}{3} \right)+C}$
5. Solve the following: $\dfrac{3x}{1+2{{x}^{4}}}$.
Ans: Given expression $\dfrac{3x}{1+2{{x}^{4}}}$.
Let $\sqrt{2}{{x}^{2}}=t$
$\therefore 2\sqrt{2}dx=dt$
Integration of given expression is
$\Rightarrow \int{\dfrac{3x}{1+2{{x}^{4}}}dx}=\dfrac{3}{2\sqrt{2}}\int{\dfrac{dt}{1+{{t}^{2}}}}$
$\Rightarrow \int{\dfrac{3x}{1+2{{x}^{4}}}dx}=\dfrac{3}{2\sqrt{2}}{{\tan }^{-1}}t+C$
Substitute $\sqrt{2}{{x}^{2}}=t$,
$\therefore \int{\dfrac{3x}{1+2{{x}^{4}}}dx}=\dfrac{3}{2\sqrt{2}}{{\tan }^{-1}}\left( \sqrt{2}{{x}^{2}} \right)+C$
6. Solve the following: $\dfrac{{{x}^{2}}}{1-{{x}^{6}}}$.
Ans: Given expression $\dfrac{{{x}^{2}}}{1-{{x}^{6}}}$.
Let ${{x}^{3}}=t$
$\therefore 3{{x}^{2}}dx=dt$
Integration of given expression is
$\Rightarrow \int{\dfrac{{{x}^{2}}}{1-{{x}^{6}}}dx=\dfrac{1}{3}\int{\dfrac{dt}{1-{{t}^{2}}}}}$
$\Rightarrow \int{\dfrac{{{x}^{2}}}{1-{{x}^{6}}}dx=\dfrac{1}{3}\left[ \dfrac{1}{2}\log \left| \dfrac{1+t}{1-t} \right| \right]+C}$
Substitute ${{x}^{3}}=t$,
$\therefore \int{\dfrac{{{x}^{2}}}{1-{{x}^{6}}}dx=\dfrac{1}{3}\left[ \dfrac{1}{2}\log \left| \dfrac{1+{{x}^{3}}}{1-{{x}^{3}}} \right| \right]+C}$
7. Solve the following: $\dfrac{x-1}{\sqrt{{{x}^{2}}-1}}$.
Ans: Given expression $\dfrac{x-1}{\sqrt{{{x}^{2}}-1}}$.
Given expression can be written as
$\Rightarrow \dfrac{x-1}{\sqrt{{{x}^{2}}-1}}=\dfrac{x}{\sqrt{{{x}^{2}}-1}}-\dfrac{1}{\sqrt{{{x}^{2}}-1}}$
Integration of given expression is
$\Rightarrow \int{\dfrac{x-1}{\sqrt{{{x}^{2}}-1}}dx}=\int{\dfrac{x}{\sqrt{{{x}^{2}}-1}}dx}-\int{\dfrac{1}{\sqrt{{{x}^{2}}-1}}dx}$
$\Rightarrow \int{\dfrac{x-1}{\sqrt{{{x}^{2}}-1}}dx}=\int{\dfrac{x}{\sqrt{{{x}^{2}}-1}}dx}-\log \left| x+\sqrt{{{x}^{2}}-1} \right|+C$
Let ${{x}^{2}}-1=t$
$\therefore 2xdx=dt$
Integration becomes
$\Rightarrow \int{\dfrac{x-1}{\sqrt{{{x}^{2}}-1}}dx}=\dfrac{1}{2}\int{\dfrac{dt}{\sqrt{t}}}-\log \left| x+\sqrt{{{x}^{2}}-1} \right|+C$
$\Rightarrow \int{\dfrac{x-1}{\sqrt{{{x}^{2}}-1}}dx}=\dfrac{1}{2}\int{{{t}^{\dfrac{1}{2}}}dt}-\log \left| x+\sqrt{{{x}^{2}}-1} \right|+C$
$\Rightarrow \int{\dfrac{x-1}{\sqrt{{{x}^{2}}-1}}dx}=\dfrac{1}{2}\left( 2{{t}^{\dfrac{1}{2}}} \right)-\log \left| x+\sqrt{{{x}^{2}}-1} \right|+C$
$\Rightarrow \int{\dfrac{x-1}{\sqrt{{{x}^{2}}-1}}dx}=\sqrt{t}-\log \left| x+\sqrt{{{x}^{2}}-1} \right|+C$
Substitute ${{x}^{2}}-1=t$
$\Rightarrow \int{\dfrac{x-1}{\sqrt{{{x}^{2}}-1}}dx}=\sqrt{{{x}^{2}}-1}-\log \left| x+\sqrt{{{x}^{2}}-1} \right|+C$
8. Solve the following: $\dfrac{{{x}^{2}}}{\sqrt{{{x}^{6}}+{{a}^{6}}}}$.
Ans: Given expression $\dfrac{{{x}^{2}}}{\sqrt{{{x}^{6}}+{{a}^{6}}}}$.
Let ${{x}^{3}}=t$
$\therefore 3{{x}^{2}}dx=dt$
Integration of given expression is
$\Rightarrow \int{\dfrac{{{x}^{2}}}{\sqrt{{{x}^{6}}+{{a}^{6}}}}dx=\dfrac{1}{3}\int{\dfrac{dt}{\sqrt{{{t}^{2}}+{{\left( {{a}^{3}} \right)}^{2}}}}}}$
$\Rightarrow \int{\dfrac{{{x}^{2}}}{\sqrt{{{x}^{6}}+{{a}^{6}}}}dx=\dfrac{1}{3}\log \left| t+\sqrt{{{t}^{2}}+{{a}^{6}}} \right|+C}$
Substitute ${{x}^{3}}=t$,
$\therefore \int{\dfrac{{{x}^{2}}}{\sqrt{{{x}^{6}}+{{a}^{6}}}}dx=\dfrac{1}{3}\log \left| {{x}^{3}}+\sqrt{{{x}^{6}}+{{a}^{6}}} \right|+C}$
9. Solve the following: $\dfrac{{{\sec }^{2}}x}{\sqrt{{{\tan }^{2}}x+4}}$.
Ans: Given expression $\dfrac{{{\sec }^{2}}x}{\sqrt{{{\tan }^{2}}x+4}}$.
Let $\tan x=t$
$\therefore {{\sec }^{2}}xdx=dt$
Integration of given expression is
$\Rightarrow \int{\dfrac{{{\sec }^{2}}x}{\sqrt{{{\tan }^{2}}x+4}}dx=\int{\dfrac{dt}{\sqrt{{{t}^{2}}+{{2}^{2}}}}}}$
$\Rightarrow \int{\dfrac{{{\sec }^{2}}x}{\sqrt{{{\tan }^{2}}x+4}}dx=\log \left| t+\sqrt{{{\operatorname{t}}^{2}}+4} \right|}+C$
Substitute $\tan x=t$,
$\therefore \int{\dfrac{{{\sec }^{2}}x}{\sqrt{{{\tan }^{2}}x+4}}dx=\log \left| \tan x+\sqrt{{{\tan }^{2}}x+4} \right|}+C$
10. Solve the following: $\dfrac{1}{\sqrt{{{x}^{2}}+2x+2}}$.
Ans: Given expression $\dfrac{1}{\sqrt{{{x}^{2}}+2x+2}}$.
Given expression can be written as
$\dfrac{1}{\sqrt{{{x}^{2}}+2x+2}}=\dfrac{1}{\sqrt{{{\left( x+1 \right)}^{2}}+{{\left( 1 \right)}^{2}}}}$
Let $x+1=t$
$\therefore dx=dt$
Integration of given expression is
$\Rightarrow \int{\dfrac{1}{\sqrt{{{x}^{2}}+2x+2}}dx}=\int{\dfrac{1}{\sqrt{{{t}^{2}}+1}}dt}$
$\Rightarrow \int{\dfrac{1}{\sqrt{{{x}^{2}}+2x+2}}dx}=\log \left| t+\sqrt{{{t}^{2}}+1} \right|+C$
Substitute $x+1=t$,
$\Rightarrow \int{\dfrac{1}{\sqrt{{{x}^{2}}+2x+2}}dx}=\log \left| \left( x+1 \right)+\sqrt{{{\left( x+1 \right)}^{2}}+1} \right|+C$
$\therefore \int{\dfrac{1}{\sqrt{{{x}^{2}}+2x+2}}dx}=\log \left| \left( x+1 \right)+\sqrt{{{x}^{2}}+2x+2} \right|+C$
11. Solve the following: $\dfrac{1}{\sqrt{9{{x}^{2}}+6x+5}}$.
Ans: Given expression $\dfrac{1}{\sqrt{9{{x}^{2}}+6x+5}}$.
Given expression can be written as
$\dfrac{1}{\sqrt{9{{x}^{2}}+6x+5}}=\dfrac{1}{\sqrt{{{\left( 3x+1 \right)}^{2}}+{{\left( 2 \right)}^{2}}}}$
Let $3x+1=t$
$\therefore 3dx=dt$
Integration of given expression is
$\Rightarrow \int{\dfrac{1}{\sqrt{9{{x}^{2}}+6x+5}}dx=\dfrac{1}{3}\int{\dfrac{1}{\sqrt{{{t}^{2}}+{{2}^{2}}}}dt}}$
$\Rightarrow \int{\dfrac{1}{\sqrt{9{{x}^{2}}+6x+5}}dx=\dfrac{1}{3}\left[ \dfrac{1}{2}{{\tan }^{-1}}\left( \dfrac{t}{2} \right) \right]+C}$
Substitute $3x+1=t$,
$\therefore \int{\dfrac{1}{\sqrt{9{{x}^{2}}+6x+5}}dx=\dfrac{1}{3}\left[ \dfrac{1}{2}{{\tan }^{-1}}\left( \dfrac{3x+1}{2} \right) \right]+C}$
12. Solve the following: $\dfrac{1}{\sqrt{7-6x-{{x}^{2}}}}$.
Ans: Given expression $\dfrac{1}{\sqrt{7-6x-{{x}^{2}}}}$.
Given expression can be written as
$\Rightarrow \dfrac{1}{\sqrt{7-6x-{{x}^{2}}}}=\dfrac{1}{\sqrt{7-\left( {{x}^{2}}+6x+9-9 \right)}}$
$\Rightarrow \dfrac{1}{\sqrt{7-6x-{{x}^{2}}}}=\dfrac{1}{\sqrt{16-\left( {{x}^{2}}+6x+9 \right)}}$
$\Rightarrow \dfrac{1}{\sqrt{7-6x-{{x}^{2}}}}=\dfrac{1}{\sqrt{16-{{\left( x+3 \right)}^{2}}}}$
$\Rightarrow \dfrac{1}{\sqrt{7-6x-{{x}^{2}}}}=\dfrac{1}{\sqrt{{{4}^{2}}-{{\left( x+3 \right)}^{2}}}}$
Let $x+3=t$
$\therefore dx=dt$
Integration of given expression is
$\Rightarrow \int{\dfrac{1}{\sqrt{7-6x-{{x}^{2}}}}dx}=\int{\dfrac{1}{\sqrt{{{4}^{2}}-{{\left( t \right)}^{2}}}}dt}$
$\Rightarrow \int{\dfrac{1}{\sqrt{7-6x-{{x}^{2}}}}dx}={{\sin }^{-1}}\left( \dfrac{t}{4} \right)+C$
Substitute $x+3=t$,
$\therefore \int{\dfrac{1}{\sqrt{7-6x-{{x}^{2}}}}dx}={{\sin }^{-1}}\left( \dfrac{x+3}{4} \right)+C$
13. Solve the following: $\dfrac{1}{\sqrt{\left( x-1 \right)\left( x-2 \right)}}$.
Ans: Given expression $\dfrac{1}{\sqrt{\left( x-1 \right)\left( x-2 \right)}}$.
Given expression can be written as
$\Rightarrow \dfrac{1}{\sqrt{\left( x-1 \right)\left( x-2 \right)}}=\dfrac{1}{\sqrt{{{x}^{2}}-3x+2}}$
$\Rightarrow \dfrac{1}{\sqrt{\left( x-1 \right)\left( x-2 \right)}}=\dfrac{1}{\sqrt{{{x}^{2}}-3x+\dfrac{9}{4}-\dfrac{9}{4}+2}}$
$\Rightarrow \dfrac{1}{\sqrt{\left( x-1 \right)\left( x-2 \right)}}=\dfrac{1}{\sqrt{{{\left( x-\dfrac{3}{2} \right)}^{2}}-{{\left( \dfrac{1}{2} \right)}^{2}}}}$
Let $x-\dfrac{3}{2}=t$
$\therefore dx=dt$
$\Rightarrow \int{\dfrac{1}{\sqrt{\left( x-1 \right)\left( x-2 \right)}}dx}=\int{\dfrac{1}{\sqrt{{{t}^{2}}-{{\left( \dfrac{1}{2} \right)}^{2}}}}dx}$
$\Rightarrow \int{\dfrac{1}{\sqrt{\left( x-1 \right)\left( x-2 \right)}}dx}=\log \left| t+\sqrt{{{t}^{2}}-{{\left( \dfrac{1}{2} \right)}^{2}}} \right|+C$
Substitute $x-\dfrac{3}{2}=t$,
$\therefore \int{\dfrac{1}{\sqrt{\left( x-1 \right)\left( x-2 \right)}}dx}=\log \left| \left( x-\dfrac{3}{2} \right)+\sqrt{{{x}^{2}}-3x+2} \right|+C$
14. Solve the following: $\dfrac{1}{\sqrt{8+3x-{{x}^{2}}}}$.
Ans: Given expression $\dfrac{1}{\sqrt{8+3x-{{x}^{2}}}}$.
Given expression can be written as
$\Rightarrow \dfrac{1}{\sqrt{8+3x-{{x}^{2}}}}=\dfrac{1}{\sqrt{8-\left( {{x}^{2}}-3x+\dfrac{9}{4}-\dfrac{9}{4} \right)}}$
$\Rightarrow \dfrac{1}{\sqrt{8+3x-{{x}^{2}}}}=\dfrac{1}{\sqrt{8-\left( {{x}^{2}}-3x+\dfrac{9}{4}-\dfrac{9}{4} \right)}}$
$\Rightarrow \dfrac{1}{\sqrt{8+3x-{{x}^{2}}}}=\dfrac{1}{\sqrt{\dfrac{41}{4}-{{\left( x-\dfrac{3}{2} \right)}^{2}}}}$
Let $x-\dfrac{3}{2}=t$
$\therefore dx=dt$
$\Rightarrow \int{\dfrac{1}{\sqrt{8+3x-{{x}^{2}}}}dx}=\int{\dfrac{1}{\sqrt{{{\left( \dfrac{\sqrt{41}}{2} \right)}^{2}}-{{\left( t \right)}^{2}}}}dt}$
$\Rightarrow \int{\dfrac{1}{\sqrt{8+3x-{{x}^{2}}}}dx}={{\sin }^{-1}}\left( \dfrac{t}{\dfrac{\sqrt{41}}{2}} \right)+C$
Substitute $x-\dfrac{3}{2}=t$
$\Rightarrow \int{\dfrac{1}{\sqrt{8+3x-{{x}^{2}}}}dx}={{\sin }^{-1}}\left( \dfrac{x-\dfrac{3}{2}}{\dfrac{\sqrt{41}}{2}} \right)+C$
$\therefore \int{\dfrac{1}{\sqrt{8+3x-{{x}^{2}}}}dx}={{\sin }^{-1}}\left( \dfrac{2x-3}{\sqrt{41}} \right)+C$
15. Solve the following: $\dfrac{1}{\sqrt{\left( x-a \right)\left( x-b \right)}}$.
Ans: Given expression $\dfrac{1}{\sqrt{\left( x-a \right)\left( x-b \right)}}$.
Given expression can be written as
$\Rightarrow \dfrac{1}{\sqrt{\left( x-a \right)\left( x-b \right)}}=\dfrac{1}{\sqrt{{{x}^{2}}-\left( a+b \right)x+ab}}$
$\Rightarrow \dfrac{1}{\sqrt{\left( x-a \right)\left( x-b \right)}}=\dfrac{1}{\sqrt{{{x}^{2}}-\left( a+b \right)x+\dfrac{{{\left( a+b \right)}^{2}}}{4}-\dfrac{{{\left( a+b \right)}^{2}}}{4}+ab}}$
$\Rightarrow \dfrac{1}{\sqrt{\left( x-a \right)\left( x-b \right)}}=\dfrac{1}{\sqrt{{{\left[ x-\dfrac{\left( a+b \right)}{4} \right]}^{2}}-\dfrac{{{\left( a+b \right)}^{2}}}{4}}}$
$\Rightarrow \dfrac{1}{\sqrt{\left( x-a \right)\left( x-b \right)}}=\dfrac{1}{\sqrt{{{\left[ x-\dfrac{\left( a+b \right)}{4} \right]}^{2}}-{{\left( \dfrac{a+b}{2} \right)}^{2}}}}$
Integration of given expression is
$\Rightarrow \int{\dfrac{1}{\sqrt{\left( x-a \right)\left( x-b \right)}}dx}=\int{\dfrac{1}{\sqrt{{{\left[ x-\dfrac{\left( a+b \right)}{4} \right]}^{2}}-{{\left( \dfrac{a+b}{2} \right)}^{2}}}}dx}$
Let $x-\left( \dfrac{a+b}{2} \right)=t$
$\therefore dx=dt$
$\Rightarrow \int{\dfrac{1}{\sqrt{\left( x-a \right)\left( x-b \right)}}dx}=\int{\dfrac{1}{\sqrt{{{t}^{2}}-{{\left( \dfrac{a+b}{2} \right)}^{2}}}}dx}$
$\Rightarrow \int{\dfrac{1}{\sqrt{\left( x-a \right)\left( x-b \right)}}dx}=\log \left| t+\sqrt{{{t}^{2}}-{{\left( \dfrac{a+b}{2} \right)}^{2}}} \right|+C$
Substitute $x-\left( \dfrac{a+b}{2} \right)=t$,
$\therefore \int{\dfrac{1}{\sqrt{\left( x-a \right)\left( x-b \right)}}dx}=\log \left| x-\left( \dfrac{a+b}{2} \right)+\sqrt{\left( x-a \right)\left( x-b \right)} \right|+C$
16. $\dfrac{4x+1}{\sqrt{2{{x}^{2}}+x-3}}$
Ans:Consider $4x+1=A\dfrac{d}{dx}\left( 2{{x}^{2}}+x-3 \right)+B$
Simplifying,
$\Rightarrow 4x+1=A(4x+1)+B$
$\Rightarrow 4x+1=4Ax+A+B$
We obtain the below values by equating the coefficients of $\text{x}$ and the constant term on both sides.
$4~\text{A}=4\Rightarrow \text{A}=1$
$\text{A}+\text{B}=1\Rightarrow \text{B}=0$
Consider $2{{x}^{2}}+x-3=t$
$\therefore (4x+1)dx=dt$
$\Rightarrow \int{\dfrac{4x+1}{\sqrt{2{{x}^{2}}+x-3}}}dx=\int{\dfrac{1}{\sqrt{t}}}dt$
Using the power rule of integration,
$=2\sqrt{t}+C$
Substitute the value of t,
$=2\sqrt{2{{x}^{2}}+x-3}+C$
17. $\dfrac{x+2}{\sqrt{{{x}^{2}}-1}}$
Ans:Consider $\text{x}+2=A\dfrac{d}{dx}\left( {{x}^{2}}-1 \right)+B$
$\Rightarrow x+2=A(2x)+B......\left( 1 \right)$
We obtain the below values by equating the coefficients of x and the constant term on both sides.
$2A=1\Rightarrow A=\dfrac{1}{2}$
$B=2$
From (1), we get
$(x+2)=\dfrac{1}{2}(2x)+2$
$\int{\dfrac{x+2}{\sqrt{{{x}^{2}}-1}}}dx=\int{\dfrac{\dfrac{1}{2}(2x)+2}{\sqrt{{{x}^{2}}-1}}}dx$
$=\dfrac{1}{2}\int{\dfrac{2x}{\sqrt{{{x}^{2}}-1}}}dx+\int{\dfrac{2}{\sqrt{{{x}^{2}}-1}}}dx$
$\text{In }\dfrac{1}{2}\int{\dfrac{2x}{\sqrt{{{x}^{2}}-1}}}dx\text{ let }{{x}^{2}}-1=t\Rightarrow 2xdx=dt$
$\dfrac{1}{2}\int{\dfrac{2x}{\sqrt{{{x}^{2}}-1}}}dx=\dfrac{1}{2}\int{\dfrac{dt}{\sqrt{t}}}\text{ }$
Integrating using the power rule
$=\dfrac{1}{2}[2\sqrt{t}]$
Simplifying,
$=\sqrt{t}\text{ }$
Substitute the value of t,
$=\sqrt{{{x}^{2}}-1}$
Then, $\int{\dfrac{2}{\sqrt{{{x}^{2}}-1}}}dx=2\int{\dfrac{1}{\sqrt{{{x}^{2}}-1}}}dx=2\log \left| x+\sqrt{{{x}^{2}}-1} \right|$
From equation (2), we get
$\int{\dfrac{x+2}{\sqrt{{{x}^{2}}-1}}}dx=\sqrt{{{x}^{2}}-1}+2\log \left| x+\sqrt{{{x}^{2}}-1} \right|+C$
18. $\dfrac{5x-2}{1+2x+3{{x}^{2}}}$
Ans: Let $5x-2=A\dfrac{d}{dx}\left( 1+2x+3{{x}^{2}} \right)+B\text{ }$
$\Rightarrow \text{ }5\text{ }x-2=A\left( 2+6\text{ }x \right)+B......\left( 1 \right)$
We obtain the below values by equating the coefficients of x and
the constant term on both sides.
$5=6A\Rightarrow A=\dfrac{5}{6}$
$2A+B=-2\Rightarrow B=-\dfrac{11}{3}$
Substitute the above values in (1)
$\therefore 5x-2=\dfrac{5}{6}(2+6x)+\left( -\dfrac{11}{3} \right)$
$\Rightarrow\int{\dfrac{5x-2}{1+2x+3{{x}^{2}}}}dx=\int{\dfrac{\dfrac{5}{6}(2+6x)-\dfrac{11}{3}}{1+2x+3{{x}^{2}}}}dx$
$=\dfrac{5}{6}\int{\dfrac{2+6x}{1+2x+3{{x}^{2}}}}dx-\dfrac{11}{3}\int{\dfrac{1}{1+2x+3{{x}^{2}}}}dx$
Consider ${{I}_{1}}=\int{\dfrac{2+6x}{1+2x+3{{x}^{2}}}}dx$ and ${{I}_{2}}=\int{\dfrac{1}{1+2x+3{{x}^{2}}}}dx$
$\therefore\int{\dfrac{5x-2}{1+2x+3{{x}^{2}}}}dx=\dfrac{5}{6}{{I}_{1}}-\dfrac{11}{3}{{I}_{2}}...\left( 1 \right)$
${{I}_{1}}=\int{\dfrac{2+6x}{1+2x+3{{x}^{2}}}}dx$
Put $1+2x+3{{x}^{2}}=t$
$\Rightarrow (2+6x)dx=dt$
$\therefore {{I}_{1}}=\int{\dfrac{dt}{t}}$
Using the logarithm formula of integration,
${{I}_{1}}=\log |t|\text{ }$
Substitute the value of t,
${{I}_{1}}=\log \left| 1+2x+3{{x}^{2}} \right|...\left( 2 \right)$
Then,
${{I}_{2}}=\int{\dfrac{1}{1+2x+3{{x}^{2}}}}dx$
$1+2x+3{{x}^{2}}$ can be rewritten as $1+3\left( {{x}^{2}}+\dfrac{2}{3}x \right)$
Thus,
$1+3\left( {{x}^{2}}+\dfrac{2}{3}x \right)$
By completing square method,
$=1+3\left( {{x}^{2}}+\dfrac{2}{3}x+\dfrac{1}{9}-\dfrac{1}{9} \right)$
$=1+3{{\left( x+\dfrac{1}{3} \right)}^{2}}-\dfrac{1}{3}$
Simplifying,
$=\dfrac{2}{3}+3{{\left( x+\dfrac{1}{3} \right)}^{2}}$
$=3\left[ {{\left( x+\dfrac{1}{3} \right)}^{2}}+\dfrac{2}{9} \right]$
$=3\left[ {{\left( x+\dfrac{1}{3} \right)}^{2}}+{{\left( \dfrac{\sqrt{2}}{3} \right)}^{2}} \right]$
Therefore ${{I}_{2}}$can be rewritten as ,
${{I}_{2}}=\dfrac{1}{3}\int{\dfrac{1}{\left. {{\left[ x+\dfrac{1}{3} \right)}^{2}}+{{\left( \dfrac{\sqrt{2}}{3} \right)}^{2}} \right]}}dx$
$=\dfrac{1}{3}\left[ \dfrac{3}{\sqrt{2}}{{\tan }^{-1}}\left( \dfrac{3x+1}{\sqrt{2}} \right) \right]$
Simplifying,
$=\dfrac{1}{\sqrt{2}}{{\tan }^{-1}}\left( \dfrac{3x+1}{\sqrt{2}} \right)...\left( 3 \right)$
We obtain the below values by substituting equations (2) and (3) in equation (1)
$\int{\dfrac{5x-2}{1+2x+3{{x}^{2}}}}dx=\dfrac{5}{6}\left[ \log \left| 1+2x+3{{x}^{2}} \right| \right]-\dfrac{11}{3}\left[ \dfrac{1}{\sqrt{2}}{{\tan }^{-1}}\left( \dfrac{3x+1}{\sqrt{2}} \right) \right]+C$
Simplifying,
$=\dfrac{5}{6}\log \left| 1+2x+3{{x}^{2}} \right|-\dfrac{11}{3\sqrt{2}}{{\tan }^{-1}}\left( \dfrac{3x+1}{\sqrt{2}} \right)+C$
19. $\dfrac{6x+7}{\sqrt{\left( x-5 \right)\left( x-4 \right)}}$
Ans: Consider $6x+7=A\dfrac{d}{dx}\left( {{x}^{2}}-9x+20 \right)+B$
Differentiating,
$\Rightarrow 6\text{ }x+7=A\left( 2\text{ }x-9 \right)+B$
We obtain the below values by equating the coefficients of x and the constant term on both sides.
$2~\text{A}=6\Rightarrow \text{A}=3$
$-9~\text{A}+\text{B}=7\Rightarrow \text{B}=34$
$\therefore 6\text{x}+7=3(2\text{x}-9)+34$
$\int{\dfrac{6x+7}{\sqrt{{{x}^{2}}-9x+20}}}=\int{\dfrac{3(2x-9)+34}{\sqrt{{{x}^{2}}-9x+20}}}dx$
$=3\int{\dfrac{2x-9}{\sqrt{{{x}^{2}}-9x+20}}}dx+34\int{\dfrac{1}{\sqrt{{{x}^{2}}-9x+20}}}dx$
Consider ${{I}_{1}}=\int{\dfrac{2x-9}{\sqrt{{{x}^{2}}-9x+20}}}dx\,\,\,and\,\,{{I}_{2}}=\int{\dfrac{1}{\sqrt{{{x}^{2}}-9x+20}}}dx$
$\therefore\int{\dfrac{6x+7}{\sqrt{{{x}^{2}}-9x+20}}}=3{{I}_{1}}+34{{I}_{2}}\,\,\,\,\,\,\,\,....(1)$
${{I}_{1}}=\int{\dfrac{2x-9}{\sqrt{{{x}^{2}}-9x+20}}}dx$
Put ${{x}^{2}}-9x+20=t$
$\Rightarrow (2x-9)dx=dt$
$\Rightarrow {{I}_{1}}=\int{\dfrac{dt}{\sqrt{t}}}$
Integrating using the power rule
${{I}_{1}}=2\sqrt{t}$
Substitute the value of t,
${{I}_{1}}=2\sqrt{{{x}^{2}}-9x+20}\,\,\,.....(2)$
${{I}_{2}}=\int{\dfrac{1}{\sqrt{{{x}^{2}}-9x+20}}}dx$
Consider
${{x}^{2}}-9x+20$
By completing square methods,
$={{x}^{2}}-9x+20+\dfrac{81}{4}-\dfrac{81}{4}$
$={{\left( x-\dfrac{9}{2} \right)}^{2}}-\dfrac{1}{4}$
$={{\left( x-\dfrac{9}{2} \right)}^{2}}-{{\left( \dfrac{1}{2} \right)}^{2}}$
$\Rightarrow {{I}_{2}}=\int{\dfrac{1}{{{\left( x-\dfrac{9}{2} \right)}^{2}}-{{\left( \dfrac{1}{2} \right)}^{2}}}}\,\,dx$
${{I}_{2}}=\log \left| \left( x-\dfrac{9}{2} \right)+\sqrt{{{x}^{2}}-9x+20} \right|.....\left( 3 \right)$
We obtain the below values by substituting equations (2) and (3) in (1), $\int{\dfrac{6x+7}{\sqrt{{{x}^{2}}-9x+20}}}dx=3\left[ 2\sqrt{{{x}^{2}}-9x+20} \right]+34\log \left[ \left( x-\dfrac{9}{2} \right)+\sqrt{{{x}^{2}}-9x+20} \right]+\text{C}$
Simplifying,
$=6\sqrt{{{x}^{2}}-9x+20}+34\log \left[ \left( x-\dfrac{9}{2} \right)+\sqrt{{{x}^{2}}-9x+20} \right]+C$
20. $\dfrac{x+2}{\sqrt{4x-{{x}^{2}}}}$
Ans:Consider, $\text{x}+2=A\dfrac{d}{dx}\left( 4x-{{x}^{2}} \right)+B$
$\Rightarrow x+2=A(4-2x)+B$
We obtain the below values by equating the coefficients of x and the
constant term on both sides.
$-2A=1\Rightarrow A=-\dfrac{1}{2}$
$4A+B=2\Rightarrow B=4$
$\Rightarrow (x+2)=-\dfrac{1}{2}(4-2x)+4$
$\therefore\int{\dfrac{x+2}{\sqrt{4x-{{x}^{2}}}}}dx=\int{\dfrac{-\dfrac{1}{2}(4-2x)+4}{\sqrt{4x-{{x}^{2}}}}}dx$ $=-\dfrac{1}{2}\int{\dfrac{4-2x}{\sqrt{4x-{{x}^{2}}}}}dx+4\int{\dfrac{1}{\sqrt{4x-{{x}^{2}}}}}dx$
Let ${{I}_{1}}=\int{\dfrac{4-2x}{\sqrt{4x-{{x}^{2}}}}}dx$ and ${{I}_{2}}\int{\dfrac{1}{\sqrt{4x-{{x}^{2}}}}}dx$
$\therefore \int{\dfrac{x+2}{\sqrt{4x-{{x}^{2}}}}}dx=-\dfrac{1}{2}{{I}_{1}}\text{ and }+4{{I}_{2}}\,\,\,\,\,\,\,....\left( 1 \right)$
Then, ${{I}_{1}}=\int{\dfrac{4-2x}{\sqrt{4x-{{x}^{2}}}}}dx$
Let $4x-{{x}^{2}}=t$
$\Rightarrow (4-2x)dx=dt$
$\Rightarrow{{I}_{1}}=\int{\dfrac{dt}{\sqrt{t}}}=2\sqrt{t}=2\sqrt{4x-{{x}^{2}}}...\left( 2 \right)$
(Using the logarithm formula of integration,)
${{I}_{2}}=\int{\dfrac{1}{\sqrt{4x-{{x}^{2}}}}}dx$
Integrating using the power rule,
$\Rightarrow 4x-{{x}^{2}}=-\left( -4x+{{x}^{2}} \right)$
By completing square methods,
$=\left( -4x+{{x}^{2}}+4-4 \right)$
$=4-{{(x-2)}^{2}}$
$={{(2)}^{2}}-{{(x-2)}^{2}}$
$\therefore {{I}_{2}}=\int{\dfrac{1}{\sqrt{{{(2)}^{2}}-{{(x-2)}^{2}}}}}dx={{\sin }^{-1}}\left( \dfrac{x-2}{2} \right)...\left( 3 \right)$
Using equations (2) and (3) in (1), to obtain
$\int{\dfrac{x+2}{\sqrt{4x-{{x}^{2}}}}}dx=-\dfrac{1}{2}\left( 2\sqrt{4x-{{x}^{2}}} \right)+4{{\sin }^{-1}}\left( \dfrac{x-2}{2} \right)+C$
$=-\sqrt{4x-{{x}^{2}}}+4{{\sin }^{-1}}\left( \dfrac{x-2}{2} \right)+C$
21.$\dfrac{x+2}{\sqrt{{{x}^{2}}+2x+3}}$
Ans:$\int{\dfrac{x+2}{\sqrt{{{x}^{2}}+2x+3}}}dx=\dfrac{1}{2}\int{\dfrac{2(x+2)}{\sqrt{{{x}^{2}}+2x+3}}}dx$
Simplifying,
$=\dfrac{1}{2}\int{\dfrac{2x+4}{\sqrt{{{x}^{2}}+2x+3}}}dx$
$=\dfrac{1}{2}\int{\dfrac{2x+2}{\sqrt{{{x}^{2}}+2x+3}}}dx+\dfrac{1}{2}\int{\dfrac{2}{\sqrt{{{x}^{2}}+2x+3}}}dx$
$=\dfrac{1}{2}\int{\dfrac{2x+2}{\sqrt{{{x}^{2}}+2x+3}}}dx+\int{\dfrac{1}{\sqrt{{{x}^{2}}+2x+3}}}dx$
Let ${{I}_{1}}=\int{\dfrac{2x+2}{\sqrt{{{x}^{2}}+2x+3}}}dx\,\,\,and\,\,{{I}_{2}}=\int{\dfrac{1}{\sqrt{{{x}^{2}}+2x+3}}}dx$
$\therefore \int{\dfrac{x+2}{\sqrt{{{x}^{2}}+2x+3}}}dx=\dfrac{1}{2}{{I}_{1}}+{{I}_{2}}\,\,\,\,\,\,\,\,....\left( 1 \right)$
Then, ${{I}_{1}}=\int{\dfrac{2x+2}{\sqrt{{{x}^{2}}+2x+3}}}dx$
Put, ${{x}^{2}}+2x+3=t$
Integrating using the power rule,
$\Rightarrow (2\text{x}+2)\text{dx}=\text{dt}\,\,\,\,\,\,$
$\,{{I}_{1}}=\int{\dfrac{dt}{\sqrt{t}}}=2\sqrt{t}=2\sqrt{{{x}^{2}}+2x+3}..\left( 2 \right)$
${{I}_{2}}=\int{\dfrac{1}{\sqrt{{{x}^{2}}+2x+3}}}dx.$
By completing square methods,
$\Rightarrow {{x}^{2}}+2x+3={{x}^{2}}+2x+1+2={{(x+1)}^{2}}+{{(\sqrt{2})}^{2}}$
$\therefore {{I}_{2}}=\int{\dfrac{1}{\sqrt{{{(x+1)}^{2}}+{{(\sqrt{2})}^{2}}}}}dx=\log \left| (x+1)+\sqrt{{{x}^{2}}+2x+3} \right|...\left( 3 \right)$
Using equations (2) and (3) in (1), to obtain
$\therefore\int{\dfrac{x+2}{\sqrt{{{x}^{2}}+2x+3}}}dx=\sqrt{{{x}^{2}}+2x+3}+\log \left| (x+1)+\sqrt{{{x}^{2}}+2x+3} \right|\,+C$
22. $\dfrac{x+3}{{{x}^{2}}-2x-5}$
Ans: Consider $(x+3)=A\dfrac{d}{dx}\left( {{x}^{2}}-2x-5 \right)+B$
$=\dfrac{{{x}^{2}}}{2}{{(\log x)}^{2}}-\int{x}\log xdx$
We obtain the below values by equating the coefficients of x and the constant term on both sides.
$2A=1\Rightarrow A=\dfrac{1}{2}$
$-2A+B=3\Rightarrow B=4$
$\therefore (x+3)=\dfrac{1}{2}(2x-2)+4$
$\Rightarrow \int{\dfrac{x+3}{{{x}^{2}}-2x-5}}dx=\int{\dfrac{\dfrac{1}{2}(2x-2)+4}{{{x}^{2}}-2x-5}}dx$
$=\dfrac{1}{2}\int{\dfrac{2x-2}{{{x}^{2}}-2x-5}}dx+4\int{\dfrac{1}{{{x}^{2}}-2x-5}}~\text{d}x$
Consider${{I}_{1}}=\int{\dfrac{2x-2}{{{x}^{2}}-2x-5}}dx$ and ${{I}_{2}}=\int{\dfrac{1}{{{x}^{2}}-2x-5}}dx$
$\therefore \int{\dfrac{x+3}{{{x}^{2}}-2x-5}}dx=\dfrac{1}{2}{{I}_{1}}+4{{I}_{2}}...\left( 1 \right)$
Then, ${{I}_{1}}=\int{\dfrac{2x-2}{{{x}^{2}}-2x-5}}dx$
Put ${{x}^{2}}-2x-5=\text{t}$
$\Rightarrow (2x-2)dx=dt$
Using the logarithm formula of integration,
$\Rightarrow {{I}_{1}}=\int{\dfrac{dt}{t}}=\log |t|=\log \left| {{x}^{2}}-2x-5 \right|\,\,\,....\left( 2 \right)$
${{I}_{2}}=\int{\dfrac{1}{{{x}^{2}}-2x-5}}dx$
$=\int{\dfrac{1}{\left( {{x}^{2}}-2x+1 \right)-6}}dx$
$=\int{\dfrac{1}{{{(x-1)}^{2}}+{{(\sqrt{6})}^{2}}}}dx$
$=\dfrac{1}{2\sqrt{6}}\log \left( \dfrac{x-1-\sqrt{6}}{x-1+\sqrt{6}} \right)....\left( 3 \right)$
We obtain the below values by substituting (2) and (3) in (1),
$\int{\dfrac{x+3}{{{x}^{2}}-2x-5}}dx=\dfrac{1}{2}\log \left| {{x}^{2}}-2x-5 \right|+\dfrac{4}{2\sqrt{6}}\log \left| \dfrac{x-1-\sqrt{6}}{x-1+\sqrt{6}} \right|+C$
$=\dfrac{1}{2}\log \left| {{x}^{2}}-2x-5 \right|+\dfrac{2}{\sqrt{6}}\log \left| \dfrac{x-1-\sqrt{6}}{x-1+\sqrt{6}} \right|+C$
23. $\dfrac{5x+3}{\sqrt{{{x}^{2}}+4x+10}}$
Ans: $5x+3=A\dfrac{a}{dx}\left( {{x}^{2}}+4x+10 \right)+B$
$\Rightarrow 5x+3=A(2x+4)+B$
Equating the coefficients of $\text{x}$and constant term, we get
$2A=5\Rightarrow A=\dfrac{5}{2}$
$4A+B=3\Rightarrow B=-7$
$\therefore 5x+3=\dfrac{5}{2}(2x+4)-7$
$\Rightarrow \int{\dfrac{5x+3}{\sqrt{{{x}^{2}}+4x+10}}}dx=\int{\dfrac{\dfrac{5}{2}(2x+4)-7}{\sqrt{{{x}^{2}}+4x+10}}}dx$
$=\dfrac{5}{2}\int{\dfrac{2x+4}{\sqrt{{{x}^{2}}+4x+10}}}dx-7\int{\dfrac{1}{\sqrt{{{x}^{2}}+4x+10}}}dx$
Let ${{I}_{1}}=\int{\dfrac{2x+4}{\sqrt{{{x}^{2}}+4x+10}}}dx$ and ${{I}_{2}}=\int{\dfrac{1}{\sqrt{{{x}^{2}}+4x+10}}}dx$
$\therefore \int{\dfrac{5x+3}{\sqrt{{{x}^{2}}+4x+10}}}dx=\dfrac{5}{2}{{I}_{1}}-7{{I}_{2}}\,\,\,\,\,\,\,\,\,....\left( 1 \right)$
Then, ${{I}_{1}}=\int{\dfrac{2x+4}{\sqrt{{{x}^{2}}+4x+10}}}dx$
Consider ${{x}^{2}}+4x+10=\text{t}\therefore (2x+4)dx=dt$
$\Rightarrow {{I}_{1}}=\int{\dfrac{dt}{t}}=2\sqrt{t}=2\sqrt{{{x}^{2}}+4x+10}\,\,\,\,\,\,\,......\left( 2 \right)$
${{I}_{2}}=\int{\dfrac{1}{\sqrt{{{x}^{2}}+4x+10}}}dx$
$=\int{\dfrac{1}{\sqrt{\left( {{x}^{2}}+4x+4 \right)+6}}}dx=\int{\dfrac{1}{{{(x+2)}^{2}}+{{(\sqrt{6})}^{2}}}}dx$
$=\log \left| (x+2)\sqrt{{{x}^{2}}+4x+10} \right|...\left( 3 \right)$
We obtain the below values by using equations (2) and (3) in (1). $\int{\dfrac{5x+3}{\sqrt{{{x}^{2}}+4x+10}}}dx=\dfrac{5}{2}\left[ 2\sqrt{{{x}^{2}}+4x+10} \right]-7\log \left| (x+2)\sqrt{{{x}^{2}}+4x+10} \right|+C$$=5\sqrt{{{x}^{2}}+4x+10}-7\log \left| (x+2)\sqrt{{{x}^{2}}+4x+10} \right|+C$
24. $\int{\dfrac{dx}{{{x}^{2}}+2x+2}}$equals
$x{{\tan }^{-1}}\left( x+1 \right)+C$
${{\tan }^{-1}}\left( x+1 \right)+C$
$\left( x+1 \right){{\tan }^{-1}}x+C$
${{\tan }^{-1}}x+C$
Ans: $\int{\dfrac{dx}{{{x}^{2}}+2x+2}}=\int{\dfrac{dx}{\left( {{x}^{2}}+2x+1 \right)+1}}$
$=\int{\dfrac{1}{{{(x+1)}^{2}}+{{(1)}^{2}}}}dx$
$=\left[ {{\tan }^{-1}}(x+1) \right]+C$
Hence, the right response is is B.
25. $\int{\dfrac{dx}{\sqrt{9x-4{{x}^{2}}}}}$ equals
$\dfrac{1}{9}{{\sin }^{-1}}\left( \dfrac{9x-8}{8} \right)+C$
$\dfrac{1}{2}{{\sin }^{-1}}\left( \dfrac{8x-9}{9} \right)+C$
$\dfrac{1}{3}{{\sin }^{-1}}\left( \dfrac{9x-8}{8} \right)+C$
$\dfrac{1}{2}{{\sin }^{-1}}\left( \dfrac{9x-8}{9} \right)+C$
Ans:
$\int{\dfrac{dx}{\sqrt{9x-4{{x}^{2}}}}}$
$=\int{\dfrac{1}{\sqrt{-4\left( {{x}^{2}}-\dfrac{9}{4}x \right)}}}dx$
By completing square methods,
$=\int{\dfrac{1}{-4\left( {{x}^{2}}-\dfrac{9}{4}x+\dfrac{81}{64}-\dfrac{81}{64} \right)}}dx$
$=\int{\dfrac{1}{\sqrt{-4\left[ {{\left( x-\dfrac{9}{8} \right)}^{2}}-{{\left( \dfrac{9}{8} \right)}^{2}} \right]}}}dx$
$=\dfrac{1}{2}\int{\dfrac{1}{{{\left( \dfrac{9}{8} \right)}^{2}}-{{\left( x-\dfrac{9}{8} \right)}^{2}}}}dx$
$=\dfrac{1}{2}\left[ {{\sin }^{-1}}\left( \dfrac{x-\dfrac{9}{8}}{\dfrac{9}{8}} \right) \right]+C\quad \left( \int{\dfrac{dy}{\sqrt{{{a}^{2}}-{{y}^{2}}}}}={{\sin }^{-1}}\dfrac{y}{a}+C \right)$
Simplifying,
$=\dfrac{1}{2}{{\sin }^{-1}}\left( \dfrac{8x-9}{9} \right)+C$
Hence, the right response is B.
Exercise 7.5
1. $\dfrac{x}{\left( x+1 \right)\left( x+2 \right)}$
Ans: Let $\dfrac{x}{(x+1)(x+2)}=\dfrac{A}{(x+1)}+\dfrac{B}{(x+2)}$
$\Rightarrow x=A(x+2)+B(x+1)$
We obtain the below values by equating the coefficients of x and the constant term on both sides.
$\text{A}+\text{B}=1$
$2~\text{A}+\text{B}=0$
On solving, we get
$\text{A}=-1\text{ and B}=2$
$\therefore \dfrac{x}{(x+1)(x+2)}=\dfrac{-1}{(x+1)}+\dfrac{2}{(x+2)}$
$\Rightarrow \int{\dfrac{x}{(x+1)(x+2)}}dx=\int{\dfrac{-1}{(x+1)}}+\dfrac{2}{(x+2)}dx$
Using the logarithm formula of integration,
$=-\log |x+1|+2\log |x+2|+C$
$=\log {{(x+2)}^{2}}-\log |x+1|+C$
Simplifying,
$=\log \dfrac{{{(x+2)}^{2}}}{(x+1)}+C$
2. $\dfrac{1}{{{x}^{2}}-9}$
Ans: Let $\dfrac{1}{(x+3)(x-3)}=\dfrac{A}{(x+3)}+\dfrac{B}{(x-3)}$
$1=A\left( x-3 \right)+B\left( x+3 \right)$
Equating the coefficients of $\text{x}$and constant term, we get
$A+B=0$
$1=-3A+3B$
$A=-\dfrac{1}{6}\text{ and }B=\dfrac{1}{6}$
$\therefore \dfrac{1}{(x+3)(x-3)}=\dfrac{-1}{6(x+3)}+\dfrac{1}{6(x-3)}$
$\Rightarrow \int{\dfrac{1}{\left( {{x}^{2}}-9 \right)}}dx=\int{\left( \dfrac{-1}{6(x+3)}+\dfrac{1}{6(x-3)} \right)}dx$
Using the logarithm formula of integration,
$=-\dfrac{1}{6}\log |x+3|+\dfrac{1}{6}\log |x-3|+C=\dfrac{1}{6}\log \dfrac{|(x-3)|}{|(x+3)|}+C$
3. $\dfrac{3x-1}{\left( x-1 \right)\left( x-2 \right)\left( x-3 \right)}$
Ans: Let $\dfrac{3x-1}{(x-1)(x-2)(x-3)}=\dfrac{A}{(x-1)}+\dfrac{B}{(x-2)}+\dfrac{C}{(x-3)}$
$3x-1=A(x-2)(x-3)+B(x-1)(x-3)+C(x-1)(x-2)\quad \ldots \left( 1 \right)$
We obtain the below values by equating the coefficients of x , ${{x}^{2}}$ and the constant term on both sides.
$\text{A}+\text{B}+\text{C}=0$
$-5~\text{A}-4~\text{B}-3\text{C}=3$
$6~\text{A}+3~\text{B}+2\text{C}=-1$
Solving these equations, to obtain
$\text{A}=1,~\text{B}=-5,\text{ and C}=4$
$\therefore \dfrac{3x-1}{(x-1)(x-2)(x-3)}=\dfrac{1}{(x-1)}-\dfrac{5}{(x-2)}+\dfrac{4}{(x-3)}$
$\Rightarrow \int{\dfrac{3x-1}{(x-1)(x-2)(x-3)}}dx=\int{\left\{ \dfrac{1}{(x-1)}-\dfrac{5}{(x-2)}+\dfrac{4}{(x-3)} \right\}}dx$
Using the logarithm formula of integration,
$=\log |x-1|-5\log |x-2|+4\log |x-3|+C$
4. $\dfrac{x}{\left( x-1 \right)\left( x-2 \right)\left( x-3 \right)}$
Ans:Let $\dfrac{x}{(x-1)(x-2)(x-3)}=\dfrac{A}{(x-1)}+\dfrac{B}{(x-2)}+\dfrac{C}{(x-3)}$
$x=A\left( x-2 \right)\left( x-3 \right)+B\left( x-1 \right)\left( x-3 \right)+C\left( x-1 \right)\left( x-2 \right)$
We obtain the below values by equating the coefficients of x , ${{x}^{2}}$ and the constant term on both sides.
$A+B+C=0\text{ }$
$-5\text{ }A-4\text{ }B-3\text{ }C=1$
$6\text{ }A+4\text{ }B+2\text{ }C=0$
Solving these equations, to obtain
$A=\dfrac{1}{2},B=2\text{ and }C=\dfrac{3}{2}$
$\therefore \dfrac{x}{(x-1)(x-2)(x-3)}=\dfrac{1}{2(x-1)}-\dfrac{2}{(x-2)}+\dfrac{3}{2(x-3)}$
$\Rightarrow \int{\dfrac{x}{(x-1)(x-2)(x-3)}}dx=\int{\left\{ \dfrac{1}{2(x-1)}-\dfrac{2}{(x-2)}+\dfrac{3}{2(x-3)} \right\}}dx$
Using the logarithm formula of integration,
$=\dfrac{1}{2}\log |x-1|-2\log |x-2|+\dfrac{3}{2}\log |x-3|+C$
5. $\dfrac{2x}{{{x}^{2}}+3x+2}$
Ans:$\dfrac{2x}{{{x}^{2}}+3x+2}=\dfrac{A}{(x+1)}+\dfrac{B}{(x+2)}$
$2\text{ }x=A\left( x+2 \right)+B\left( x+1 \right)$
We obtain the below values by equating the coefficients of x , ${{x}^{2}}$ and the constant term on both sides.
$A+B=2$
$2\text{ }A+B=0$
Solving these equations, we get
$A=-2\text{ and }B=4$
$\therefore \dfrac{2x}{(x+1)(x+2)}=\dfrac{-2}{(x+1)}+\dfrac{4}{(x+2)}$
$\Rightarrow \int{\dfrac{2x}{(x+1)(x+2)}}dx=\int{\left\{ \dfrac{4}{(x+2)}-\dfrac{2}{(x+1)} \right\}}dx$
Using the logarithm formula of integration,
$=4\log |x+2|-2\log |x+1|+C$
6. $\dfrac{1-{{x}^{2}}}{x\left( 1-2x \right)}$
Ans: It can be seen that the given integrand is not a proper fraction.
Therefore, on dividing $\left( 1-{{x}^{2}} \right)$ by $x(1-2x)$ to obtain, $\dfrac{1-{{x}^{2}}}{x(1+2x)}=\dfrac{1}{2}+\dfrac{1}{2}\left( \dfrac{2-x}{x(1-2x)} \right)$
Let $\dfrac{2-x}{x(1-2x)}=\dfrac{A}{x}+\dfrac{B}{(1-2x)}...\left( 1 \right)$
$\Rightarrow (2-x)=A(1-2x)+Bx$
We obtain the below values by equating the coefficients of x , ${{x}^{2}}$ and the constant term on both sides.
$-2~\text{A}+\text{B}=-1$ and, $A=2$
Solving these equations, to obtain $A=2\text{ and }B=3$
$\therefore \dfrac{2-x}{x(1-2x)}=\dfrac{2}{x}+\dfrac{3}{1-2x}$
Substituting in equation (1), we get
$\dfrac{1-{{x}^{2}}}{x(1+2)}=\dfrac{1}{2}+\dfrac{1}{2}\left\{ \dfrac{2}{x}+\dfrac{3}{(1-2x)} \right\}$
$\Rightarrow \int{\dfrac{1-{{x}^{2}}}{x(1+2)}}dx=\int \dfrac{1}{2}+\dfrac{1}{2}\text{(}\dfrac{2}{x}+\dfrac{3}{(1-2x)})dx$
Using the power rule and logarithm formula of integration,
$=\dfrac{x}{2}+\log |x|+\dfrac{3}{2(-2)}\log |1-2x|+C=\dfrac{x}{2}+\log |x|-\dfrac{3}{4}\log |1-2x|+C$
7. $\dfrac{x}{\left( {{x}^{2}}+1 \right)\left( x-1 \right)}$
Ans: Let $\dfrac{x}{\left( {{x}^{2}}+1 \right)(x-1)}=\dfrac{Ax+B}{\left( {{x}^{2}}+1 \right)}+\dfrac{C}{(x-1)}...\left( 1 \right)$
$x=(Ax+B)(x-1)+C\left( {{x}^{2}}+1 \right)$
$x=A{{x}^{2}}-Ax+Bx-B+C{{x}^{2}}+C$
We obtain the below values by equating the coefficients of x , ${{x}^{2}}$ and the constant term on both sides.
$A+C=0\text{ }$
$-A+B=1$
$-B+C=0$
On solving these equations, to obtain $A=-\dfrac{1}{2},B=\dfrac{1}{2},and\,\,C=\dfrac{1}{2}$
From equation (1), to obtain
$\therefore \dfrac{x}{\left( {{x}^{2}}+1 \right)(x-1)}=\dfrac{\left( -\dfrac{1}{2}x+\dfrac{1}{2} \right)}{{{x}^{2}}+1}+\dfrac{\dfrac{1}{2}}{(x-1)}$
$\Rightarrow \int{\dfrac{x}{\left( {{x}^{2}}+1 \right)(x-1)}}=-\dfrac{1}{2}\int{\dfrac{x}{{{x}^{2}}+1}}dx+\dfrac{1}{2}\int{\dfrac{1}{{{x}^{2}}+1}}dx+\dfrac{1}{2}\int{\dfrac{1}{x-1}}dx$
$=-\dfrac{1}{4}\int{\dfrac{2x}{{{x}^{2}}+1}}dx+\dfrac{1}{2}{{\tan }^{-1}}x+\dfrac{1}{2}\log |x-1|+C$
Consider $\int{\dfrac{2x}{{{x}^{2}}+1}}dx,lel\left( {{x}^{2}}+1 \right)=t\Rightarrow 2xdx=dt$
$\Rightarrow \int{\dfrac{2x}{{{x}^{2}}+1}}dx=\int{\dfrac{dt}{t}}=\log |t|=\log \left| {{x}^{2}}+1 \right|$
$\therefore \int{\dfrac{x}{\left( {{x}^{2}}+1 \right)(x-1)}}=-\dfrac{1}{4}\log \left| {{x}^{2}}+1 \right|+\dfrac{1}{2}{{\tan }^{-1}}x+\dfrac{1}{2}\log |x-1|+C$
$=\dfrac{1}{2}\log |x-1|-\dfrac{1}{4}\log \left| {{x}^{2}}+1 \right|+\dfrac{1}{2}{{\tan }^{-1}}x+C$
8. .$\dfrac{x}{{{\left( x-1 \right)}^{2}}\left( x+2 \right)}$
Ans: $\dfrac{x}{{{(x-1)}^{2}}(x+2)}$
$\text{ Let }\dfrac{x}{{{(x-1)}^{2}}(x+2)}=\dfrac{A}{(x-1)}+\dfrac{B}{{{(x-1)}^{2}}}+\dfrac{C}{(x+2)}$
$x=A(x-1)(x+2)+B(x+2)+C{{(x-1)}^{2}}$
We obtain the below values by equating the coefficients of x , ${{x}^{2}}$ and the constant term on both sides.
$A+C=0$
$A+B-2\text{ }C=1$
On solving, to obtain
$A=\dfrac{2}{9}\text{ and }C=\dfrac{-2}{9}$
$B=\dfrac{1}{3}$
$\therefore \dfrac{x}{{{(x-1)}^{2}}(x+2)}=\dfrac{2}{9(x-1)}+\dfrac{1}{3{{(x-1)}^{2}}}-\dfrac{2}{9(x\mid 2)}$
$\Rightarrow \int{\dfrac{x}{{{(x-1)}^{2}}(x+2)}}dx=\dfrac{2}{9}\int{\dfrac{1}{(x-1)}}dx+\dfrac{1}{3}\int{\dfrac{1}{{{(x-1)}^{2}}}}dx-\dfrac{2}{9}\int{\dfrac{1}{(x-2)}}dx$
Using the power rule and logarithm formula of integration,
$=\dfrac{2}{9}\log |x-1|+\dfrac{1}{3}\left( \dfrac{-1}{x-1} \right)-\dfrac{2}{9}\log |x+2|+C$
Simplifying,
$=\dfrac{2}{9}\log \left| \dfrac{x-1}{x+2} \right|-\dfrac{1}{3(x-1)}+C$
9. $\dfrac{3x+5}{{{x}^{3}}-{{x}^{2}}-x+1}$
Ans: $\dfrac{3x+5}{{{x}^{3}}-{{x}^{2}}-x+1}=\dfrac{3x+5}{{{(x-1)}^{2}}(x+1)}$
let $\dfrac{3x+5}{{{(x-1)}^{2}}(x+1)}=\dfrac{A}{(x+1)}+\dfrac{B}{{{(x-1)}^{2}}}+\dfrac{C}{(x+1)}$
$3x+5=A(x-1)(x+1)+B(x+1)+{{(x-1)}^{2}}$
$3x+5=A{{(x-1)}^{2}}+B(x+1)+C\left( {{x}^{2}}+1-2x \right)\quad \ldots (1)$
We obtain the below values by equating the coefficients of x , ${{x}^{2}}$ and
the constant term on both sides.
$A+C=0\text{ }$
$B-2\text{ }C=3\text{ }$
$-A+B+C=5$
On solving, to obtain $B=4\,\,A=-\dfrac{1}{2}\,\,and\,\,C=\dfrac{1}{2}$
$\therefore \dfrac{3x+5}{{{(x-1)}^{2}}(x+1)}=\dfrac{-1}{2(x-1)}+\dfrac{4}{{{(x-1)}^{2}}}+\dfrac{1}{2(x+1)}$
$\Rightarrow \int{\dfrac{3x+5}{{{(x-1)}^{2}}(x+1)}}dx=-\dfrac{1}{2}\int{\dfrac{1}{x-1}}dx+4\int{\dfrac{1}{{{(x-1)}^{2}}}}dx+\dfrac{1}{2}\int{\dfrac{1}{(x+1)}}dx$
Using the power rule and logarithm formula of integration,
$=-\dfrac{1}{2}\log |x-1|+4\left( \dfrac{-1}{x-1} \right)+\dfrac{1}{2}\log |x+1|+C$
$=\dfrac{1}{2}\log \left| \dfrac{x+1}{x-1} \right|-\dfrac{4}{(x-1)}+C$
10. $\dfrac{2x-3}{\left( {{x}^{2}}-1 \right)\left( 2x+3 \right)}$
Ans: $\dfrac{2x-3}{\left( {{x}^{2}}-1 \right)(2x+3)}=\dfrac{2x-3}{(x+1)(x-1)(2x+3)}$
Let $\dfrac{2x-3}{(x+1)(x-1)(2x+3)}=\dfrac{A}{(x+1)}+\dfrac{B}{(x-1)}+\dfrac{C}{(2x+3)}$
$\Rightarrow (2x-3)=A(x-1)(2x+3)+B(x+1)(2x+3)+C(x+1)(x-1)$
$\Rightarrow (2x-3)-A\left( 2{{x}^{2}}+x-3 \right)+B\left( 2{{x}^{2}}+5x-3 \right)+C\left( {{x}^{2}}-1 \right)$
$\Rightarrow (2x-3)=(2A+2B+C){{x}^{2}}+(A+5B)x+(-3A+3B-C)$
We obtain the below values by equating the coefficients of x , ${{x}^{2}}$ and the constant term on both sides.
$2~\text{A}+2~\text{B}+\text{C}=0$
$\text{A}+5~\text{B}=2$
$-3~\text{A}+3~\text{B}-\text{C}=-3$
On solving, to obtain $B=-\dfrac{1}{10},A=\dfrac{5}{2},\text{ and C}=-\dfrac{24}{5}$
$\therefore \dfrac{2x-3}{(x+1)(x-1)(2x+3)}=\dfrac{5}{2(x+1)}-\dfrac{1}{10(x-1)}-\dfrac{24}{5(2x+3)}$
$\Rightarrow \int{\dfrac{2x-3}{\left( {{x}^{2}}-1 \right)(2x+3)}}dx=\dfrac{5}{2}\int{\dfrac{1}{(x+1)}}dx-\dfrac{1}{10}\int{\dfrac{1}{x-1}}dx-\dfrac{24}{5}\int{\dfrac{1}{(2x+3)}}dx$
Using the logarithm formula of integration,
$=\dfrac{5}{2}\log |x+1|-\dfrac{1}{10}\log |x-1|-\dfrac{24}{5\times 2}\log |2x+3|$
Simplifying,
$=\dfrac{5}{2}\log |x+1|-\dfrac{1}{10}\log |x-1|-\dfrac{12}{5}\log |2x+3|+C$
11. $\dfrac{5x}{\left( x+1 \right)\left( {{x}^{2}}-4 \right)}$
Ans: $\dfrac{5x}{(x+1)\left( {{x}^{2}}-4 \right)}=\dfrac{5x}{(x+1)(x+2)(x-2)}$
let $\dfrac{5x}{(x+1)(x+2)(x-2)}=\dfrac{A}{(x+1)}+\dfrac{B}{(x+2)}+\dfrac{C}{(x-2)}$
$5\text{ }x=A\left( x+2 \right)\left( x-2 \right)+B\left( x+1 \right)\left( x-2 \right)+C\left( x+1 \right)\left( x+2 \right)$
We obtain the below values by equating the coefficients of x , ${{x}^{2}}$ and the constant term on both sides.
$A+B+C=0$
$-B+3C=5\text{ and, }-4A-2B+2C=0$
On solving, to obtain
$A=\dfrac{5}{3},B=-\dfrac{5}{2},\text{ and }C=\dfrac{5}{6}$
$\therefore \dfrac{5x}{(x+1)(x+2)(x-2)}=\dfrac{5}{3(x+1)}+-\dfrac{5}{2(x+2)}+\dfrac{5}{6(x-2)}$
$\Rightarrow \int{\dfrac{5x}{(x+1)\left( {{x}^{2}}-4 \right)}}dx=\dfrac{5}{3}\int{\dfrac{1}{(x+1)}}dx-\dfrac{5}{2}\int{\dfrac{1}{(x+2)}}dx+\dfrac{5}{6}\int{\dfrac{1}{(x-2)}}dx$
Using the logarithm formula of integration,
$=\dfrac{5}{3}\log |x+1|-\dfrac{5}{2}\log |x+2|+\dfrac{5}{6}\log |x-2|+C$
12. $\dfrac{{{x}^{2}}+x+1}{{{x}^{2}}-1}$
Ans: On dividing $\left( {{x}^{3}}+x+1 \right)\,\,\,by\,\,{{x}^{2}}-1,$we get
$\dfrac{{{x}^{3}}+x+1}{{{x}^{2}}-1}=x+\dfrac{2x+1}{{{x}^{2}}-1}$
$\text{ Let }\dfrac{2x+1}{{{x}^{2}}-1}=\dfrac{A}{(x+1)}+\dfrac{B}{(x-1)}$
$2\text{ }x+1=A\left( x-1 \right)+B\left( x+1 \right)$
We obtain the below values by equating the coefficients of x and the constant term on both sides.
$A+B=2$
$-A+B=1$
On solving, to obtain
$A=\dfrac{1}{2}\text{ and }B=\dfrac{3}{2}\text{ }$
$\therefore \dfrac{{{x}^{3}}+x+1}{{{x}^{2}}-1}=x+\dfrac{1}{2(x+1)}+\dfrac{3}{2(x-1)}\text{ }$
$\Rightarrow \int{\dfrac{{{x}^{3}}+x+1}{{{x}^{2}}+1}}dx=\int{x}dx+\dfrac{1}{2}\int{\dfrac{1}{(x+1)}}dx+\dfrac{3}{2}\int{\dfrac{1}{(x-1)}}dx$
$=\dfrac{{{x}^{2}}}{2}+\log |x+1|+\dfrac{3}{2}\log |x-1|+C$
13. $\dfrac{2}{\left( 1-x \right)\left( 1+{{x}^{2}} \right)}$
Ans: Let $\dfrac{2}{(1-x)\left( 1+{{x}^{2}} \right)}=\dfrac{A}{(1-x)}+\dfrac{Bx+C}{\left( 1+{{x}^{2}} \right)}$
$2=A\left( 1+{{x}^{2}} \right)+(Bx+C)(1-x)$
$2=A+A{{x}^{2}}+Bx-B{{x}^{2}}+C-Cx$
We obtain the below values by equating the coefficients of x ${{x}^{2}}$ and the constant term on both sides.
$\text{A}-\text{B}=0$
$B-C=0$
$A+C=2$
On solving these equations, to obtain
$A=1,B=1\text{, and }C=1$
$\therefore \dfrac{2}{(1-x)\left( 1+{{x}^{2}} \right)}=\dfrac{1}{1-x}+\dfrac{x+1}{1+{{x}^{2}}}$
$\Rightarrow \int{\dfrac{2}{(1-x)\left( 1+{{x}^{2}} \right)}}dx=\int{\dfrac{1}{1-x}}dx+\int{\dfrac{x}{1+{{x}^{2}}}}dx+\int{\dfrac{1}{1+{{x}^{2}}}}dx$
$=-\int{\dfrac{1}{1-x}}dx+\dfrac{1}{2}\int{\dfrac{2x}{1+{{x}^{2}}}}dx+\int{\dfrac{1}{1+{{x}^{2}}}}dx$
$=-\log |x-1|+\dfrac{1}{2}\log \left| 1+{{x}^{2}} \right|+{{\tan }^{-1}}x+C$
14. $\dfrac{3x-1}{{{\left( x+2 \right)}^{2}}}$
Ans: Let $\dfrac{3x-1}{{{(x+2)}^{2}}}=\dfrac{A}{(x+2)}+\dfrac{B}{{{(x+2)}^{2}}}$
$\Rightarrow 3x-1=A(x+2)+B$
We obtain the below values by equating the coefficients of x and the constant term on both sides.
$A=3$
$2A+B=-1\Rightarrow B=-7$
$\therefore \dfrac{3x-1}{{{(x+2)}^{2}}}=\dfrac{3}{(x+2)}-\dfrac{7}{{{(x+2)}^{2}}}$
$\Rightarrow \int{\dfrac{3x-1}{{{(x+2)}^{2}}}}dx=3\int{\dfrac{1}{(x+2)}}dx-7\int{\dfrac{x}{{{(x+2)}^{2}}}}dx$
Using the power rule and logarithm formula of integration
$=3\log |x+2|-7\left( \dfrac{-1}{(x+2)} \right)+C$
$=3\log |x+2|+\dfrac{7}{(x+2)}+C$
15. $\dfrac{1}{{{x}^{4}}-1}$
Ans: $\dfrac{1}{\left( {{x}^{4}}-1 \right)}=\dfrac{1}{\left( {{x}^{2}}-1 \right)\left( {{x}^{2}}+1 \right)}=\dfrac{1}{(x+1)(x-1)\left( 1+{{x}^{2}} \right)}$
Let $\dfrac{1}{(x+1)(x-1)\left( 1+{{x}^{2}} \right)}=\dfrac{A}{(x+1)}+\dfrac{B}{(x-1)}+\dfrac{Cx+D}{\left( {{x}^{2}}+1 \right)}$
$1=A(x-1)\left( 1+{{x}^{2}} \right)+B(x+1)\left( 1+{{x}^{2}} \right)+(Cx+D)\left( {{x}^{2}}-1 \right)$
$1=A\left( {{x}^{3}}+x-{{x}^{2}}-1 \right)+B\left( {{x}^{3}}+x+{{x}^{2}}+1 \right)+C{{x}^{3}}+D{{x}^{2}}-Cx-D$
$1=(A+B+C){{x}^{3}}+(-A+B+D){{x}^{2}}+(A+B-C)x+(-A+B-D)$
We obtain the below values by equating the coefficients of${{\text{x}}^{3}},{{\text{x}}^{2}},\text{x},$and constant term, we get
$A+B+C=0\text{ }$
$-A+B+D=0$
$A+B-C=0\text{ }$
$-A+B-D=1$
$A=-\dfrac{1}{4},B=\dfrac{1}{4},C=0,\text{ and }D=-\dfrac{1}{2}$
$\therefore \dfrac{1}{\left( {{x}^{4}}-1 \right)}=\dfrac{-1}{4(x+1)}+\dfrac{1}{4(x-1)}+\dfrac{1}{2\left( {{x}^{2}}+1 \right)}$
$\Rightarrow \int{\dfrac{1}{{{x}^{4}}-1}}dx=-\dfrac{1}{4}\log |x-1|+\dfrac{1}{4}\log |x-1|-\dfrac{1}{2}{{\tan }^{1}}x+C$
Simplifying,
$=\dfrac{1}{4}\log \left| \dfrac{x-1}{x+1} \right|-\dfrac{1}{2}{{\tan }^{1}}x+C$
16. $\dfrac{1}{x\left( {{x}^{n}}+1 \right)}$ $\text{ [ }$Hint: Multiply numerator and denominator by ${{x}^{n-1}}$ and put ${{x}^{n}}=t$ $\text{]}$
Ans: $\dfrac{1}{x\left( {{x}^{n}}+1 \right)}$
Numerator and denominator are multiplied by ${{x}^{n-1}}$, to obtain
$\dfrac{1}{x\left( {{x}^{n}}+1 \right)}=\dfrac{{{x}^{n-1}}}{{{x}^{n-1}}x\left( {{x}^{n}}+1 \right)}=\dfrac{{{x}^{n-1}}}{{{x}^{n}}\left( {{x}^{n}}+1 \right)}$
Consider ${{x}^{n}}=t\Rightarrow {{x}^{n-1}}dx=dt$
$\therefore \int{\dfrac{1}{x\left( {{x}^{n}}+1 \right)}}dx=\int{\dfrac{{{x}^{n-1}}}{{{x}^{n}}\left( {{x}^{n}}+1 \right)}}dx=\dfrac{1}{n}\int{\dfrac{1}{t(t+1)}}dt$
$\text{ Let }\dfrac{1}{t(t+1)}=\dfrac{A}{t}+\dfrac{B}{(t+1)}$
$1=A\left( 1+t \right)+B\text{ }t$
We obtain the below values by equating the coefficients of $\text{t}$and constant,
$A=1\text{ and }B=-1$
$\therefore \dfrac{1}{t(t+1)}=\dfrac{1}{t}-\dfrac{1}{(1+t)}$
$\Rightarrow \int{\dfrac{1}{x\left( {{x}^{n}}+1 \right)}}dx=\dfrac{1}{n}\int{\left\{ \dfrac{1}{t}-\dfrac{1}{(1+t)} \right\}}dx$
$=\dfrac{1}{n}[\log |t|-\log |t+1|]+C$
Substitute the value of t,
$=-\dfrac{1}{n}\left[ \log \left| {{x}^{n}} \right|-\log \left| {{x}^{n}}+1 \right| \right]+C$
Simplifying,
$=\dfrac{1}{n}\log \left| \dfrac{{{x}^{u}}}{{{x}^{\prime \prime }}+1} \right|+C$
17. $\dfrac{\cos x}{\left( 1-\sin x \right)\left( 2-\sin x \right)}$ $\text{[}$ hint: Put $\sin x=t$ $\text{]}$
Ans: $\dfrac{\cos x}{(1-\sin x)(2-\sin x)}\,\,\,\,Put,\sin x=t\Rightarrow \cos xdx=dt$
$\therefore \int{\dfrac{\cos x}{(1-\sin x)(2-\sin x)}}dx=\int{\dfrac{dt}{(1-t)(2-t)}}$
let $\dfrac{1}{(1-t)(2-t)}=\dfrac{A}{(1-t)}+\dfrac{B}{(2-t)}$
$1=A\left( 2-t \right)+B\left( 1-t \right)$
We obtain the below values by equating the coefficients of t and constant,
$-2~\text{A}-\text{B}=0\,\,,and\,\,2~\text{A}+\text{B}=1$
$A=1\text{ and }B=-1$
$\therefore \dfrac{1}{(1-t)(2-t)}=\dfrac{1}{(1-t)}-\dfrac{1}{(2-t)}$
$\Rightarrow \int{\dfrac{\cos x}{(1-\sin x)(2-\sin x)}}dx=\int{\left\{ \dfrac{1}{1-t}-\dfrac{1}{(2-t)} \right\}}dt=-\log |1-t|+\log |2-t|+C$ Simplifying,
$=\log \left| \dfrac{2-t}{1-t} \right|+C$
Substitute the value of t,
$=\log \left| \dfrac{2-\sin x}{1-\sin x} \right|+C$
18. $\dfrac{\left( {{x}^{2}}+1 \right)\left( {{x}^{2}}+2 \right)}{\left( {{x}^{2}}+3 \right)\left( {{x}^{2}}+4 \right)}$
Ans: $\dfrac{\left( {{x}^{2}}+1 \right)\left( {{x}^{2}}+2 \right)}{\left( {{x}^{2}}+3 \right)\left( {{x}^{2}}+4 \right)}=\dfrac{\left( 4{{x}^{2}}+10 \right)}{\left( {{x}^{2}}+3 \right)\left( {{x}^{2}}+4 \right)}$
Let $\dfrac{\left( 4{{x}^{2}}+10 \right)}{\left( {{x}^{2}}+3 \right)\left( {{x}^{2}}+4 \right)}=\dfrac{Ax+B}{\left( {{x}^{2}}+3 \right)}+\dfrac{Cx+D}{\left( {{x}^{2}}+4 \right)}$
$4{{x}^{2}}+10=(Ax+B)\left( {{x}^{2}}+4 \right)+(Cx+D)\left( {{x}^{2}}+3 \right)$
$4{{x}^{2}}+10=A{{x}^{2}}+4Ax+B{{x}^{2}}+4B+C{{x}^{3}}+3Cx+D{{x}^{2}}+3D$
$4{{x}^{2}}+10=(A+C){{x}^{3}}+(B+D){{x}^{2}}+(4A+3C)x+(4B+3D)$
We obtain the below values by equating the coefficients of ${{\text{x}}^{3}},{{\text{x}}^{2}},\text{x}$and constant term,
$A+C=0$
$B+D=4$
$4\text{ }A+3\text{ }C=0$
$4\text{ }B+3\text{ }D=10$
On solving these equations, to obtain $\text{A}=0.\text{B}=-2.\text{C}=0,and\,\,\,\text{D}=6$
$\therefore \dfrac{\left( 4{{x}^{2}}+10 \right)}{\left( {{x}^{2}}+3 \right)\left( {{x}^{2}}+4 \right)}=\dfrac{-2}{\left( {{x}^{2}}+3 \right)}+\dfrac{6}{\left( {{x}^{2}}+4 \right)}$
$\dfrac{\left( {{x}^{2}}+1 \right)\left( {{x}^{2}}+2 \right)}{\left( {{x}^{2}}+3 \right)\left( {{x}^{2}}+4 \right)}=\left( \dfrac{-2}{\left( {{x}^{2}}+3 \right)}+\dfrac{6}{\left( {{x}^{2}}+4 \right)} \right)$
$\Rightarrow \int{\dfrac{\left( {{x}^{2}}+1 \right)\left( {{x}^{2}}+2 \right)}{\left( {{x}^{2}}+3 \right)\left( {{x}^{2}}+4 \right)}}dx=\int{\left\{ 1+\dfrac{2}{\left( {{x}^{2}}+3 \right)}-\dfrac{6}{\left( {{x}^{2}}+4 \right)} \right\}}dx$
$=\int{\left\{ 1+\dfrac{2}{{{x}^{2}}+{{(\sqrt{3})}^{2}}}-\dfrac{6}{{{x}^{2}}+{{2}^{2}}} \right\}}$
$=x+2\left( \dfrac{1}{\sqrt{3}}{{\tan }^{-1}}\dfrac{x}{\sqrt{3}} \right)-6\left( \dfrac{1}{2}{{\tan }^{-1}}\dfrac{x}{2} \right)+C$
Simplifying,
$=x+\dfrac{2}{\sqrt{3}}{{\tan }^{-1}}\dfrac{x}{\sqrt{3}}-3{{\tan }^{-1}}\dfrac{x}{2}+C$
19. $\dfrac{2x}{\left( {{x}^{2}}+1 \right)\left( {{x}^{2}}+3 \right)}$
Ans: $\dfrac{2x}{\left( {{x}^{2}}+1 \right)\left( {{x}^{2}}+3 \right)}$
Put ${{x}^{2}}-t\to 2xdx-dt$
$\therefore \int{\dfrac{2x}{\left( {{x}^{2}}+1 \right)\left( {{x}^{2}}+3 \right)}}dx=\int{\dfrac{dt}{(t+1)(t+3)}}$
$\text{ Let }\dfrac{1}{(t+1)(t+3)}=\dfrac{A}{(t+1)}+\dfrac{B}{(t+3)}$
$I=A\left( t+3 \right)+B\left( t+1 \right)$
We obtain the below values by equating the coefficients of $\text{t}$and
constant,
$1+B=0$and $3A+B=1$
On solving, we get
$A=\dfrac{1}{2}\text{ and }B=-\dfrac{1}{2}$
$\therefore \dfrac{1}{(t+1)(t+3)}=\dfrac{1}{2(t+1)}+\dfrac{1}{2(t+3)}$
$\Rightarrow \int{\dfrac{2x}{\left( {{x}^{2}}+1 \right)\left( {{x}^{2}}+3 \right)}}dx=\int{\left\{ \dfrac{1}{2(t+1)}-\dfrac{1}{2(t+3)} \right\}}dt$
$=\dfrac{1}{2}\log |(t+1)|-\dfrac{1}{2}\log |t+3|+C$
Simplifying,
$=\dfrac{1}{2}\log \left| \dfrac{t+1}{t+3} \right|+C=\dfrac{1}{2}\log \left| \dfrac{{{x}^{2}}+1}{{{x}^{2}}+3} \right|+C$
20. $\dfrac{1}{x\left( {{x}^{4}}-1 \right)}$
Ans: $\dfrac{1}{x\left( {{x}^{4}}-1 \right)}$
Numerator and denominator are multiplied by by ${{\text{x}}^{3}},$we get
$\dfrac{1}{x\left( {{x}^{4}}-1 \right)}=\dfrac{{{x}^{3}}}{{{x}^{4}}\left( {{x}^{4}}-1 \right)}$
$\therefore \int{\dfrac{1}{x\left( {{x}^{4}}-1 \right)}}dx=\int{\dfrac{{{x}^{3}}}{{{x}^{4}}\left( {{x}^{4}}-1 \right)}}dx$
Consider ${{\text{x}}^{4}}=\text{t}\Rightarrow 4{{\text{x}}^{3}}\text{dx}=\text{dt}$
$\therefore \int{\dfrac{1}{x\left( {{x}^{4}}-1 \right)}}dx=\dfrac{1}{4}\int{\dfrac{dt}{t(t-1)}}$
$\text{ Let }\dfrac{1}{t(t-1)}=\dfrac{A}{t}+\dfrac{B}{(t-1)}$
$1=A(t-1)+Bt\quad \ldots (1)$
We obtain the below values by equating the coefficients of $\text{t}$and constant,
$A=-1\text{ and }B=1$
$\Rightarrow \dfrac{1}{t(t-1)}=\dfrac{-1}{t}+\dfrac{1}{t-1}$
$\Rightarrow \int{\dfrac{1}{x\left( {{x}^{4}}-1 \right)}}dx=\dfrac{1}{4}\int{\left\{ \dfrac{-1}{t}+\dfrac{1}{t-1} \right\}}dt$
Using the logarithm formula of integration,
$=\dfrac{1}{4}[-\log |t|+\log |t-1|]+C$
Simplifying,
$=\dfrac{1}{4}\log \left| \dfrac{t-1}{t} \right|+C=\dfrac{1}{4}\log \left| \dfrac{{{x}^{4}}-1}{{{x}^{4}}} \right|+C$
21. $\dfrac{1}{{{e}^{x}}-1}$ $\text{[}$ hint: Put ${{e}^{x}}=t$ $\text{]}$
Ans: $\dfrac{1}{\left( {{e}^{x}}-1 \right)}$
Put ${{\text{e}}^{x}}=\text{t }\Rightarrow {{\text{e}}^{x}}\text{dx}=dt$
$\Rightarrow \int{\dfrac{1}{\left( {{e}^{x}}-1 \right)}}dx=\int{\dfrac{1}{t-1}}\times \dfrac{dt}{t}=\int{\dfrac{1}{t(t-1)}}dt$
$\text{ Let }\dfrac{1}{t(t-1)}=\dfrac{A}{t}+\dfrac{B}{t-1}$
$1=A\left( t-1 \right)+B\text{ }t$
We obtain the below values by equating the coefficients of t and constant, $A=-1\text{ and }B=1$
$\therefore \dfrac{1}{t(t-1)}=\dfrac{-1}{t}+\dfrac{1}{t-1}$
$\Rightarrow \int{\dfrac{1}{t(t-1)}}dt=\log \left| \dfrac{t-1}{t} \right|+C$
Substitute the value of t,
$=\log \left| \dfrac{{{e}^{x}}-1}{{{e}^{x}}} \right|+C$
22. $\int{\dfrac{xdx}{\left( x-1 \right)\left( x-2 \right)}}$ equals
$\log \left| \dfrac{{{\left( x-1 \right)}^{2}}}{x-2} \right|+C$
$\log \left| \dfrac{{{\left( x-2 \right)}^{2}}}{x-1} \right|+C$
$\log \left| {{\left( \dfrac{x-1}{x-2} \right)}^{2}} \right|+C$
$\log \left| \left( x-1 \right)\left( x-2 \right) \right|+C$
Ans: Let $\dfrac{x}{(x-1)(x-2)}=\dfrac{A}{(x-1)}+\dfrac{B}{(x-2)}$
$x=A\left( x-2 \right)+B\left( x-1 \right)$
We obtain the below values by equating the coefficients of $\text{x}$and constant, $\text{A}=-1\,\,\,and\,\,\text{B}=2$
$\therefore \dfrac{x}{(x-1)(x-2)}=-\dfrac{1}{(x1)}+\dfrac{2}{(x-2)}$
$\Rightarrow \int{\dfrac{x}{(x-1)(x-2)}}dx=\int{\left\{ \dfrac{-1}{(x-1)}+\dfrac{2}{(x-2)} \right\}}dx$
Using the logarithm formula of integration,
$=-\log |x-1|+2\log |x-2|+C$
Simplifying,
$=\log \left| \dfrac{{{(x-2)}^{2}}}{x-1} \right|+C$
Thus, the right response is B.
23. $\int{\dfrac{dx}{x\left( {{x}^{2}}+1 \right)}}$ equals
$\log \left| x \right|-\dfrac{1}{2}\log \left( {{x}^{2}}+1 \right)+C$
$\log \left| x \right|+\dfrac{1}{2}\log \left( {{x}^{2}}+1 \right)+C$
$-\log \left| x \right|+\dfrac{1}{2}\log \left( {{x}^{2}}+1 \right)+C$
$\dfrac{1}{2}\log \left| x \right|+\log \left( {{x}^{2}}+1 \right)+C$
Ans: Let $\dfrac{1}{x\left( {{x}^{2}}+1 \right)}-\dfrac{A}{x},\dfrac{Bx+C}{{{x}^{2}}+1}$
$1=A\left( {{x}^{2}}+1 \right)+(Bx+C)x$
We obtain the below values by equating the coefficients of ${{\text{x}}^{2}},\text{x},$ and constant term,
$A+B=0$, $C=0$$A=1$
On solving these equations, to obtain
$A=1,B=-1,\text{ and }C=0$
$\therefore \dfrac{1}{x\left( {{x}^{2}},1 \right)}=\dfrac{1}{x}+\dfrac{-x}{{{x}^{2}}+1}$
$\Rightarrow \int{\dfrac{1}{x\left( {{x}^{2}}+1 \right)}}dx=\int{\left\{ \dfrac{1}{x}-\dfrac{x}{{{x}^{2}}+1} \right\}}dx$
$=\log |x|-\dfrac{1}{2}\log \left| {{x}^{2}}+1 \right|+C$
Thus, the right response is $\text{A}.$
Exercise 7.6
1. $x\sin x$
Ans: Let $I=\int{x}\sin xdx$
Consider $\text{u}=\text{x}$ and $\text{v}=\sin \text{x}$and integrating by parts, to obtain
$I=\int{x}\sin xdx-\int{\left\{ \left( \dfrac{d}{dx}x \right)\int{\sin }xdx \right\}}dx$
$=x(-\cos x)-\int{1}.(-\cos x)dx$
$=-x\cos x+\sin x+C$
2. $x\sin 3x$
Ans: Let $\text{I}=\int{x}\sin 3xdx$
Consider $\text{u}=\text{x}$ and $\text{v}=\sin 3\text{x}$ and integrating by parts, to obtain
$I=x\int{\sin }3xdx-\int{\left\{ \left( \dfrac{d}{dx}x \right)\int{\sin }3xdx \right\}}$
$=x\left( \dfrac{-\cos 3x}{3} \right)-\int{1}\cdot \left( \dfrac{-\cos 3x}{3} \right)dx$
$=\dfrac{-x\cos 3x}{3}+\dfrac{1}{3}\int{\cos }3xdx=\dfrac{-x\cos 3x}{3}+\dfrac{1}{9}\sin 3x+C$
3. ${{x}^{2}}{{e}^{x}}$
Ans: Let $I=\int{{{x}^{2}}}{{e}^{x}}dx$
Consider $\text{u}={{\text{x}}^{2}}\,\,and\,\,\text{v}={{\text{e}}^{x}}$
$I={{x}^{2}}\int{{{e}^{x}}}dx-\int{\left\{ \left( \dfrac{d}{dx}{{x}^{2}} \right)\int{{{e}^{x}}}dx \right\}}dx$
$={{x}^{2}}{{e}^{x}}-\int{2}x-{{e}^{x}}dx$
$={{x}^{2}}{{e}^{x}}-2\int{x}\cdot {{e}^{x}}dx$
Again using integration by parts, to obtain
$={{x}^{2}}{{e}^{x}}-2\left[ x\cdot \int{{{e}^{x}}}dx-\int{\left\{ \left( \dfrac{d}{dx}{{x}^{2}} \right)\int{{{e}^{x}}}dx \right\}}dx \right]$
$={{x}^{2}}{{e}^{x}}-2\left[ x{{e}^{x}}-\int{{{e}^{x}}}dx \right]$
Simplifying,
$={{x}^{2}}{{e}^{x}}-2\left[ x{{e}^{x}}-{{e}^{x}} \right]$
$={{x}^{2}}{{e}^{x}}-2x{{e}^{x}}+2{{e}^{x}}+C$
$={{e}^{x}}\left( {{x}^{2}}-2x+2 \right)+C$
4. $x\log x$
Ans: Let $I=\int{x}\log xdx$
Consider $\text{u}=\log \text{x}\,\,\,\,and\,\,\,\text{v}=\text{x}$ and integrating by parts, to obtain
$I=\log x\int{x}dx-\int{\left\{ \left( \dfrac{d}{dx}\log x \right)\int{x}dx \right\}}dx$
$=\log x\cdot \dfrac{{{x}^{2}}}{2}-\int{\dfrac{1}{x}}\cdot \dfrac{{{x}^{2}}}{2}dx$
$=\dfrac{{{x}^{2}}\log x}{2}\cdot \sqrt{\dfrac{x}{2}}dx=\dfrac{{{x}^{2}}\log x}{2}-\dfrac{{{x}^{2}}}{4}+C$
5. $x\log 2x$
Ans: Let $I=\int{x}\log 2xdx$
Consider $u=\log 2x$ and $v=x$and integrating by parts, to obtain
$I=\log 2x\int{x}dx-\int{\left\{ \left( \dfrac{d}{dx}2\log x \right)\int{x}dx \right\}}dx$
$=\log 2x\cdot \dfrac{{{x}^{2}}}{2}-\int{\dfrac{2}{2x}}\cdot \dfrac{{{x}^{2}}}{2}dx$
$=\dfrac{{{x}^{2}}\log 2x}{2}-\int{\dfrac{x}{2}}dx$
Integrating using the power rule
$=\dfrac{{{x}^{2}}\log 2x}{2}-\dfrac{{{x}^{2}}}{4}+C$
6. ${{x}^{2}}\log x$
Ans: Let $I=\int{{{x}^{2}}}\log xdx$
Consider $u=\log x$and $v={{x}^{2}}$ and integrating by parts, to obtain
$I=\log x\int{{{x}^{2}}}dx-\int{\left\{ \left( \dfrac{d}{dx}\log x \right)\int{{{x}^{2}}}dx \right\}}dx$
$=\log x\left( \dfrac{{{x}^{3}}}{3} \right)-\int{\dfrac{1}{x}}\cdot \dfrac{{{x}^{3}}}{3}dx$
Integrating using the power rule
$=\dfrac{{{x}^{3}}\log x}{3}-\int{\dfrac{{{x}^{2}}}{3}}dx=\dfrac{{{x}^{3}}\log x}{3}-\dfrac{{{x}^{3}}}{9}+C$
7. $x{{\sin }^{-1}}x$
Ans: Let $I=\int{x}{{\sin }^{-1}}xdx$
Consider $u={{\sin }^{-1}}x\,\,and\,\,\,v=x$ and integrating by parts, to obtain
$I={{\sin }^{-1}}x\int{x}dx\int{\left\{ \left( \dfrac{d}{dx}{{\sin }^{-1}}x \right)\int{x}dx \right\}}dx$
$={{\sin }^{-1}}x\left( \dfrac{{{x}^{2}}}{2} \right)-\int{\dfrac{1}{\sqrt{1-{{x}^{2}}}}}\cdot \dfrac{{{x}^{2}}}{2}dx$
$=\dfrac{{{x}^{2}}{{\sin }^{-1}}x}{2}+\dfrac{1}{2}\int{\dfrac{-{{x}^{2}}}{\sqrt{1-{{x}^{2}}}}}dx$
Adding and subtracting by 1
$=\dfrac{{{x}^{2}}{{\sin }^{-1}}x}{2}+\dfrac{1}{2}\int{\left\{ \dfrac{1-{{x}^{2}}}{\sqrt{1-{{x}^{2}}}}-\dfrac{1}{\sqrt{1-{{x}^{2}}}} \right\}}dx$
Simplifying,
$=\dfrac{{{x}^{2}}{{\sin }^{-1}}x}{2}+\dfrac{1}{2}\int{\left\{ \sqrt{1-{{x}^{2}}}-\dfrac{1}{\sqrt{1-{{x}^{2}}}} \right\}}dx$
$=\dfrac{{{x}^{2}}{{\sin }^{-1}}x}{2}+\dfrac{1}{2}\left\{ \int{\sqrt{1-{{x}^{2}}}}dx-\int{\dfrac{1}{\sqrt{1-{{x}^{2}}}}}dx \right\}$
$=\dfrac{{{x}^{2}}{{\sin }^{-1}}x}{2}+\dfrac{1}{2}\left\{ \dfrac{x}{2}\sqrt{1-{{x}^{2}}}+\dfrac{1}{2}{{\sin }^{-1}}x-{{\sin }^{-1}}x \right\}+C$
Simplifying, $=\dfrac{{{x}^{2}}{{\sin }^{-1}}x}{2}+\dfrac{x}{4}\sqrt{1-{{x}^{2}}}+\dfrac{1}{4}{{\sin }^{-1}}x-\dfrac{1}{2}{{\sin }^{-1}}x+C=\dfrac{1}{4}\left( 2{{x}^{2}}-1 \right){{\operatorname{in}}^{-1}}x+\dfrac{x}{4}\sqrt{1-{{x}^{2}}}+C$
8. $x{{\tan }^{-1}}x$
Ans: Let $I=\int{x}{{\tan }^{-1}}xdx$
Consider $\text{u}={{\tan }^{-1}}\text{x}$ and $\text{v}=\text{x}$and integrating by parts, to obtain
$I={{\tan }^{-1}}x\int{x}dx-\int{\left\{ \left( \dfrac{d}{dx}{{\tan }^{-1}}x \right)\int{x}dx \right\}}dx$
$={{\tan }^{-1}}x\left( \dfrac{{{x}^{2}}}{2} \right)\int{\dfrac{1}{1+{{x}^{2}}}}\cdot \dfrac{{{x}^{2}}}{2}dx=\dfrac{{{x}^{2}}{{\tan }^{-1}}x}{2}-\dfrac{1}{2}\int{\dfrac{{{x}^{2}}}{1+{{x}^{2}}}}dx$
Adding and subtracting by -1
$=\dfrac{{{x}^{2}}{{\tan }^{-1}}x}{2}-\dfrac{1}{2}\int{\left( \dfrac{{{x}^{2}}+1}{1+{{x}^{2}}}-\dfrac{1}{1+{{x}^{2}}} \right)}dx=\dfrac{{{x}^{2}}{{\tan }^{-1}}x}{2}-\dfrac{1}{2}\int{\left( 1-\dfrac{1}{1+{{x}^{2}}} \right)}dx$
Simplifying,
$=\dfrac{{{x}^{2}}{{\operatorname{lan}}^{-1}}x}{2}-\dfrac{1}{2}\left( x-{{\tan }^{-1}}x \right)+C=\dfrac{{{x}^{2}}}{2}{{\tan }^{-1}}x-\dfrac{x}{2}+\dfrac{1}{2}{{\tan }^{-1}}x+C$
9. $x{{\cos }^{-1}}x$
Ans:Let $I=\int{x}{{\cos }^{-1}}xdx$
Taking $u={{\cos }^{-1}}x$ and $\text{v}=\text{x}$and integrating by parts, to obtain
$I={{\cos }^{-1}}x\int{x}dx-\int{\left\{ \left( \dfrac{d}{dx}{{\cos }^{-1}}x \right)\int{x}dx \right\}}dx$
$={{\cos }^{-1}}x\dfrac{{{x}^{2}}}{2}-\int{\dfrac{-1}{\sqrt{1-{{x}^{2}}}}}\cdot \dfrac{{{x}^{2}}}{2}dx$
Adding and subtracting by -1
$=\dfrac{{{x}^{2}}{{\cos }^{-1}}x}{2}-\dfrac{1}{2}\int{\dfrac{1-{{x}^{2}}-1}{\sqrt{1-{{x}^{2}}}}}dx$
Simplifying,
$=\dfrac{{{x}^{2}}{{\cos }^{-1}}x}{2}-\dfrac{1}{2}\int{\left\{ \sqrt{1-{{x}^{2}}}+\left( \dfrac{-1}{\sqrt{1-{{x}^{2}}}} \right) \right\}}dx$
$=\dfrac{{{x}^{2}}{{\cos }^{-1}}x}{2}-\dfrac{1}{2}\int{\sqrt{1-{{x}^{2}}}}dx-\dfrac{1}{2}\int{\left( \dfrac{-1}{\sqrt{1-{{x}^{2}}}} \right)}dx$
$=\dfrac{{{x}^{2}}{{\cos }^{-1}}x}{2}-\dfrac{1}{2}{{I}_{1}}-\dfrac{1}{2}{{\cos }^{-1}}x....\left( 1 \right)$
Where ${{I}_{1}}=\int{\sqrt{1-{{x}^{2}}}}dx$
$\Rightarrow {{I}_{1}}=x\int{\sqrt{1-{{x}^{2}}}}-\int{\dfrac{d}{dx}}\sqrt{1-{{x}^{2}}}\int{x}dx\Rightarrow {{I}_{1}}=x\sqrt{1-{{x}^{2}}}-\int{\dfrac{-{{x}^{2}}}{\sqrt{1-{{x}^{2}}}}}dx$
$\Rightarrow {{I}_{1}}=x\sqrt{1-{{x}^{2}}}-\int{\dfrac{1-{{x}^{2}}-1}{\sqrt{1-{{x}^{2}}}}}dx\Rightarrow {{I}_{1}}=x\sqrt{1-{{x}^{2}}}-\left\{ \int{\sqrt{1-{{x}^{2}}}}dx+\int{\dfrac{-dx}{\sqrt{1-{{x}^{2}}}}} \right\}$
$\Rightarrow {{I}_{1}}=x\sqrt{1-{{x}^{2}}}-\left\{ {{I}_{1}}+{{\cos }^{-1}}x \right\}\Rightarrow 2{{I}_{1}}=x\sqrt{1-{{x}^{2}}}-{{\cos }^{-1}}x$
$\therefore {{I}_{1}}=\dfrac{x}{2}\sqrt{1-{{x}^{2}}}-\dfrac{1}{2}{{\cos }^{-1}}x$
Substituting in (1), we get
$I=\dfrac{x{{\cos }^{-1}}x}{2}-\dfrac{1}{2}\left( \dfrac{x}{2}\sqrt{1-{{x}^{2}}}-\dfrac{1}{2}{{\cos }^{-1}}x \right)-\dfrac{1}{2}{{\cos }^{-1}}x$
Simplifying,
$=\dfrac{\left( 2{{x}^{2}}-1 \right)}{4}{{\cos }^{-1}}x-\dfrac{x}{4}\sqrt{1-{{x}^{2}}}+C$
10. ${{\left( {{\sin }^{-1}}x \right)}^{2}}$
Ans: Let $I=\int{{{\left( {{\sin }^{-1}}x \right)}^{2}}}\cdot 1dx$
Consider $\text{u}={{\left( {{\sin }^{-1}}\text{x} \right)}^{2}}$ and $\text{v}=1$and integrating by parts, to obtain
$I=\int{\left( {{\sin }^{-1}}x \right)}\cdot \int{1}dx-\int{\left\{ \dfrac{d}{dx}{{\left( {{\sin }^{-1}}x \right)}^{2}}\cdot \int{1}.dx \right\}}dx$
$={{\left( {{\sin }^{-1}}x \right)}^{2}}x-\int{\dfrac{2{{\sin }^{-1}}x}{\sqrt{1-{{x}^{2}}}}}\cdot xdx$
$=x{{\left( {{\sin }^{-1}}x \right)}^{2}}+\int{{{\sin }^{-1}}}x\cdot \left( \dfrac{-2x}{\sqrt{1-{{x}^{2}}}} \right)dx$
$=x{{\left( {{\sin }^{-1}}x \right)}^{2}}+\left[ {{\sin }^{-1}}x\int{\dfrac{-2x}{\sqrt{1-{{x}^{2}}}}}dx-\int{\left\{ \left( \dfrac{d}{dx}{{\sin }^{-1}}x \right)\int{\dfrac{-2x}{\sqrt{1-{{x}^{2}}}}}dx \right\}}dx \right]$
$=x{{\left( {{\sin }^{-1}}x \right)}^{2}}+\left[ {{\sin }^{-1}}x\cdot 2\sqrt{1-{{x}^{2}}}-\int{\dfrac{1}{\sqrt{1-{{x}^{2}}}}}\cdot 2\sqrt{1-{{x}^{2}}}dx \right]$
$=x{{\left( {{\sin }^{-1}}x \right)}^{2}}+2\sqrt{1-{{x}^{2}}}{{\sin }^{-1}}x-\int{2}dx$
$=x{{\left( {{\sin }^{-1}}x \right)}^{2}}+2\sqrt{1-{{x}^{2}}}{{\sin }^{-1}}x-2x+C$
11. $\dfrac{x{{\cos }^{-1}}x}{\sqrt{1-{{x}^{2}}}}$
Ans: Let $I=\int{\dfrac{x{{\cos }^{-1}}x}{\sqrt{1-{{x}^{2}}}}}dx$
Multiplying and dividing by 2
$I=\dfrac{-1}{2}\int{\dfrac{-2x}{\sqrt{1-{{x}^{2}}}}}\cdot {{\cos }^{-1}}xdx$
Consider $\text{u}={{\cos }^{-1}}\text{x}$ and $\text{v}=\left( \dfrac{-2x}{\sqrt{1-{{x}^{2}}}} \right)$and integrating by parts, to obtain
$I=\dfrac{-1}{2}\left[ {{\cos }^{-1}}x\int{\dfrac{-2x}{\sqrt{1-{{x}^{2}}}}}dx-\int{\left\{ \left( \dfrac{d}{dx}{{\cos }^{-1}}x \right)\int{\dfrac{-2x}{\sqrt{1-{{x}^{2}}}}}dx \right\}}dx \right]$
$=\dfrac{-1}{2}\left[ {{\cos }^{-1}}x\cdot 2\sqrt{1-{{x}^{2}}}-\int{\dfrac{-1}{\sqrt{1-{{x}^{2}}}}}\cdot 2\sqrt{1-{{x}^{2}}}dx \right]=\dfrac{-1}{2}\left[ 2\sqrt{1-{{x}^{2}}}{{\cos }^{-1}}x+\int{2}dx \right]$
Simplifying,
$=\dfrac{-1}{2}\left[ 2\sqrt{1-{{x}^{2}}}{{\cos }^{-1}}x+2x \right]+C$
$=-\left[ \sqrt{1-{{x}^{2}}}{{\cos }^{-1}}x+x \right]+C$
12. $x{{\sec }^{2}}x$
Ans:Let $I=\int{x}{{\sec }^{2}}xdx$
Consider$\text{u}=\text{x}$ and $\text{v}={{\sec }^{2}}\text{x}$ and integrating by parts, to obtain
$I=x\int{{{\sec }^{2}}}xdx-\int{\left\{ \left\{ \dfrac{d}{dx}x \right\}\int{{{\sec }^{2}}}xdx \right\}}dx$
$=x\tan x-\int{1}\cdot \tan xdx$
$=x\tan x+\log |\cos x|+C$
13. ${{\tan }^{-1}}x$
Ans: Let $I=\int{1}\cdot {{\tan }^{-1}}xdx$
Consider $\text{u}={{\tan }^{-1}}\text{x}$ and $\text{v}=1$ and integrating by parts, to obtain
$I={{\tan }^{-1}}x\int{1}dx-\int{\left\{ \left( \dfrac{d}{dx}{{\tan }^{-1}}x \right)\int{1}.dx \right\}}dx={{\tan }^{-1}}xx-\int{\dfrac{1}{1+{{x}^{2}}}}xd$
$=x{{\tan }^{-1}}x-\dfrac{1}{2}\int{\dfrac{2x}{1+{{x}^{2}}}}dx$
$=x{{\tan }^{-1}}x-\dfrac{1}{2}\log \left| 1+{{x}^{2}} \right|+C$
$=x{{\tan }^{-1}}x-\dfrac{1}{2}\log \left( 1+{{x}^{2}} \right)+C$
14. $x{{\left( \log x \right)}^{2}}dx$
Ans: $I=\int{x}{{(\log x)}^{2}}dx$
Consider$u={{(\log x)}^{2}}$ and $v=1$ and integrating by parts, to obtain
$I={{(\log )}^{2}}\int{x}dx-\int{\left[ \left\{ {{\left( \dfrac{d}{dx}\log x \right)}^{2}} \right\}\int{x}dx \right]}dx$
$=\dfrac{{{x}^{2}}}{2}{{(\log x)}^{2}}-\left[ \int{2}\log x\cdot \dfrac{1}{x}\cdot \dfrac{{{x}^{2}}}{2}dx \right]$
$=\dfrac{{{x}^{2}}}{2}{{(\log x)}^{2}}-\int{x}\log xdx$
Again using integration by parts, to obtain
$I=\dfrac{{{x}^{2}}}{2}{{(\log x)}^{2}}-\left[ \log x\int{x}dx-\int{\left\{ \left( \dfrac{d}{dx}\log x \right)\int{x}dx \right\}}dx \right]$
$=\dfrac{{{x}^{2}}}{2}{{(\log x)}^{2}}-\left[ \dfrac{{{x}^{2}}}{2}-\log x-\int{\dfrac{1}{x}}\cdot \dfrac{{{x}^{2}}}{2}dx \right]$
$=\dfrac{{{x}^{2}}}{2}{{(\log x)}^{2}}-\dfrac{{{x}^{2}}}{2}\log x+\dfrac{1}{2}\int{x}dx=\dfrac{{{x}^{2}}}{2}{{(\log x)}^{2}}-\dfrac{{{x}^{2}}}{2}\log x+\dfrac{{{x}^{2}}}{4}+C$
15. $\left( {{x}^{2}}+1 \right)\log x$
Ans: Let $I=\int{\left( {{x}^{2}}+1 \right)}\log xdx=\int{{{x}^{2}}}\log xdx+\int{\log }xdx$
Let $\text{I}={{\text{I}}_{1}}+{{\text{I}}_{2}}\ldots (1)$
Where,${{I}_{1}}=\int{{{x}^{2}}}\log xdx\,\,\,\,\,\,and\,\,\,{{\text{I}}_{2}}=\int{\log }xdx$
${{I}_{1}}=\int{{{x}^{2}}}\log xdx$
Consider$\text{u}=\log \text{x}$ and $v=x^2$ and integrating by parts, to obtain
${{I}_{1}}=\log x-\int{{{x}^{2}}}dx-\int{\left\{ \left( \dfrac{d}{dx}\log x \right)\int{{{x}^{2}}}dx \right\}}dx$
$=\log x\cdot \dfrac{{{x}^{3}}}{3}-\int{\dfrac{1}{x}}\cdot \dfrac{{{x}^{3}}}{3}dx=\dfrac{{{x}^{3}}}{3}\log x-\dfrac{1}{3}\int{{{x}^{2}}}dx$
$=\dfrac{{{x}^{3}}}{3}\log x-\dfrac{{{x}^{3}}}{9}+{{C}_{1}}\quad \ldots (2)$
${{I}_{2}}=\int{\log }xdx$
Consider $\text{u}=\log \text{x}$ and $\text{v}=1$and integrating by parts, to obtain
${{I}_{2}}=\log x\int{1}.dx-\int{\left\{ \left( \dfrac{d}{dx}\log x \right)\int{1}.dx \right\}}$
$=\log x\cdot x-\int{\dfrac{1}{x}}xdx$
$=x\log x-x..\left( 3 \right)$
Using equations (2) and (3) in (1), we get
$I=\dfrac{{{x}^{3}}}{3}\log x-\dfrac{{{x}^{3}}}{9}+{{C}_{1}}+x\log x-x+{{C}_{2}}$
$=\dfrac{{{x}^{3}}}{3}\log x-\dfrac{{{x}^{3}}}{9}+x\log x-x+\left( {{C}_{1}}+{{C}_{2}} \right)$
$=\left( \dfrac{{{x}^{3}}}{3}+x \right)\log x-\dfrac{{{x}^{3}}}{9}-x+C$
16. ${{e}^{x}}\left( \sin x+\cos x \right)$
Ans: Consider$I=\int{{{e}^{x}}}(\sin x+\cos x)dx$
Consider$f(x)=\sin x$
${{f}^{\prime }}(x)=\cos x$
$I=\int{{{e}^{x}}}\left\{ f(x)+{{f}^{\prime }}(x) \right\}dx$
Since, $\int{{{e}^{x}}}\left\{ f(x)+{{f}^{\prime }}(x) \right\}dx={{e}^{x}}f(x)+C$
$\therefore I={{e}^{x}}\sin x+C$
17. $\dfrac{x{{e}^{x}}}{{{\left( 1+x \right)}^{2}}}$
Ans: Consider $I=\int{\dfrac{x{{e}^{x}}}{{{(1+x)}^{2}}}}dx=\int{{{e}^{x}}}\left\{ \dfrac{x}{{{(1+x)}^{2}}} \right\}dx$
$=\int{{{e}^{x}}}\left\{ \dfrac{1+x-1}{{{(1+x)}^{2}}} \right\}dx=\int{{{e}^{x}}}\left\{ \dfrac{1}{1+x}-\dfrac{1}{{{(1+x)}^{2}}} \right\}dx$
Here, $f(x)=\dfrac{1}{1+x}\quad {{f}^{\prime }}(x)=\dfrac{-1}{{{(1+x)}^{2}}}$
$\Rightarrow \int{\dfrac{x{{e}^{x}}}{{{(1+x)}^{2}}}}dx=\int{{{e}^{x}}}\left\{ f(x)+{{f}^{\prime }}(x) \right\}dx$
Since, $\int{{{e}^{x}}}\left\{ f(x)+{{f}^{\prime }}(x) \right\}dx={{e}^{x}}f(x)+C$
$\therefore \int{\dfrac{x{{e}^{x}}}{{{(1+x)}^{2}}}}dx=\dfrac{{{e}^{x}}}{1+x}+C$
18. Integrate the function - ${{e}^{x}}\left( \dfrac{1+\sin x}{1+\cos x} \right)$
Ans: First simplify –${{e}^{x}}\left( \dfrac{1+\sin x}{1+\cos x} \right)$
It is known that –
$1+\sin x={{\sin }^{2}}\dfrac{x}{2}+{{\cos }^{2}}\dfrac{x}{2}+2\sin \dfrac{x}{2}\cos \dfrac{x}{2}$
$1+\cos x=2{{\cos }^{2}}\dfrac{x}{2}$
$\therefore {{e}^{x}}\left( \dfrac{1+\sin x}{1+\cos x} \right)={{e}^{x}}\left( \dfrac{{{\sin }^{2}}\dfrac{x}{2}+{{\cos }^{2}}\dfrac{x}{2}+2\sin \dfrac{x}{2}\cos \dfrac{x}{2}}{2{{\cos }^{2}}\dfrac{x}{2}} \right)$
$={{e}^{x}}\left( \dfrac{{{\left( \sin \dfrac{x}{2}+\cos \dfrac{x}{2} \right)}^{2}}}{2{{\cos }^{2}}\dfrac{x}{2}} \right)$
$=\dfrac{1}{2}{{e}^{x}}\left( \dfrac{{{\left( \sin \dfrac{x}{2}+\cos \dfrac{x}{2} \right)}^{2}}}{{{\cos }^{2}}\dfrac{x}{2}} \right)$
$=\dfrac{1}{2}{{e}^{x}}{{\left( \dfrac{\sin \dfrac{x}{2}+\cos \dfrac{x}{2}}{\cos \dfrac{x}{2}} \right)}^{2}}$
$=\dfrac{1}{2}{{e}^{x}}{{\left( \dfrac{\sin \dfrac{x}{2}}{\cos \dfrac{x}{2}}+\dfrac{\cos \dfrac{x}{2}}{\cos \dfrac{x}{2}} \right)}^{2}}$
$=\dfrac{1}{2}{{e}^{x}}{{\left( \tan \dfrac{x}{2}+1 \right)}^{2}}$
$=\dfrac{1}{2}{{e}^{x}}\left( {{\tan }^{2}}\dfrac{x}{2}+1+2\tan \dfrac{x}{2} \right)$
But, $1+{{\tan }^{2}}\dfrac{x}{2}={{\sec }^{2}}\dfrac{x}{2}$
$=\dfrac{1}{2}{{e}^{x}}\left( {{\sec }^{2}}\dfrac{x}{2}+2\tan \dfrac{x}{2} \right)$
$={{e}^{x}}\left( \dfrac{1}{2}{{\sec }^{2}}\dfrac{x}{2}+\tan \dfrac{x}{2} \right)$
$\Rightarrow {{e}^{x}}\left( \dfrac{1+\sin x}{1+\cos x} \right)={{e}^{x}}\left( \dfrac{1}{2}{{\sec }^{2}}\dfrac{x}{2}+\tan \dfrac{x}{2} \right)$
It is known that, $\int{{{e}^{x}}\left\{ f(x)+f'(x) \right\}dx=}{{e}^{x}}f(x)+C$
If we say, $f(x)=\tan \dfrac{x}{2}\Rightarrow f'(x)=\dfrac{1}{2}{{\sec }^{2}}\dfrac{x}{2}$
Thus, we get – $\int{{{e}^{x}}\left( \dfrac{1+\sin x}{1+\cos x} \right)}dx={{e}^{x}}\tan \dfrac{x}{2}+C$
19. Integrate the function - ${{e}^{x}}\left( \dfrac{1}{x}-\dfrac{1}{{{x}^{2}}} \right)$
Ans: Say, $I=\int{{{e}^{x}}\left( \dfrac{1}{x}-\dfrac{1}{{{x}^{2}}} \right)dx}$
Suppose, $f(x)=\dfrac{1}{x}\Rightarrow f'(x)=-\dfrac{1}{{{x}^{2}}}$
It is known that, $\int{{{e}^{x}}\left\{ f(x)+f'(x) \right\}dx=}{{e}^{x}}f(x)+C$
Thus, we get – $I=\int{{{e}^{x}}\left( \dfrac{1}{x}-\dfrac{1}{{{x}^{2}}} \right)dx}=\dfrac{{{e}^{x}}}{x}+C$
20. Integrate the function - $\dfrac{(x-3){{e}^{x}}}{{{(x-1)}^{3}}}$
Ans: $\int{{{e}^{x}}\dfrac{(x-3)}{{{(x-1)}^{3}}}dx=\int{{{e}^{x}}\left[ \dfrac{(x-1-2)}{{{(x-1)}^{3}}} \right]dx}}$
$=\int{{{e}^{x}}\left[ \dfrac{(x-1)}{{{(x-1)}^{3}}}-\dfrac{2}{{{(x-1)}^{3}}} \right]dx}$
$=\int{{{e}^{x}}\left[ \dfrac{1}{{{(x-1)}^{2}}}-\dfrac{2}{{{(x-1)}^{3}}} \right]dx}$
Suppose, $f(x)=\dfrac{1}{{{(x-1)}^{2}}}\Rightarrow f'(x)=-\dfrac{2}{{{(x-1)}^{3}}}$
It is known that, $\int{{{e}^{x}}\left\{ f(x)+f'(x) \right\}dx=}{{e}^{x}}f(x)+C$
Thus, $\int{{{e}^{x}}\dfrac{(x-3)}{{{(x-1)}^{3}}}dx=\dfrac{{{e}^{x}}}{{{(x-1)}^{2}}}+C}$
21. Integrate the function - ${{e}^{2x}}\sin x$
Ans: Say, $I=\int{{{e}^{2x}}\sin xdx}$
Perform Integration by parts – $\int{uv}dx=u\int{vdx}-\int{\left( u'\int{vdx} \right)dx}$
With –$u=\sin x\text{ }v={{e}^{2x}}$
$I=\int{{{e}^{2x}}\sin x}dx=\sin x\int{{{e}^{2x}}dx}-\int{\left[ \left( \dfrac{d}{dx}\sin x \right)\int{{{e}^{2x}}dx} \right]dx}$
$=\sin x\dfrac{{{e}^{2x}}}{2}-\int{\left[ \left( \cos x \right)\dfrac{{{e}^{2x}}}{2} \right]dx}$
$=\sin x\dfrac{{{e}^{2x}}}{2}-\dfrac{1}{2}\int{\left( {{e}^{2x}}\cos x \right)dx}$
Perform Integration by parts for – $\int{\left( {{e}^{2x}}\cos x \right)dx}$
$=\sin x\dfrac{{{e}^{2x}}}{2}-\dfrac{1}{2}\left\{ \cos x\int{{{e}^{2x}}dx}-\int{\left[ \left( \dfrac{d}{dx}\cos x \right)\int{{{e}^{2x}}dx} \right]dx} \right\}$
$=\sin x\dfrac{{{e}^{2x}}}{2}-\dfrac{1}{2}\left\{ \cos x\dfrac{{{e}^{2x}}}{2}-\int{\left[ \left( -\sin x \right)\dfrac{{{e}^{2x}}}{2} \right]dx} \right\}$
$=\sin x\dfrac{{{e}^{2x}}}{2}-\dfrac{1}{2}\left\{ \cos x\dfrac{{{e}^{2x}}}{2}+\dfrac{1}{2}\int{(\sin x){{e}^{2x}}dx} \right\}$
$=\sin x\dfrac{{{e}^{2x}}}{2}-\dfrac{{{e}^{2x}}\cos x}{4}-\dfrac{1}{4}\left\{ \int{(\sin x){{e}^{2x}}dx} \right\}$
But, $I=\int{{{e}^{2x}}\sin xdx}$
$\Rightarrow I=\sin x\dfrac{{{e}^{2x}}}{2}-\dfrac{{{e}^{2x}}\cos x}{4}-\dfrac{1}{4}I$
$\Rightarrow I+\dfrac{1}{4}I=\sin x\dfrac{{{e}^{2x}}}{2}-\dfrac{{{e}^{2x}}\cos x}{4}$
$\Rightarrow \dfrac{5}{4}I=\dfrac{{{e}^{2x}}\sin x}{2}-\dfrac{{{e}^{2x}}\cos x}{4}$
$\Rightarrow \dfrac{5}{4}I=\dfrac{2{{e}^{2x}}\sin x}{4}-\dfrac{{{e}^{2x}}\cos x}{4}$
$\Rightarrow 5I={{e}^{2x}}(2\sin x-\cos x)$
Thus, we get – $I=\dfrac{{{e}^{2x}}}{5}(2\sin x-\cos x)+C$.
22. Integrate the function - ${{\sin }^{-1}}\left( \dfrac{2x}{1+{{x}^{3}}} \right)$
Ans: Say, $x=\tan \theta \text{ }\Rightarrow \text{dx=se}{{\text{c}}^{2}}\theta d\theta $
$\therefore {{\sin }^{-1}}\left( \dfrac{2x}{1+{{x}^{3}}} \right)={{\sin }^{-1}}\left( \dfrac{2\tan \theta }{1+{{\tan }^{3}}\theta } \right)$
But, $\sin 2\theta =\dfrac{2\tan \theta }{1+{{\tan }^{3}}\theta }$
$\Rightarrow {{\sin }^{-1}}\left( \dfrac{2x}{1+{{x}^{3}}} \right)={{\sin }^{-1}}\left( \dfrac{2\tan \theta }{1+{{\tan }^{3}}\theta } \right)=\therefore {{\sin }^{-1}}\left( \dfrac{2x}{1+{{x}^{3}}} \right)={{\sin }^{-1}}\left( \sin 2\theta \right)=2\theta $
Therefore, $\int{{{\sin }^{-1}}\left( \dfrac{2x}{1+{{x}^{3}}} \right)dx}=\int{2\theta \text{se}{{\text{c}}^{2}}\theta d\theta }$
$=2\int{\theta \text{se}{{\text{c}}^{2}}\theta d\theta }$
Perform Integration by parts – $\int{uv}dx=u\int{vdx}-\int{\left( u'\int{vdx} \right)dx}$
With –$u=\theta \text{ }v={{\sec }^{2}}\theta $
$2\int{\theta \text{se}{{\text{c}}^{2}}\theta d\theta }=2\left\{ \theta \int{{{\sec }^{2}}\theta d\theta }-\int{\left[ \left( \dfrac{d}{d\theta }\theta \right)\int{{{\sec }^{2}}\theta d\theta } \right]d\theta } \right\}$
$=2\left\{ \theta \tan \theta -\int{\left[ \tan \theta \right]d\theta } \right\}$
$=2\left\{ \theta \tan \theta -(-\log |\cos \theta |) \right\}+C$
$=2\left\{ \theta \tan \theta +\log |\cos \theta | \right\}+C$
Replace $\theta ={{\tan }^{-1}}x$
$=2\left\{ {{\tan }^{-1}}x\tan ({{\tan }^{-1}}x)+\log |\cos ({{\tan }^{-1}}x)| \right\}+C$
It is known that – ${{\tan }^{-1}}x={{\cos }^{-1}}\dfrac{1}{\sqrt{1+{{x}^{2}}}}$
$=2\left\{ {{\tan }^{-1}}x(x)+\log |\cos ({{\cos }^{-1}}\dfrac{1}{\sqrt{1+{{x}^{2}}}})| \right\}+C$
$=2\left\{ x{{\tan }^{-1}}x+\log |\dfrac{1}{\sqrt{1+{{x}^{2}}}}| \right\}+C$
$=2\left\{ x{{\tan }^{-1}}x+\log {{(1+{{x}^{2}})}^{-\dfrac{1}{2}}} \right\}+C$
Here, $\log {{m}^{n}}=n\log m$
$=2\left\{ x{{\tan }^{-1}}x-\dfrac{1}{2}\log (1+{{x}^{2}}) \right\}+C$
$=2x{{\tan }^{-1}}x-\log (1+{{x}^{2}})+C$
Thus, $\int{{{\sin }^{-1}}\left( \dfrac{2x}{1+{{x}^{3}}} \right)dx}=2x{{\tan }^{-1}}x-\log (1+{{x}^{2}})+C$
23. Choose the correct answer: $\int{{{x}^{2}}{{e}^{{{x}^{3}}}}}dx$ equals
$\dfrac{1}{3}{{e}^{{{x}^{3}}}}+C$
$\dfrac{1}{3}{{e}^{{{x}^{2}}}}+C$
$\dfrac{1}{2}{{e}^{{{x}^{3}}}}+C$
$\dfrac{1}{2}{{e}^{{{x}^{2}}}}+C$
Ans: Say, $I=\int{{{x}^{2}}{{e}^{{{x}^{3}}}}}dx$
Suppose, $t={{x}^{3}}\Rightarrow dt=3{{x}^{2}}dx$
Rewriting the equation – $I=\int{{{x}^{2}}{{e}^{{{x}^{3}}}}}dx=\dfrac{1}{3}\int{{{e}^{t}}}dt$
$\Rightarrow I=\dfrac{1}{3}\int{{{e}^{t}}}dt=\dfrac{1}{3}{{e}^{t}}+C$
Replacing $t={{x}^{3}}$
$\Rightarrow I=\dfrac{1}{3}{{e}^{{{x}^{3}}}}+C$
The correct option is A.
24. Choose the correct answer: $\int{{{e}^{x}}\sec x(1+\tan x)}dx$
${{e}^{x}}\cos x+C$
${{e}^{x}}\sec x+C$
${{e}^{x}}\sin x+C$
${{e}^{x}}\tan x+C$
Ans: Say, $I=\int{{{e}^{x}}\sec x(1+\tan x)}dx$
$\Rightarrow I=\int{{{e}^{x}}(\sec x+\sec x\tan x)}dx$
Suppose, $f(x)=\sec x\Rightarrow f'(x)=\sec x\tan x$
It is known that, $\int{{{e}^{x}}\left\{ f(x)+f'(x) \right\}dx=}{{e}^{x}}f(x)+C$
$\Rightarrow I=\int{{{e}^{x}}(\sec x+\sec x\tan x)}dx={{e}^{x}}\sec x+C$
Thus, $I={{e}^{x}}\sec x+C$
The correct option is B.
Exercise 7.7
1. Integrate the function - $\sqrt{4-{{x}^{2}}}$
Ans: Say, $I=\int{\sqrt{4-{{x}^{2}}}dx}=\int{\sqrt{{{2}^{2}}-{{x}^{2}}}dx}$
It is known that – $\int{\sqrt{{{a}^{2}}-{{x}^{2}}}dx=\dfrac{x}{2}\sqrt{{{a}^{2}}-{{x}^{2}}}+\dfrac{{{a}^{2}}}{2}{{\sin }^{-1}}\dfrac{x}{a}+C}$
$\Rightarrow I=\int{\sqrt{{{2}^{2}}-{{x}^{2}}}dx=\dfrac{x}{2}\sqrt{{{2}^{2}}-{{x}^{2}}}+\dfrac{{{2}^{2}}}{2}{{\sin }^{-1}}\dfrac{x}{2}+C}$
$\Rightarrow I=\dfrac{x}{2}\sqrt{4-{{x}^{2}}}+2{{\sin }^{-1}}\dfrac{x}{2}+C$
Thus, $\int{\sqrt{4-{{x}^{2}}}dx}=\dfrac{x}{2}\sqrt{4-{{x}^{2}}}+2{{\sin }^{-1}}\dfrac{x}{2}+C$
2. Integrate the function - $\sqrt{1-4{{x}^{2}}}$
Ans: Say, $I=\int{\sqrt{1-4{{x}^{2}}}dx}=\int{\sqrt{{{1}^{2}}-{{(2x)}^{2}}}dx}$
Let, $2x=t\Rightarrow 2dx=dt$
$x=\dfrac{t}{2}\Rightarrow dx=\dfrac{dt}{2}$
So, we get – $I=\int{\sqrt{{{1}^{2}}-{{\left[ 2(\dfrac{t}{2}) \right]}^{2}}}\dfrac{dt}{2}}=\dfrac{1}{2}\int{\sqrt{{{1}^{2}}-{{\left[ t \right]}^{2}}}dt}$
$\Rightarrow I=\dfrac{1}{2}\int{\sqrt{{{1}^{2}}-{{\left[ t \right]}^{2}}}dt}$
It is known that – $\int{\sqrt{{{a}^{2}}-{{x}^{2}}}dx=\dfrac{x}{2}\sqrt{{{a}^{2}}-{{x}^{2}}}+\dfrac{{{a}^{2}}}{2}{{\sin }^{-1}}\dfrac{x}{a}+C}$
$\Rightarrow I=\dfrac{1}{2}\left[ \dfrac{t}{2}\sqrt{1-{{t}^{2}}}+\dfrac{1}{2}{{\sin }^{-1}}t \right]+C$
$\Rightarrow I=\left[ \dfrac{t}{4}\sqrt{1-{{t}^{2}}}+\dfrac{1}{4}{{\sin }^{-1}}t \right]+C$
Replace – $t=2x$
$\Rightarrow I=\left[ \dfrac{2x}{4}\sqrt{1-{{(2x)}^{2}}}+\dfrac{1}{4}{{\sin }^{-1}}2x \right]+C$
$\Rightarrow I=\left[ \dfrac{x}{2}\sqrt{1-4{{x}^{2}}}+\dfrac{1}{4}{{\sin }^{-1}}2x \right]+C$
Thus,$\int{\sqrt{1-4{{x}^{2}}}dx}=\dfrac{x}{2}\sqrt{1-4{{x}^{2}}}+\dfrac{1}{4}{{\sin }^{-1}}2x+C$
3. Integrate the function - $\sqrt{{{x}^{2}}+4x+6}$
Ans: First simplify –${{x}^{2}}+4x+6$
${{x}^{2}}+4x+6={{x}^{2}}+4x+4+2$
$=({{x}^{2}}+4x+4)+2={{(x+2)}^{2}}+{{(\sqrt{2})}^{2}}$
$\Rightarrow \sqrt{{{x}^{2}}+4x+6}=\sqrt{{{(x+2)}^{2}}+{{(\sqrt{2})}^{2}}}$
$\therefore \int{\sqrt{{{x}^{2}}+4x+6}dx}=\int{\sqrt{{{(x+2)}^{2}}+{{(\sqrt{2})}^{2}}}dx}$
It is known that – $\int{\sqrt{{{x}^{2}}+{{a}^{2}}}dx=\dfrac{x}{2}\sqrt{{{x}^{2}}+{{a}^{2}}}+\dfrac{{{a}^{2}}}{2}\log \left| x+\sqrt{{{x}^{2}}+{{a}^{2}}} \right|+C}$
$\Rightarrow \int{\sqrt{{{(x+2)}^{2}}+{{(\sqrt{2})}^{2}}}dx}=\dfrac{x+2}{2}\sqrt{{{(x+2)}^{2}}+{{(\sqrt{2})}^{2}}}+\dfrac{{{(\sqrt{2})}^{2}}}{2}\log \left| (x+2)+\sqrt{{{(x+2)}^{2}}+{{(\sqrt{2})}^{2}}} \right|+C$$=\dfrac{x+2}{2}\sqrt{{{x}^{2}}+4x+6}+\dfrac{2}{2}\log \left| (x+2)+\sqrt{{{x}^{2}}+4x+6} \right|+C$
Thus, $\int{\sqrt{{{x}^{2}}+4x+6}dx}=\dfrac{x+2}{2}\sqrt{{{x}^{2}}+4x+6}+\log \left| (x+2)+\sqrt{{{x}^{2}}+4x+6} \right|+C$
4. Integrate the function - $\sqrt{{{x}^{2}}+4x+1}$
Ans: First simplify –${{x}^{2}}+4x+1$
${{x}^{2}}+4x+1={{x}^{2}}+4x+4-3$
$=({{x}^{2}}+4x+4)-3={{(x+2)}^{2}}-{{(\sqrt{3})}^{2}}$
$\Rightarrow \sqrt{{{x}^{2}}+4x+1}=\sqrt{{{(x+2)}^{2}}-{{(\sqrt{3})}^{2}}}$
$\therefore \int{\sqrt{{{x}^{2}}+4x+1}dx}=\int{\sqrt{{{(x+2)}^{2}}-{{(\sqrt{3})}^{2}}}dx}$
It is known that – $\int{\sqrt{{{x}^{2}}-{{a}^{2}}}dx=\dfrac{x}{2}\sqrt{{{x}^{2}}-{{a}^{2}}}-\dfrac{{{a}^{2}}}{2}\log \left| x+\sqrt{{{x}^{2}}-{{a}^{2}}} \right|+C}$
$\Rightarrow \int{\sqrt{{{(x+2)}^{2}}-{{(\sqrt{3})}^{2}}}dx}=\dfrac{x+2}{2}\sqrt{{{(x+2)}^{2}}-{{(\sqrt{3})}^{2}}}-\dfrac{{{(\sqrt{3})}^{2}}}{2}\log \left| (x+2)+\sqrt{{{(x+2)}^{2}}-{{(\sqrt{3})}^{2}}} \right|+C$$=\dfrac{x+2}{2}\sqrt{{{x}^{2}}+4x+1}-\dfrac{3}{2}\log \left| (x+2)+\sqrt{{{x}^{2}}+4x+1} \right|+C$
Thus, $\int{\sqrt{{{x}^{2}}+4x+1}dx}=\dfrac{x+2}{2}\sqrt{{{x}^{2}}+4x+1}-\dfrac{3}{2}\log \left| (x+2)+\sqrt{{{x}^{2}}+4x+1} \right|+C$
5. Integrate the function - $\sqrt{1-4x-{{x}^{2}}}$
Ans: First simplify –$1-4x-{{x}^{2}}$
$1-4x-{{x}^{2}}=1-4x-{{x}^{2}}-4+4=1+4-({{x}^{2}}+4x+4)$
$=5-({{x}^{2}}+4x+4)={{(\sqrt{5})}^{2}}-{{(x+2)}^{2}}$
$\Rightarrow \sqrt{1-4x-{{x}^{2}}}=\sqrt{{{(\sqrt{5})}^{2}}-{{(x+2)}^{2}}}$
$\therefore \int{\sqrt{1-4x-{{x}^{2}}}dx}=\int{\sqrt{{{(\sqrt{5})}^{2}}-{{(x+2)}^{2}}}dx}$
It is known that – $\int{\sqrt{{{a}^{2}}-{{x}^{2}}}dx=\dfrac{x}{2}\sqrt{{{a}^{2}}-{{x}^{2}}}+\dfrac{{{a}^{2}}}{2}{{\sin }^{-1}}\dfrac{x}{a}+C}$
$\Rightarrow \int{\sqrt{{{(\sqrt{5})}^{2}}-{{(x+2)}^{2}}}dx}=\dfrac{x+2}{2}\sqrt{{{(\sqrt{5})}^{2}}-{{(x+2)}^{2}}}+\dfrac{{{(\sqrt{5})}^{2}}}{2}{{\sin }^{-1}}\dfrac{x+2}{\sqrt{5}}+C$$=\dfrac{x+2}{2}\sqrt{1-4x-{{x}^{2}}}+\dfrac{5}{2}{{\sin }^{-1}}\dfrac{x+2}{\sqrt{5}}+C$
Thus, $\int{\sqrt{1-4x-{{x}^{2}}}dx}=\dfrac{x+2}{2}\sqrt{1-4x-{{x}^{2}}}+\dfrac{5}{2}{{\sin }^{-1}}\dfrac{x+2}{\sqrt{5}}+C$
6. Integrate the function - $\sqrt{{{x}^{2}}+4x+5}$
Ans: First simplify –${{x}^{2}}+4x-5$
${{x}^{2}}+4x-5={{x}^{2}}+4x-5+4-4=({{x}^{2}}+4x+4)-5-4$
$=({{x}^{2}}+4x+4)-9={{(x+2)}^{2}}-{{(3)}^{2}}$
$\Rightarrow \sqrt{{{x}^{2}}+4x-5}=\sqrt{{{(x+2)}^{2}}-{{(3)}^{2}}}$
$\therefore \int{\sqrt{{{x}^{2}}+4x-5}dx}=\int{\sqrt{{{(x+2)}^{2}}-{{(3)}^{2}}}dx}$
It is known that – $\int{\sqrt{{{x}^{2}}-{{a}^{2}}}dx=\dfrac{x}{2}\sqrt{{{x}^{2}}-{{a}^{2}}}-\dfrac{{{a}^{2}}}{2}\log \left| x+\sqrt{{{x}^{2}}-{{a}^{2}}} \right|+C}$
$\Rightarrow \int{\sqrt{{{(x+2)}^{2}}-{{(3)}^{2}}}dx}=\dfrac{x+2}{2}\sqrt{{{(x+2)}^{2}}-{{(3)}^{2}}}-\dfrac{{{(3)}^{2}}}{2}\log \left| (x+2)+\sqrt{{{(x+2)}^{2}}-{{(3)}^{2}}} \right|+C$$=\dfrac{x+2}{2}\sqrt{{{x}^{2}}+4x-5}-\dfrac{9}{2}\log \left| (x+2)+\sqrt{{{x}^{2}}+4x-5} \right|+C$
Thus, $\int{\sqrt{{{x}^{2}}+4x-5}dx}=\dfrac{x+2}{2}\sqrt{{{x}^{2}}+4x-5}-\dfrac{9}{2}\log \left| (x+2)+\sqrt{{{x}^{2}}+4x-5} \right|+C$
7. Integrate the function - $\sqrt{1+3x-{{x}^{2}}}$
Ans: First simplify –$1+3x-{{x}^{2}}$
$1+3x-{{x}^{2}}=1-{{x}^{2}}+3x+\dfrac{9}{4}-\dfrac{9}{4}=1+\dfrac{9}{4}-({{x}^{2}}-3x+\dfrac{9}{4})$
$=\dfrac{9+4}{4}-({{x}^{2}}-3x+\dfrac{9}{4})=\left( \dfrac{13}{4} \right)-({{x}^{2}}-3x+\dfrac{9}{4})={{\left( \dfrac{\sqrt{13}}{2} \right)}^{2}}-{{\left( x-\dfrac{3}{2} \right)}^{2}}$
$\Rightarrow \sqrt{1+3x-{{x}^{2}}}=\sqrt{{{\left( \dfrac{\sqrt{13}}{2} \right)}^{2}}-{{\left( x-\dfrac{3}{2} \right)}^{2}}}$
$\therefore \int{\sqrt{1+3x-{{x}^{2}}}dx}=\int{\sqrt{{{\left( \dfrac{\sqrt{13}}{2} \right)}^{2}}-{{\left( x-\dfrac{3}{2} \right)}^{2}}}dx}$
It is known that – $\int{\sqrt{{{a}^{2}}-{{x}^{2}}}dx=\dfrac{x}{2}\sqrt{{{a}^{2}}-{{x}^{2}}}+\dfrac{{{a}^{2}}}{2}{{\sin }^{-1}}\dfrac{x}{a}+C}$
$\Rightarrow \int{\sqrt{{{\left( \dfrac{\sqrt{13}}{2} \right)}^{2}}-{{\left( x-\dfrac{3}{2} \right)}^{2}}}dx}=\dfrac{\left( x-\dfrac{3}{2} \right)}{2}\sqrt{{{\left( \dfrac{\sqrt{13}}{2} \right)}^{2}}-{{\left( x-\dfrac{3}{2} \right)}^{2}}}+\dfrac{{{\left( \dfrac{\sqrt{13}}{2} \right)}^{2}}}{2}{{\sin }^{-1}}\dfrac{\left( x-\dfrac{3}{2} \right)}{\left( \dfrac{\sqrt{13}}{2} \right)}+C$$=\dfrac{2x-3}{4}\sqrt{1+3x-{{x}^{2}}}+\dfrac{13}{8}{{\sin }^{-1}}\dfrac{2x-3}{\sqrt{13}}+C$
Thus, $\int{\sqrt{1+3x-{{x}^{2}}}dx}=\dfrac{2x-3}{4}\sqrt{1+3x-{{x}^{2}}}+\dfrac{13}{8}{{\sin }^{-1}}\dfrac{2x-3}{\sqrt{13}}+C$
8. Integrate the function - $\sqrt{{{x}^{2}}+3x}$
Ans: First simplify –${{x}^{2}}+3x$
${{x}^{2}}+x={{x}^{2}}+3x+\dfrac{9}{4}-\dfrac{9}{4}=({{x}^{2}}+3x+\dfrac{9}{4})-\dfrac{9}{4}$
$={{\left( x+\dfrac{3}{2} \right)}^{2}}-\left( \dfrac{9}{4} \right)={{\left( x+\dfrac{3}{2} \right)}^{2}}-{{\left( \dfrac{3}{2} \right)}^{2}}$
$\Rightarrow \sqrt{{{x}^{2}}+3x}=\sqrt{{{\left( x+\dfrac{3}{2} \right)}^{2}}-{{\left( \dfrac{3}{2} \right)}^{2}}}$
$\therefore \int{\sqrt{{{x}^{2}}+3x}dx}=\int{\sqrt{{{\left( x+\dfrac{3}{2} \right)}^{2}}-{{\left( \dfrac{3}{2} \right)}^{2}}}dx}$
It is known that – $\int{\sqrt{{{x}^{2}}-{{a}^{2}}}dx=\dfrac{x}{2}\sqrt{{{x}^{2}}-{{a}^{2}}}-\dfrac{{{a}^{2}}}{2}\log \left| x+\sqrt{{{x}^{2}}-{{a}^{2}}} \right|+C}$
$\Rightarrow \int{\sqrt{{{\left( x+\dfrac{3}{2} \right)}^{2}}-{{\left( \dfrac{3}{2} \right)}^{2}}}dx}=\dfrac{\left( x+\dfrac{3}{2} \right)}{2}\sqrt{{{\left( x+\dfrac{3}{2} \right)}^{2}}-{{\left( \dfrac{3}{2} \right)}^{2}}}-\dfrac{{{\left( \dfrac{3}{2} \right)}^{2}}}{2}\log \left| \left( x+\dfrac{3}{2} \right)+\sqrt{{{\left( x+\dfrac{3}{2} \right)}^{2}}-{{\left( \dfrac{3}{2} \right)}^{2}}} \right|+C$$=\dfrac{2x+3}{4}\sqrt{{{x}^{2}}+3x}-\dfrac{9}{8}\log \left| \left( x+\dfrac{3}{2} \right)+\sqrt{{{x}^{2}}+3x} \right|+C$
Thus, $\int{\sqrt{{{x}^{2}}+3x}dx}=\dfrac{2x+3}{4}\sqrt{{{x}^{2}}+3x}-\dfrac{9}{8}\log \left| \left( x+\dfrac{3}{2} \right)+\sqrt{{{x}^{2}}+3x} \right|+C$
9. Integrate the function - $\sqrt{1+\dfrac{{{x}^{2}}}{9}}$
Ans: First simplify –$1+\dfrac{{{x}^{2}}}{9}$
$1+\dfrac{{{x}^{2}}}{9}=\dfrac{1}{9}(9+{{x}^{2}})=\dfrac{1}{9}({{3}^{2}}+{{x}^{2}})$
$\Rightarrow \sqrt{1+\dfrac{{{x}^{2}}}{9}}=\sqrt{\dfrac{1}{9}({{3}^{2}}+{{x}^{2}})}=\dfrac{1}{3}\sqrt{({{3}^{2}}+{{x}^{2}})}$
$\therefore \int{\sqrt{1+\dfrac{{{x}^{2}}}{9}}dx}=\int{\dfrac{1}{3}\sqrt{({{3}^{2}}+{{x}^{2}})}dx}=\dfrac{1}{3}\int{\sqrt{({{3}^{2}}+{{x}^{2}})}dx}$
It is known that – $\int{\sqrt{{{x}^{2}}+{{a}^{2}}}dx=\dfrac{x}{2}\sqrt{{{x}^{2}}+{{a}^{2}}}+\dfrac{{{a}^{2}}}{2}\log \left| x+\sqrt{{{x}^{2}}+{{a}^{2}}} \right|+C}$
$\Rightarrow \dfrac{1}{3}\int{\sqrt{({{3}^{2}}+{{x}^{2}})}dx}=\dfrac{1}{3}\left\{ \dfrac{x}{2}\sqrt{{{(x)}^{2}}+{{(3)}^{2}}}+\dfrac{{{(3)}^{2}}}{2}\log \left| (x)+\sqrt{{{(x)}^{2}}+{{(3)}^{2}}} \right| \right\}+C$$=\dfrac{1}{3}\left\{ \dfrac{x}{2}\sqrt{{{x}^{2}}+9}+\dfrac{9}{2}\log \left| x+\sqrt{{{x}^{2}}+9} \right| \right\}+C$
$\dfrac{x}{6}{\sqrt{x^2+9}}+\dfrac{3}{2}\log \left| x+\sqrt{{{x}^{2}}+9} \right| +C$
Thus, $\int{\sqrt{1+\dfrac{{{x}^{2}}}{9}}dx}=\dfrac{x}{6}\sqrt{{{x}^{2}}+9}+\dfrac{3}{2}\log \left| x+\sqrt{{{x}^{2}}+9} \right|+C$
10. Choose the correct answer: $\int{\sqrt{1+{{x}^{2}}}dx}$ is equal to –
$\dfrac{x}{2}\sqrt{1+{{x}^{2}}}+\dfrac{1}{2}\log \left| (x+\sqrt{1+{{x}^{2}}}) \right|+C$
$\dfrac{2}{3}{{(1+{{x}^{2}})}^{\dfrac{3}{2}}}+C$
$\dfrac{2}{3}x{{(1+{{x}^{2}})}^{\dfrac{3}{2}}}+C$
$\dfrac{{{x}^{2}}}{2}\sqrt{1+{{x}^{2}}}+\dfrac{1}{2}{{x}^{2}}\log \left| (x+\sqrt{1+{{x}^{2}}}) \right|+C$
Ans: It is known that – $\int{\sqrt{{{x}^{2}}+{{a}^{2}}}dx=\dfrac{x}{2}\sqrt{{{x}^{2}}+{{a}^{2}}}+\dfrac{{{a}^{2}}}{2}\log \left| x+\sqrt{{{x}^{2}}+{{a}^{2}}} \right|+C}$
Thus, $\int{\sqrt{{{x}^{2}}+{{1}^{2}}}dx=\dfrac{x}{2}\sqrt{{{x}^{2}}+{{1}^{2}}}+\dfrac{{{1}^{2}}}{2}\log \left| x+\sqrt{{{x}^{2}}+{{1}^{2}}} \right|+C}$
$\int{\sqrt{{{x}^{2}}+1}dx=\dfrac{x}{2}\sqrt{{{x}^{2}}+1}+\dfrac{1}{2}\log \left| x+\sqrt{{{x}^{2}}+1} \right|+C}$
The correct answer is option A.
11. Choose the correct answer: $\int{\sqrt{{{x}^{2}}-8x+7}dx}$ is equal to –
$\dfrac{1}{2}(x-4)\sqrt{{{x}^{2}}-8x+7}+9\log \left| (x-4+\sqrt{{{x}^{2}}-8x+7}) \right|+C$
$\dfrac{1}{2}(x+4)\sqrt{{{x}^{2}}-8x+7}+9\log \left| (x+4+\sqrt{{{x}^{2}}-8x+7}) \right|+C$
$\dfrac{1}{2}(x-4)\sqrt{{{x}^{2}}-8x+7}-3\sqrt{2}\log \left| (x-4+\sqrt{{{x}^{2}}-8x+7}) \right|+C$
$\dfrac{1}{2}(x-4)\sqrt{{{x}^{2}}-8x+7}+\dfrac{9}{2}\log \left| (x-4+\sqrt{{{x}^{2}}-8x+7}) \right|+C$
Ans: First simplify –${{x}^{2}}-8x+7$
${{x}^{2}}-8x+7+9-9={{x}^{2}}-8x+16-9=({{x}^{2}}-8x+16)-9$
$={{(x-4)}^{2}}-{{(3)}^{2}}$
$\Rightarrow \sqrt{{{x}^{2}}-8x+7}=\sqrt{{{(x-4)}^{2}}-{{(3)}^{2}}}$
$\therefore \int{\sqrt{{{x}^{2}}-8x+7}dx}=\int{\sqrt{{{(x-4)}^{2}}-{{(3)}^{2}}}dx}$
It is known that – $\int{\sqrt{{{x}^{2}}-{{a}^{2}}}dx=\dfrac{x}{2}\sqrt{{{x}^{2}}-{{a}^{2}}}-\dfrac{{{a}^{2}}}{2}\log \left| x+\sqrt{{{x}^{2}}-{{a}^{2}}} \right|+C}$
$\Rightarrow \int{\sqrt{{{(x-4)}^{2}}-{{(3)}^{2}}}dx}=\dfrac{(x-4)}{2}\sqrt{{{(x-4)}^{2}}-{{(3)}^{2}}}-\dfrac{{{(3)}^{2}}}{2}\log \left| (x-4)+\sqrt{{{(x-4)}^{2}}-{{(3)}^{2}}} \right|+C$$=\dfrac{x-4}{2}\sqrt{{{x}^{2}}-8x+7}-\dfrac{9}{2}\log \left| (x-4)+\sqrt{{{x}^{2}}-8x+7} \right|+C$
Thus, $\int{\sqrt{{{x}^{2}}-8x+7}dx}=\dfrac{x-4}{2}\sqrt{{{x}^{2}}-8x+7}-\dfrac{9}{2}\log \left| (x-4)+\sqrt{{{x}^{2}}-8x+7} \right|+C$
The correct answer is option D
Exercise 7.8
1. Evaluate the definite integral– $\int\limits_{-1}^{1}{(x+1)dx}$
Ans: The second fundamental theorem of integral calculus states that – $\int\limits_{a}^{b}{f(x)dx}=F(b)-F(a)$
Here, $\int{(x+1)dx}=\dfrac{{{x}^{2}}}{2}+x$
So, $\int\limits_{-1}^{1}{(x+1)dx=}\left[ \dfrac{{{x}^{2}}}{2}+x \right]_{-1}^{1}$
$=\left[ \dfrac{{{1}^{2}}}{2}+1 \right]-\left[ \dfrac{{{(-1)}^{2}}}{2}+(-1) \right]$
$=\left[ \dfrac{1}{2}+1 \right]-\left[ \dfrac{1}{2}-1 \right]$
$=\dfrac{1}{2}+1-\dfrac{1}{2}+1=2$
Thus, $\int\limits_{-1}^{1}{(x+1)dx=}2$
2. Evaluate the definite integral– $\int\limits_{2}^{3}{\dfrac{1}{x}dx}$
Ans: The second fundamental theorem of integral calculus states that – $\int\limits_{a}^{b}{f(x)dx}=F(b)-F(a)$
Here, $\int{\dfrac{1}{x}dx}=\log \left| x \right|$
So, $\int\limits_{2}^{3}{\dfrac{1}{x}dx}=\left[ \log \left| x \right| \right]_{2}^{3}$
$=\left[ \log \left| 3 \right| \right]-\left[ \log \left| 2 \right| \right]$
$=\log \dfrac{3}{2}$
Thus, $\int\limits_{2}^{3}{\dfrac{1}{x}dx}=\log \dfrac{3}{2}$
3. Evaluate the definite integral– $\int\limits_{1}^{2}{(4{{x}^{3}}-5{{x}^{2}}+6x+9)dx}$
Ans: The second fundamental theorem of integral calculus states that – $\int\limits_{a}^{b}{f(x)dx}=F(b)-F(a)$
Here, $\int{(4{{x}^{3}}-5{{x}^{2}}+6x+9)dx}=4\left( \dfrac{{{x}^{4}}}{4} \right)-5\left( \dfrac{{{x}^{3}}}{3} \right)+6\left( \dfrac{{{x}^{2}}}{2} \right)+9x$
$={{x}^{4}}-\dfrac{5{{x}^{3}}}{3}+3{{x}^{2}}+9x$
So, $\int\limits_{1}^{2}{(4{{x}^{3}}-5{{x}^{2}}+6x+9)dx}=\left[ {{x}^{4}}-\dfrac{5{{x}^{3}}}{3}+3{{x}^{2}}+9x \right]_{1}^{2}$
$=\left[ {{2}^{4}}-\dfrac{5{{(2)}^{3}}}{3}+3{{(2)}^{2}}+9(2) \right]-\left[ {{1}^{4}}-\dfrac{5{{(1)}^{3}}}{3}+3{{(1)}^{2}}+9(1) \right]$
$=\left[ 16-\dfrac{40}{3}+12+18 \right]-\left[ 1-\dfrac{5}{3}+3+9 \right]$
$=\left[ 46-\dfrac{40}{3} \right]-\left[ 13-\dfrac{5}{3} \right]$
$=46-\dfrac{40}{3}-13+\dfrac{5}{3}$
$=33-\dfrac{35}{3}$
$=\dfrac{99-35}{3}$
$=\dfrac{64}{3}$
Thus, $\int\limits_{1}^{2}{(4{{x}^{3}}-5{{x}^{2}}+6x+9)dx}=\dfrac{64}{3}$
4. Evaluate the definite integral– $\int\limits_{0}^{\dfrac{\pi }{4}}{\sin 2xdx}$
Ans: The second fundamental theorem of integral calculus states that – $\int\limits_{a}^{b}{f(x)dx}=F(b)-F(a)$
Here, $\int{\sin 2xdx}=\dfrac{-\cos 2x}{2}$
So, $\int\limits_{0}^{\dfrac{\pi }{4}}{\sin 2xdx}=\left[ \dfrac{-\cos 2x}{2} \right]_{0}^{\dfrac{\pi }{4}}$
$=\left[ \dfrac{-\cos 2\left( \dfrac{\pi }{4} \right)}{2} \right]-\left[ \dfrac{-\cos 0}{2} \right]$
$=\left[ \dfrac{-\cos \left( \dfrac{\pi }{2} \right)}{2} \right]+\left[ \dfrac{1}{2} \right]$
$=\left[ \dfrac{0}{2} \right]+\left[ \dfrac{1}{2} \right]$
$=\dfrac{1}{2}$
Thus, $\int\limits_{0}^{\dfrac{\pi }{4}}{\sin 2xdx}=\dfrac{1}{2}$
5. Evaluate the definite integral– $\int\limits_{0}^{\dfrac{\pi }{2}}{\cos 2xdx}$
Ans: The second fundamental theorem of integral calculus states that – $\int\limits_{a}^{b}{f(x)dx}=F(b)-F(a)$
Here, $\int{\cos 2xdx}=\dfrac{\sin 2x}{2}$
So, $\int\limits_{0}^{\dfrac{\pi }{2}}{\cos 2xdx}=\left[ \dfrac{\sin 2x}{2} \right]_{0}^{\dfrac{\pi }{2}}$
$=\left[ \dfrac{\sin 2\left( \dfrac{\pi }{2} \right)}{2} \right]-\left[ \dfrac{\sin 0}{2} \right]$
$=\left[ \dfrac{\sin \pi }{2} \right]+\left[ \dfrac{0}{2} \right]$
$=0+0$
$=0$
Thus, $\int\limits_{0}^{\dfrac{\pi }{2}}{\cos 2xdx}=0$
6. Evaluate the definite integral– $\int\limits_{4}^{5}{{{e}^{x}}dx}$
Ans: The second fundamental theorem of integral calculus states that – $\int\limits_{a}^{b}{f(x)dx}=F(b)-F(a)$
Here, $\int{{{e}^{x}}dx}={{e}^{x}}$
So, $\int\limits_{4}^{5}{{{e}^{x}}dx}=\left[ {{e}^{x}} \right]_{4}^{5}$
$=\left[ {{e}^{5}} \right]-\left[ {{e}^{4}} \right]$
$={{e}^{4}}(e-1)$
Thus, $\int\limits_{4}^{5}{{{e}^{x}}dx}={{e}^{4}}(e-1)$
7. $\int\limits_{0}^{\dfrac{\pi }{4}}{\tan xdx}$
Ans: We know that,
$\int{\tan xdx}=-\log \left| \cos x \right|+C$
Therefore, by second fundamental theorem of calculus
$\int{\tan x dx} = \left[ -\log \left| \cos x \right| \right]_{0}^{\dfrac{\pi}{4}}$
$\Rightarrow \int\limits_{0}^{\dfrac{\pi }{4}}{\tan xdx}=\left[ -\log \left| \cos \dfrac{\pi }{4} \right|+\log \left| \cos 0 \right| \right]$
$\Rightarrow \int\limits_{0}^{\dfrac{\pi }{4}}{\tan xdx}=\left[ -\log \left| \dfrac{1}{\sqrt{2}} \right|+\log \left| 1 \right| \right]$
$\therefore \int\limits_{0}^{\dfrac{\pi }{4}}{\tan xdx}=\dfrac{1}{2}\log 2$
8. $\int\limits_{\dfrac{\pi }{6}}^{\dfrac{\pi }{4}}{\cos ecxdx}$
Ans: We know that,
$\int{\cos ecxdx}=\log \left| \cos ecx-\cot x \right|+C$
Therefore, by second fundamental theorem of calculus
$\int\limits_{\dfrac{\pi }{6}}^{\dfrac{\pi }{4}}{\cos ecxdx}=\left[ \log \left| \cos ecx-\cot x \right| \right]_{\dfrac{\pi }{6}}^{\dfrac{\pi }{4}}$
$\Rightarrow \int\limits_{\dfrac{\pi }{6}}^{\dfrac{\pi }{4}}{\cos ecxdx}=\left[ \log \left| \cos ec\dfrac{\pi }{4}-\cot \dfrac{\pi }{4} \right|-\log \left| \cos ec\dfrac{\pi }{6}-\cot \dfrac{\pi }{6} \right| \right]$
$\Rightarrow \int\limits_{\dfrac{\pi }{6}}^{\dfrac{\pi }{4}}{\cos ecxdx}=\left[ \log \left| \sqrt{2}-1 \right|-\log \left| 2-\sqrt{3} \right| \right]$
$\therefore \int\limits_{\dfrac{\pi }{6}}^{\dfrac{\pi }{4}}{\cos ecxdx}=\log \left( \dfrac{\sqrt{2}-1}{2-\sqrt{3}} \right)$
9. $\int\limits_{0}^{1}{\dfrac{1}{\sqrt{1-{{x}^{2}}}}dx}$
Ans: We know that,
$\int{\dfrac{1}{\sqrt{1-{{x}^{2}}}}dx}={{\sin }^{-1}}x+C$
Therefore, by second fundamental theorem of calculus
$\int\limits_{0}^{1}{\dfrac{1}{\sqrt{1-{{x}^{2}}}}dx}=\left[ {{\sin }^{-1}}x \right]_{0}^{1}$
$\Rightarrow \int\limits_{0}^{1}{\dfrac{1}{\sqrt{1-{{x}^{2}}}}dx}=\left[ {{\sin }^{-1}}1-{{\sin }^{-1}}0 \right]$
$\Rightarrow \int\limits_{0}^{1}{\dfrac{1}{\sqrt{1-{{x}^{2}}}}dx}=\left[ \dfrac{\pi }{2}-0 \right]$
$\therefore \int\limits_{0}^{1}{\dfrac{1}{\sqrt{1-{{x}^{2}}}}dx}=\dfrac{\pi }{2}$
10. $\int\limits_{0}^{1}{\dfrac{1}{1+{{x}^{2}}}dx}$
Ans: We know that,
$\int{\dfrac{1}{1+{{x}^{2}}}dx}={{\tan }^{-1}}x+C$
Therefore, by second fundamental theorem of calculus
$\int\limits_{0}^{1}{\dfrac{1}{1+{{x}^{2}}}dx}=\left[ {{\tan }^{-1}}x \right]_{0}^{1}$
$\Rightarrow \int\limits_{0}^{1}{\dfrac{1}{1+{{x}^{2}}}dx}=\left[ {{\tan }^{-1}}1-{{\tan }^{-1}}0 \right]$
$\Rightarrow \int\limits_{0}^{1}{\dfrac{1}{1+{{x}^{2}}}dx}=\left[ \dfrac{\pi }{4}-0 \right]$
$\therefore \int\limits_{0}^{1}{\dfrac{1}{1+{{x}^{2}}}dx}=\dfrac{\pi }{4}$
11. $\int\limits_{2}^{3}{\dfrac{1}{{{x}^{2}}-1}dx}$
Ans: We know that,
$\int{\dfrac{1}{{{x}^{2}}-1}dx}=\dfrac{1}{2}\log \left| \dfrac{x-1}{x+1} \right|+C$
Therefore, by second fundamental theorem of calculus
$\int\limits_{2}^{3}{\dfrac{1}{{{x}^{2}}-1}dx}=\dfrac{1}{2}\left[ \log \left| \dfrac{x-1}{x+1} \right| \right]_{2}^{3}$
$\Rightarrow \int\limits_{2}^{3}{\dfrac{1}{{{x}^{2}}-1}dx}=\dfrac{1}{2}\left[ \log \left| \dfrac{3-1}{3+1} \right|-\log \left| \dfrac{2-1}{2+1} \right| \right]$
$\Rightarrow \int\limits_{2}^{3}{\dfrac{1}{{{x}^{2}}-1}dx}=\dfrac{1}{2}\left[ \log \dfrac{1}{2}-\log \dfrac{1}{3} \right]$
$\therefore \int\limits_{2}^{3}{\dfrac{1}{{{x}^{2}}-1}dx}=\dfrac{1}{2}\log \dfrac{3}{2}$
12. $\int\limits_{0}^{\dfrac{\pi }{4}}{{{\cos }^{2}}xdx}$
Ans: We know that,
$\int{{{\cos }^{2}}xdx}=\int{\left( \dfrac{1+\cos 2x}{2} \right)dx}$
$\Rightarrow \int{{{\cos }^{2}}xdx}=\dfrac{x}{2}+\dfrac{\sin 2x}{4}$
Therefore, by second fundamental theorem of calculus
$\int\limits_{0}^{\dfrac{\pi }{4}}{{{\cos }^{2}}xdx}=\left[ \dfrac{x}{2}+\dfrac{\sin 2x}{4} \right]_{0}^{\dfrac{\pi }{4}}$
$\Rightarrow \int\limits_{0}^{\dfrac{\pi }{4}}{{{\cos }^{2}}xdx}=\dfrac{1}{2}\left[ \dfrac{\pi }{2}-\dfrac{\sin \pi }{2}-0-\dfrac{\sin 0}{2} \right]$
$\Rightarrow \int\limits_{0}^{\dfrac{\pi }{4}}{{{\cos }^{2}}xdx}=\dfrac{1}{2}\left[ \dfrac{\pi }{2}+0-0-0 \right]$
$\therefore \int\limits_{0}^{\dfrac{\pi }{4}}{{{\cos }^{2}}xdx}=\dfrac{\pi }{4}$
13. $\int\limits_{2}^{3}{\dfrac{xdx}{{{x}^{2}}+1}}$
Ans: We know that,
$\int{\dfrac{xdx}{{{x}^{2}}+1}}=\dfrac{1}{2}\int{\left( \dfrac{2x}{{{x}^{2}}+1} \right)dx}$
$\Rightarrow \int{\dfrac{xdx}{{{x}^{2}}+1}}=\dfrac{1}{2}\log \left( 1+{{x}^{2}} \right)$
Therefore, by second fundamental theorem of calculus
$\int\limits_{2}^{3}{\dfrac{xdx}{{{x}^{2}}+1}}=\left[ \log \left( 1+{{3}^{2}} \right)-\log \left( 1+{{2}^{2}} \right) \right]_{2}^{3}$
$\Rightarrow \int\limits_{2}^{3}{\dfrac{xdx}{{{x}^{2}}+1}}=\dfrac{1}{2}\left[ \log 10-\log 5 \right]$
$\Rightarrow \int\limits_{2}^{3}{\dfrac{xdx}{{{x}^{2}}+1}}=\dfrac{1}{2}\log \dfrac{10}{5}$
$\therefore \int\limits_{2}^{3}{\dfrac{xdx}{{{x}^{2}}+1}}=\dfrac{1}{4}\log 2$
14. $\int\limits_{0}^{1}{\dfrac{2x+3}{5{{x}^{2}}+1}dx}$
Ans: Solving $\int{\dfrac{2x+3}{5{{x}^{2}}+1}dx}$ ,
$\int{\dfrac{2x+3}{5{{x}^{2}}+1}dx}=\dfrac{1}{5}\int{\dfrac{5\left( 2x+3 \right)}{5{{x}^{2}}+1}dx}$
$\int{\dfrac{2x+3}{5{{x}^{2}}+1}dx}=\dfrac{1}{5}\int{\dfrac{10x+15}{5{{x}^{2}}+1}dx}$
$\int{\dfrac{2x+3}{5{{x}^{2}}+1}dx}=\dfrac{1}{5}\int{\dfrac{10x}{5{{x}^{2}}+1}dx}+3\int{\dfrac{1}{5{{x}^{2}}+1}dx}$
$\int{\dfrac{2x+3}{5{{x}^{2}}+1}dx}=\dfrac{1}{5}\int{\dfrac{10x}{5{{x}^{2}}+1}dx}+3\int{\dfrac{1}{5\left( {{x}^{2}}+\dfrac{1}{5} \right)}dx}$
$\int{\dfrac{2x+3}{5{{x}^{2}}+1}dx}=\dfrac{1}{5}\log \left( 5{{x}^{2}}+1 \right)+\dfrac{3}{\sqrt{5}}{{\tan }^{-1}}\left( \sqrt{5} \right)x$
Therefore, by second fundamental theorem of calculus
$\int\limits_{0}^{1}{\dfrac{2x+3}{5{{x}^{2}}+1}dx}=\left\{ \dfrac{1}{5}\log \left( 5+1 \right)+\dfrac{3}{\sqrt{5}}{{\tan }^{-1}}\left( \sqrt{5} \right)x \right\}-\left\{ \dfrac{1}{5}\log \left( 1 \right)+\dfrac{3}{\sqrt{5}}{{\tan }^{-1}}0 \right\}$
$\therefore \int\limits_{0}^{1}{\dfrac{2x+3}{5{{x}^{2}}+1}dx}=\dfrac{1}{5}\log 6+\dfrac{3}{\sqrt{5}}{{\tan }^{-1}}\sqrt{5}$
15. $\int\limits_{0}^{1}{x{{e}^{{{x}^{2}}}}dx}$
Ans: Let ${{x}^{2}}=t$ ,
Differentiating it we get,
$2xdx=dt$
Therefore, the integral becomes,
$\dfrac{1}{2}\int\limits_{0}^{1}{{{e}^{t}}dt}$
$\dfrac{1}{2}\int\limits_{0}^{1}{{{e}^{t}}dt}=\left[ \dfrac{1}{2}{{e}^{t}} \right]_{0}^{1}$
$\dfrac{1}{2}\int\limits_{0}^{1}{{{e}^{t}}dt}=\dfrac{1}{2}e-\dfrac{1}{2}{{e}^{0}}$
$\dfrac{1}{2}\left( e-1 \right)$
16. $\int\limits_{1}^{2}{\dfrac{5{{x}^{2}}}{{{x}^{2}}+4x+3}dx}$
Ans: The given integral can be written as
$\int\limits_{1}^{2}{\dfrac{5{{x}^{2}}}{{{x}^{2}}+4x+3}dx}=\int\limits_{1}^{2}{\left\{ 5-\dfrac{20x+15}{{{x}^{2}}+4x+3} \right\}dx}$
$\int\limits_{1}^{2}{\dfrac{5{{x}^{2}}}{{{x}^{2}}+4x+3}dx}=\left[ 5x \right]_{1}^{2}-\int\limits_{1}^{2}{\dfrac{20x+15}{{{x}^{2}}+4x+3}dx}$ …(1)
Solving $\int\limits_{1}^{2}{\dfrac{20x+15}{{{x}^{2}}+4x+3}dx}$ ,
Let $20x+15=A\dfrac{d}{dx}\left( {{x}^{2}}+4x+3 \right)+B$
Equating the coefficients of $x$ and constant term we get,
$A=10,B=-25$
Let ${{x}^{2}}+4x+3=t$
Differentiating it we get,
$\left( 2x+4 \right)dx=dt$
Therefore, the integral becomes
$10\int{\dfrac{dt}{t}-25\int{\dfrac{dx}{{{\left( x+2 \right)}^{2}}-{{1}^{2}}}}}$
$10\int{\dfrac{dt}{t}-25\int{\dfrac{dx}{{{\left( x+2 \right)}^{2}}-{{1}^{2}}}}}=10\log t-25\left[ \dfrac{1}{2}\log \left( \dfrac{x+2-1}{x+2+1} \right) \right]$
$\Rightarrow \int\limits_{1}^{2}{\dfrac{20x+15}{{{x}^{2}}+4x+3}dx}=\left[ 10\log \left( {{x}^{2}}+4x+3 \right)-25\left[ \dfrac{1}{2}\log \left( \dfrac{x+1}{x+3} \right) \right] \right]_{1}^{2}$
$\Rightarrow \int\limits_{1}^{2}{\dfrac{20x+15}{{{x}^{2}}+4x+3}dx}=10\log 15-10\log 8-25\left[ \dfrac{1}{2}\log \dfrac{3}{5}-\dfrac{1}{2}\log \dfrac{2}{4} \right]$
$\Rightarrow \int\limits_{1}^{2}{\dfrac{20x+15}{{{x}^{2}}+4x+3}dx}=10\log 5+10\log 3-10\log 4-10\log 2-\dfrac{25}{2}\left[ \log 3-\log 5-\log 2+\log 4 \right]$
$\Rightarrow \int\limits_{1}^{2}{\dfrac{20x+15}{{{x}^{2}}+4x+3}dx}=\dfrac{45}{2}\log 5-\dfrac{45}{2}\log 4-\dfrac{5}{2}\log 3+\dfrac{5}{2}\log 2$
$\therefore \int\limits_{1}^{2}{\dfrac{20x+15}{{{x}^{2}}+4x+3}dx}=\dfrac{45}{2}\log \dfrac{5}{4}-\dfrac{5}{2}\log \dfrac{3}{2}$
Substituting it in (1) we get,
$\int\limits_{1}^{2}{\dfrac{5{{x}^{2}}}{{{x}^{2}}+4x+3}dx}=5-\left[ \dfrac{45}{2}\log \dfrac{5}{4}-\dfrac{5}{2}\log \dfrac{3}{2} \right]$
$\therefore \int\limits_{1}^{2}{\dfrac{5{{x}^{2}}}{{{x}^{2}}+4x+3}dx}=5-\dfrac{5}{2}\left[ 9\log \dfrac{5}{4}-\log \dfrac{3}{2} \right]$
17. $\int\limits_{0}^{\dfrac{\pi }{4}}{\left( 2{{\sec }^{2}}x+{{x}^{3}}+2 \right)dx}$
Ans: We know that,
$\int{\left( 2{{\sec }^{2}}x+{{x}^{3}}+2 \right)dx}=2\tan x+\dfrac{{{x}^{4}}}{4}+2x$
Therefore, by second fundamental theorem of calculus
$\int{\left( 2{{\sec }^{2}}x+{{x}^{3}}+2 \right)dx}=\left[ 2\tan x+\dfrac{{{x}^{4}}}{4}+2x \right]_{0}^{\dfrac{\pi }{4}}$
$\Rightarrow \int{\left( 2{{\sec }^{2}}x+{{x}^{3}}+2 \right)dx}=\left[ 2\tan \dfrac{\pi }{4}+\dfrac{1}{4}{{\left( \dfrac{\pi }{4} \right)}^{2}}+2\left( \dfrac{\pi }{4} \right)-\left( 2\tan 0+0+0 \right) \right]$
$\Rightarrow \int{\left( 2{{\sec }^{2}}x+{{x}^{3}}+2 \right)dx}=2\tan \dfrac{\pi }{4}+\dfrac{{{\pi }^{4}}}{{{4}^{5}}}+\dfrac{\pi }{2}$
$\therefore \int{\left( 2{{\sec }^{2}}x+{{x}^{3}}+2 \right)dx}=2+\dfrac{\pi }{2}+\dfrac{{{\pi }^{4}}}{1024}$
18. $\int\limits_{0}^{\pi }{\left( {{\sin }^{2}}\dfrac{x}{2}-{{\cos }^{2}}\dfrac{x}{2} \right)dx}$
Ans: We know that,
$\int\limits_{0}^{\pi }{\left( {{\sin }^{2}}\dfrac{x}{2}-{{\cos }^{2}}\dfrac{x}{2} \right)dx}=-\int\limits_{0}^{\pi }{\left( {{\cos }^{2}}\dfrac{x}{2}-{{\sin }^{2}}\dfrac{x}{2} \right)dx}$
$\Rightarrow -\int\limits_{0}^{\pi }{\left( {{\cos }^{2}}\dfrac{x}{2}-{{\sin }^{2}}\dfrac{x}{2} \right)dx}=-\int\limits_{0}^{\pi }{\cos xdx}$
$\int{\cos xdx}=\sin x+C$
Therefore, by second fundamental theorem of calculus
$\int\limits_{0}^{\pi }{\cos xdx}=\sin \pi -sin0$
$\therefore \int\limits_{0}^{\pi }{\cos xdx}=0$
19. $\int\limits_{0}^{2}{\dfrac{6x+3}{{{x}^{2}}+4}dx}$
Ans: Solving the integral we get,
$\int{\dfrac{6x+3}{{{x}^{2}}+4}dx}=3\int{\dfrac{2x+1}{{{x}^{2}}+4}dx}$
$\int{\dfrac{6x+3}{{{x}^{2}}+4}dx}=3\int{\dfrac{2x}{{{x}^{2}}+4}dx}+3\int{\dfrac{1}{{{x}^{2}}+4}dx}$
$\therefore \int{\dfrac{6x+3}{{{x}^{2}}+4}dx}=3\log \left( {{x}^{2}}+4 \right)+\dfrac{3}{2}{{\tan }^{-1}}\dfrac{x}{2}$
Therefore, by second fundamental theorem of calculus
$\int\limits_{0}^{2}{\dfrac{6x+3}{{{x}^{2}}+4}dx}=\left[ 3\log \left( {{x}^{2}}+4 \right)+\dfrac{3}{2}{{\tan }^{-1}}\dfrac{x}{2} \right]_{0}^{2}$
$\Rightarrow \int\limits_{0}^{2}{\dfrac{6x+3}{{{x}^{2}}+4}dx}=\left[ 3\log \left( {{2}^{2}}+4 \right)+\dfrac{3}{2}{{\tan }^{-1}}\dfrac{2}{2}-3\log \left( {{0}^{2}}+4 \right)-\dfrac{3}{2}{{\tan }^{-1}}\dfrac{0}{2} \right]$
$\Rightarrow \int\limits_{0}^{2}{\dfrac{6x+3}{{{x}^{2}}+4}dx}=3\log 8+\dfrac{3}{2}{{\tan }^{-1}}1-3\log 4-\dfrac{3}{2}{{\tan }^{-1}}0$
$\Rightarrow \int\limits_{0}^{2}{\dfrac{6x+3}{{{x}^{2}}+4}dx}=3\log 8+\dfrac{3}{2}\left( \dfrac{\pi }{4} \right)-3\log 4-0$
$\Rightarrow \int\limits_{0}^{2}{\dfrac{6x+3}{{{x}^{2}}+4}dx}=3\log \dfrac{8}{4}+\dfrac{3\pi }{8}$
$\therefore \int\limits_{0}^{2}{\dfrac{6x+3}{{{x}^{2}}+4}dx}=3\log 2+\dfrac{3\pi }{8}$
20. $\int\limits_{0}^{1}{\left( x{{e}^{x}}+\sin \dfrac{\pi x}{4} \right)dx}$
Ans: Solving the integral we get,
$\int\limits_{0}^{1}{\left( x{{e}^{x}}+\sin \dfrac{\pi x}{4} \right)dx}=x\int{{{e}^{x}}dx}-\int{\left\{ \left( \dfrac{d}{dx}x \right)\int{{{e}^{x}}dx} \right\}dx}+\left\{ \dfrac{-\cos \dfrac{\pi x}{4}}{\dfrac{\pi }{4}} \right\}$
$\int\limits_{0}^{1}{\left( x{{e}^{x}}+\sin \dfrac{\pi x}{4} \right)dx}=x{{e}^{x}}-\int{{{e}^{x}}dx}-\dfrac{4}{\pi }\cos \dfrac{x}{4}$
$\therefore \int\limits_{0}^{1}{\left( x{{e}^{x}}+\sin \dfrac{\pi x}{4} \right)dx}=x{{e}^{x}}-{{e}^{x}}-\dfrac{4}{\pi }\cos \dfrac{x}{4}$
Therefore, by second fundamental theorem of calculus
$\int\limits_{0}^{1}{\left( x{{e}^{x}}+\sin \dfrac{\pi x}{4} \right)dx}=\left( 1{{e}^{1}}-{{e}^{1}}-\dfrac{4}{\pi }\cos \dfrac{\pi }{4} \right)-\left( 0{{e}^{0}}-{{e}^{0}}-\dfrac{4}{\pi }\cos 0 \right)$
$\therefore \int\limits_{0}^{1}{\left( x{{e}^{x}}+\sin \dfrac{\pi x}{4} \right)dx}=\left( 1+\dfrac{4}{\pi }-\dfrac{2\sqrt{2}}{\pi } \right)$
21. $\int\limits_{1}^{\sqrt{3}}{\dfrac{1}{1+{{x}^{2}}}dx}$
$\dfrac{\pi }{3}$
$\dfrac{2\pi }{3}$
$\dfrac{\pi }{6}$
$\dfrac{\pi }{12}$
Ans: Solving the integral we get,
$\int\limits_{1}^{\sqrt{3}}{\dfrac{1}{1+{{x}^{2}}}dx}={{\tan }^{-1}}x$
Therefore, by second fundamental theorem of calculus
$\int\limits_{1}^{\sqrt{3}}{\dfrac{1}{1+{{x}^{2}}}dx}={{\tan }^{-1}}\sqrt{3}-{{\tan }^{-1}}1$
$\Rightarrow \int\limits_{1}^{\sqrt{3}}{\dfrac{1}{1+{{x}^{2}}}dx}=\dfrac{\pi }{3}-\dfrac{\pi }{4}$
$\therefore \int\limits_{1}^{\sqrt{3}}{\dfrac{1}{1+{{x}^{2}}}dx}=\dfrac{\pi }{12}$
Thus, the correct option is (D)
22. $\int\limits_{0}^{\dfrac{2}{3}}{\dfrac{1}{4+9{{x}^{2}}}dx}$
$\dfrac{\pi }{6}$
$\dfrac{\pi }{12}$
$\dfrac{\pi }{24}$
$\dfrac{\pi }{4}$
Ans: Solving the integral we get,
$\int{\dfrac{1}{4+9{{x}^{2}}}dx}=\int{\dfrac{1}{{{2}^{2}}+{{\left( 3x \right)}^{2}}}dx}$
Let $3x=t$ ,
Differentiating it we get,
$3dx=dt$
$\therefore \int{\dfrac{1}{{{2}^{2}}+{{\left( 3x \right)}^{2}}}dx}=\dfrac{1}{6}{{\tan }^{-1}}\left( \dfrac{3x}{2} \right)$
Therefore, by second fundamental theorem of calculus
$\int\limits_{0}^{\dfrac{2}{3}}{\dfrac{1}{4+9{{x}^{2}}}dx}=\dfrac{1}{6}{{\tan }^{-1}}\left( \dfrac{3}{2}\cdot \dfrac{2}{3} \right)-\dfrac{1}{6}{{\tan }^{-1}}0$
$\int\limits_{0}^{\dfrac{2}{3}}{\dfrac{1}{4+9{{x}^{2}}}dx}=\dfrac{1}{6}\cdot \dfrac{\pi }{4}$
$\therefore \int\limits_{0}^{\dfrac{2}{3}}{\dfrac{1}{4+9{{x}^{2}}}dx}=\dfrac{\pi }{24}$
Thus, the correct answer is (C).
Exercise 7.9
Solve the following integrals.
1. $\int\limits_{0}^{1}{\dfrac{x}{{{x}^{2}}+1}dx}$
Ans: Let ${{x}^{2}}+1$
Differentiating $2xdx=dt$ ,
Therefore, the integral becomes
$\int\limits_{0}^{1}{\dfrac{x}{{{x}^{2}}+1}dx}=\dfrac{1}{2}\int\limits_{1}^{2}{\dfrac{dt}{t}}$
$\Rightarrow \int\limits_{0}^{1}{\dfrac{x}{{{x}^{2}}+1}dx}=\dfrac{1}{2}\left[ \log \left| t \right| \right]_{1}^{2}$
$\Rightarrow \int\limits_{0}^{1}{\dfrac{x}{{{x}^{2}}+1}dx}=\dfrac{1}{2}\left[ \log 2-\log 1 \right]$
$\Rightarrow \int\limits_{0}^{1}{\dfrac{x}{{{x}^{2}}+1}dx}=\dfrac{1}{2}\log 2$
2. $\int\limits_{0}^{\dfrac{\pi }{2}}{\sqrt{\sin \phi }{{\cos }^{5}}\phi d\phi }$
Ans: The integral can be written as:
$\int\limits_{0}^{\dfrac{\pi }{2}}{\sqrt{\sin \phi }{{\cos }^{5}}\phi d\phi }=\int\limits_{0}^{\dfrac{\pi }{2}}{\sqrt{\sin \phi }{{\cos }^{4}}\phi \cos \phi d\phi }$
$\Rightarrow \int\limits_{0}^{\dfrac{\pi }{2}}{\sqrt{\sin \phi }{{\cos }^{5}}\phi d\phi }=\dfrac{64}{231}$
Let $\sin \phi =t$
Differentiating it we get,
$\cos \phi d\phi =dt$
Therefore, the integral becomes
$\int\limits_{0}^{\dfrac{\pi }{2}}{\sqrt{\sin \phi }{{\cos }^{5}}\phi d\phi }=\int\limits_{0}^{1}{\sqrt{t}{{\left( 1-{{t}^{2}} \right)}^{2}}dt}$
$\Rightarrow \int\limits_{0}^{\dfrac{\pi }{2}}{\sqrt{\sin \phi }{{\cos }^{5}}\phi d\phi }=\int\limits_{0}^{1}{\sqrt{t}\left( 1+{{t}^{4}}-2{{t}^{2}} \right)dt}$
$\Rightarrow \int\limits_{0}^{\dfrac{\pi }{2}}{\sqrt{\sin \phi }{{\cos }^{5}}\phi d\phi }=\int\limits_{0}^{1}{\left( {{t}^{\dfrac{1}{2}}}+{{t}^{\dfrac{9}{2}}}-2{{t}^{\dfrac{5}{2}}} \right)dt}$
$\Rightarrow \int\limits_{0}^{\dfrac{\pi }{2}}{\sqrt{\sin \phi }{{\cos }^{5}}\phi d\phi }=\left[ \dfrac{{{t}^{\dfrac{3}{2}}}}{\dfrac{3}{2}}+\dfrac{{{t}^{\dfrac{11}{2}}}}{\dfrac{11}{2}}-\dfrac{2{{t}^{\dfrac{7}{2}}}}{\dfrac{7}{2}} \right]_{0}^{1}$
$\Rightarrow \int\limits_{0}^{\dfrac{\pi }{2}}{\sqrt{\sin \phi }{{\cos }^{5}}\phi d\phi }=\dfrac{2}{3}+\dfrac{2}{11}-\dfrac{4}{7}$
$\Rightarrow \int\limits_{0}^{\dfrac{\pi }{2}}{\sqrt{\sin \phi }{{\cos }^{5}}\phi d\phi }=\dfrac{154+42-132}{231}$
$\therefore \int\limits_{0}^{\dfrac{\pi }{2}}{\sqrt{\sin \phi }{{\cos }^{5}}\phi d\phi }=\dfrac{64}{231}$
3. $\int\limits_{0}^{1}{{{\sin }^{-1}}\left( \dfrac{2x}{1+{{x}^{2}}} \right)dx}$
Ans: Let $x=\tan \theta $
Differentiating it we get,
$dx={{\sec }^{2}}\theta d\theta $
Therefore, the integral becomes
$\int\limits_{0}^{1}{{{\sin }^{-1}}\left( \dfrac{2x}{1+{{x}^{2}}} \right)dx}=\int\limits_{0}^{\dfrac{\pi }{4}}{{{\sin }^{-1}}\left( \dfrac{2\tan \theta }{1+{{\left( \tan \theta \right)}^{2}}} \right){{\sec }^{2}}\theta d\theta }$
$\int\limits_{0}^{1}{{{\sin }^{-1}}\left( \dfrac{2x}{1+{{x}^{2}}} \right)dx}=\int\limits_{0}^{\dfrac{\pi }{4}}{{{\sin }^{-1}}\left( \sin 2\theta \right){{\sec }^{2}}\theta d\theta }$
$\int\limits_{0}^{1}{{{\sin }^{-1}}\left( \dfrac{2x}{1+{{x}^{2}}} \right)dx}=2\int\limits_{0}^{\dfrac{\pi }{4}}{\theta {{\sec }^{2}}\theta d\theta }$
Let $u=\theta $
And $v={{\sec }^{2}}\theta $
Using integration by parts we get,
$\int\limits_{0}^{1}{{{\sin }^{-1}}\left( \dfrac{2x}{1+{{x}^{2}}} \right)dx}=2\left[ \theta \int{{{\sec }^{2}}\theta d\theta }-\int{\left\{ \left( \dfrac{d}{d\theta }\theta \right)\int{{{\sec }^{2}}\theta d\theta } \right\}d\theta } \right]_{0}^{\dfrac{\pi }{4}}$
$\Rightarrow \int\limits_{0}^{1}{{{\sin }^{-1}}\left( \dfrac{2x}{1+{{x}^{2}}} \right)dx}=2\left[ \theta \tan \theta -\int{\tan \theta d\theta } \right]_{0}^{\dfrac{\pi }{4}}$
$\Rightarrow \int\limits_{0}^{1}{{{\sin }^{-1}}\left( \dfrac{2x}{1+{{x}^{2}}} \right)dx}=2\left[ \theta \tan \theta -\log \left| \cos \theta \right| \right]_{0}^{\dfrac{\pi }{4}}$
$\Rightarrow \int\limits_{0}^{1}{{{\sin }^{-1}}\left( \dfrac{2x}{1+{{x}^{2}}} \right)dx}=2\left[ \dfrac{\pi }{4}\tan \dfrac{\pi }{4}-\log \left| \cos \dfrac{\pi }{4} \right|-\log \left| \cos 0 \right| \right]$
$\Rightarrow \int\limits_{0}^{1}{{{\sin }^{-1}}\left( \dfrac{2x}{1+{{x}^{2}}} \right)dx}=2\left[ \dfrac{\pi }{4}-\log \left| \dfrac{1}{\sqrt{2}} \right|-\log 1 \right]$
$\therefore \int\limits_{0}^{1}{{{\sin }^{-1}}\left( \dfrac{2x}{1+{{x}^{2}}} \right)dx}=\dfrac{\pi }{2}-\log 2$
4. $\int\limits_{0}^{2}{x\sqrt{x+2}dx}$
(Put $x+2={{t}^{2}}$)
Ans: Let $x+2={{t}^{2}}$
Differentiating it, $dx=2tdt$
Therefore, the integral becomes
$\int\limits_{0}^{2}{x\sqrt{x+2}dx}=\int\limits_{\sqrt{2}}^{2}{2{{t}^{2}}\left( {{t}^{2}}-2 \right)dt}$
$\Rightarrow \int\limits_{0}^{2}{x\sqrt{x+2}dx}=2\left[ \dfrac{{{t}^{5}}}{5}-\dfrac{2{{t}^{3}}}{3} \right]_{\sqrt{2}}^{2}$
$\Rightarrow \int\limits_{0}^{2}{x\sqrt{x+2}dx}=2\left[ \dfrac{35}{5}-\dfrac{16}{3}-\dfrac{4\sqrt{2}}{5}+\dfrac{4\sqrt{2}}{3} \right]$
$\therefore \int\limits_{0}^{2}{x\sqrt{x+2}dx}=\dfrac{16\sqrt{2}\left( \sqrt{2}+1 \right)}{15}$
5. $\int\limits_{0}^{\dfrac{\pi }{2}}{\dfrac{\sin x}{1+{{\cos }^{2}}x}dx}$
Ans: Let $\cos x=t$
Differentiating it, $-\sin xdx=dt$
Therefore, the integral becomes
$\int\limits_{0}^{\dfrac{\pi }{2}}{\dfrac{\sin x}{1+{{\cos }^{2}}x}dx}=-\int\limits_{1}^{0}{\dfrac{dt}{1+{{t}^{2}}}}$
$\int\limits_{0}^{\dfrac{\pi }{2}}{\dfrac{\sin x}{1+{{\cos }^{2}}x}dx}=-\left[ {{\tan }^{-1}}t \right]_{1}^{0}$
$\Rightarrow \int\limits_{0}^{\dfrac{\pi }{2}}{\dfrac{\sin x}{1+{{\cos }^{2}}x}dx}=-\left[ {{\tan }^{-1}}0-{{\tan }^{-1}}1 \right]$
$\therefore \int\limits_{0}^{\dfrac{\pi }{2}}{\dfrac{\sin x}{1+{{\cos }^{2}}x}dx}=\dfrac{\pi }{4}$
6. $\int\limits_{0}^{2}{\dfrac{1}{x+4-{{x}^{2}}}dx}$
Ans: The integral can be written as
$\int\limits_{0}^{2}{\dfrac{1}{x+4-{{x}^{2}}}dx}=\int\limits_{0}^{2}{\dfrac{dx}{-\left( {{\left( x-\dfrac{1}{2} \right)}^{2}}-\dfrac{17}{4} \right)}}$
$\int\limits_{0}^{2}{\dfrac{1}{x+4-{{x}^{2}}}dx}=\int\limits_{0}^{2}{\dfrac{dx}{\left( {{\left( \dfrac{\sqrt{17}}{2} \right)}^{2}}-{{\left( x-\dfrac{1}{2} \right)}^{2}} \right)}}$
Let $x-\dfrac{1}{2}=t$
Differentiating it we get,
$dx=dt$
Therefore, the integral becomes
$\int\limits_{0}^{2}{\dfrac{1}{x+4-{{x}^{2}}}dx}=\int\limits_{\dfrac{-1}{2}}^{\dfrac{3}{2}}{\dfrac{dt}{\left( {{\left( \dfrac{\sqrt{17}}{2} \right)}^{2}}-{{\left( t \right)}^{2}} \right)}}$
$\Rightarrow \int\limits_{\dfrac{-1}{2}}^{\dfrac{3}{2}}{\dfrac{dt}{\left( {{\left( \dfrac{\sqrt{17}}{2} \right)}^{2}}-{{\left( t \right)}^{2}} \right)}}=\left[ \dfrac{1}{2\left( \dfrac{\sqrt{17}}{2} \right)}\log \dfrac{\left( \dfrac{\sqrt{17}}{2} \right)+t}{\left( \dfrac{\sqrt{17}}{2} \right)-t} \right]_{\dfrac{-1}{2}}^{\dfrac{3}{2}}$
$\Rightarrow \int\limits_{\dfrac{-1}{2}}^{\dfrac{3}{2}}{\dfrac{dt}{\left( {{\left( \dfrac{\sqrt{17}}{2} \right)}^{2}}-{{\left( t \right)}^{2}} \right)}}=\dfrac{1}{\sqrt{17}}\left[ \log \dfrac{\left( \dfrac{\sqrt{17}}{2} \right)+\dfrac{3}{2}}{\left( \dfrac{\sqrt{17}}{2} \right)-\dfrac{3}{2}}-\log \dfrac{\left( \dfrac{\sqrt{17}}{2} \right)-\dfrac{1}{2}}{\left( \dfrac{\sqrt{17}}{2} \right)+\dfrac{1}{2}} \right]$
$\Rightarrow \int\limits_{\dfrac{-1}{2}}^{\dfrac{3}{2}}{\dfrac{dt}{\left( {{\left( \dfrac{\sqrt{17}}{2} \right)}^{2}}-{{\left( t \right)}^{2}} \right)}}=\dfrac{1}{\sqrt{17}}\left[ \log \dfrac{\sqrt{17}+3}{\sqrt{17}-3}-\log \dfrac{\sqrt{17}-1}{\sqrt{17}+1} \right]$
$\Rightarrow \int\limits_{\dfrac{-1}{2}}^{\dfrac{3}{2}}{\dfrac{dt}{\left( {{\left( \dfrac{\sqrt{17}}{2} \right)}^{2}}-{{\left( t \right)}^{2}} \right)}}=\dfrac{1}{\sqrt{17}}\left[ \log \dfrac{\sqrt{17}+3}{\sqrt{17}-3}\times \dfrac{\sqrt{17}+1}{\sqrt{17}-1} \right]$
$\Rightarrow \int\limits_{\dfrac{-1}{2}}^{\dfrac{3}{2}}{\dfrac{dt}{\left( {{\left( \dfrac{\sqrt{17}}{2} \right)}^{2}}-{{\left( t \right)}^{2}} \right)}}=\dfrac{1}{\sqrt{17}}\left[ \log \dfrac{25+17+10\sqrt{17}}{8} \right]$
$\therefore \int\limits_{0}^{2}{\dfrac{1}{x+4-{{x}^{2}}}dx}=\dfrac{1}{\sqrt{17}}\left[ \log \dfrac{21+5\sqrt{17}}{4} \right]$
7. $\int\limits_{-1}^{1}{\dfrac{1}{{{x}^{2}}+2x+5}dx}$
Ans: The integral can be written as
$\int\limits_{-1}^{1}{\dfrac{1}{{{x}^{2}}+2x+5}dx}=\int\limits_{-1}^{1}{\dfrac{1}{{{\left( x-1 \right)}^{2}}+{{2}^{2}}}dx}$
Let $x+1=t$
Differentiating it we get,
$dx=dt$
Therefore, the integral becomes
$\int\limits_{-1}^{1}{\dfrac{1}{{{x}^{2}}+2x+5}dx}=\int\limits_{0}^{2}{\dfrac{dt}{{{t}^{2}}+{{2}^{2}}}}$
$\Rightarrow \int\limits_{-1}^{1}{\dfrac{1}{{{x}^{2}}+2x+5}dx}=\left[ \dfrac{1}{2}{{\tan }^{-1}}\dfrac{t}{2} \right]_{0}^{2}$
$\Rightarrow \int\limits_{-1}^{1}{\dfrac{1}{{{x}^{2}}+2x+5}dx}=\left[ \dfrac{1}{2}{{\tan }^{-1}}1-\dfrac{1}{2}{{\tan }^{-1}}0 \right]$
$\therefore \int\limits_{-1}^{1}{\dfrac{1}{{{x}^{2}}+2x+5}dx}=\dfrac{\pi }{8}$
8. $\int\limits_{1}^{2}{\left( \dfrac{1}{x}-\dfrac{1}{2{{x}^{2}}} \right){{e}^{2x}}dx}$
Ans: Let $2x=t$
Differentiating it $2dx=dt$ ,
Therefore, the integral becomes
$\int\limits_{1}^{2}{\left( \dfrac{1}{x}-\dfrac{1}{2{{x}^{2}}} \right){{e}^{2x}}dx}=\dfrac{1}{2}\int\limits_{2}^{1}{\left( \dfrac{2}{t}-\dfrac{2}{{{t}^{2}}} \right){{e}^{t}}dt}$
$\Rightarrow \int\limits_{1}^{2}{\left( \dfrac{1}{x}-\dfrac{1}{2{{x}^{2}}} \right){{e}^{2x}}dx}=\int\limits_{2}^{1}{\left( \dfrac{1}{t}-\dfrac{1}{{{t}^{2}}} \right){{e}^{t}}dt}$
Let $\dfrac{1}{t}=f\left( t \right)$
Then, $f'\left( t \right)=-\dfrac{1}{{{t}^{2}}}$
$\int\limits_{2}^{1}{\left( \dfrac{1}{t}-\dfrac{1}{{{t}^{2}}} \right){{e}^{t}}dt}=\int\limits_{2}^{1}{\left[ f\left( t \right)+f'\left( t \right) \right]{{e}^{t}}dt}$
$\Rightarrow \int\limits_{2}^{1}{\left( \dfrac{1}{t}-\dfrac{1}{{{t}^{2}}} \right){{e}^{t}}dt}=\left[ {{e}^{t}}f\left( t \right) \right]_{2}^{4}$
$\Rightarrow \int\limits_{2}^{1}{\left( \dfrac{1}{t}-\dfrac{1}{{{t}^{2}}} \right){{e}^{t}}dt}=\left[ \dfrac{{{e}^{t}}}{t} \right]_{2}^{4}$
$\Rightarrow \int\limits_{2}^{1}{\left( \dfrac{1}{t}-\dfrac{1}{{{t}^{2}}} \right){{e}^{t}}dt}=\dfrac{{{e}^{4}}}{4}-\dfrac{{{e}^{2}}}{2}$
$\therefore \int\limits_{1}^{2}{\left( \dfrac{1}{x}-\dfrac{1}{2{{x}^{2}}} \right){{e}^{2x}}dx}=\dfrac{{{e}^{2}}\left( {{e}^{2}}-2 \right)}{4}$
9. The value of the integral $\int\limits_{\dfrac{1}{3}}^{1}{\dfrac{{{\left( x-{{x}^{3}} \right)}^{\dfrac{1}{3}}}}{{{x}^{4}}}dx}$
$6$
$0$
$3$
$4$
Ans: Let $x=\sin \theta $
Differentiating it, $dx=\cos \theta d\theta $
Therefore, the integral becomes
$\int\limits_{\dfrac{1}{3}}^{1}{\dfrac{{{\left( x-{{x}^{3}} \right)}^{\dfrac{1}{3}}}}{{{x}^{4}}}dx}=\int\limits_{{{\sin }^{-1}}\dfrac{1}{3}}^{\dfrac{\pi }{2}}{\dfrac{{{\left( \sin \theta -{{\sin }^{3}}\theta \right)}^{\dfrac{1}{3}}}}{{{\sin }^{4}}\theta }\cos \theta d\theta }$
$\Rightarrow \int\limits_{{{\sin }^{-1}}\dfrac{1}{3}}^{\dfrac{\pi }{2}}{\dfrac{{{\left( \sin \theta -{{\sin }^{3}}\theta \right)}^{\dfrac{1}{3}}}}{{{\sin }^{4}}\theta }\cos \theta d\theta }=\int \limits_{{{\sin }^{-1}}\dfrac{1}{3}}^{\dfrac{\pi }{2}}{\dfrac{{{\left( \sin \theta \right)}^{\dfrac{1}{3}}}{{\left( \cos \theta \right)}^{\dfrac{2}{3}}}}{{{\sin }^{4}}\theta }\cos \theta d\theta }$
$\Rightarrow \int\limits_{{{\sin }^{-1}}\dfrac{1}{3}}^{\dfrac{\pi }{2}}{\dfrac{{{\left( \sin \theta -{{\sin }^{3}}\theta \right)}^{\dfrac{1}{3}}}}{{{\sin }^{4}}\theta }\cos \theta d\theta }=\int\limits_{{{\sin }^{-1}}\dfrac{1}{3}}^{\dfrac{\pi }{2}}{\dfrac{{{\left( \cos \theta \right)}^{\dfrac{5}{3}}}}{{{\sin }^{\dfrac{5}{3}}}\theta }\cos e{{c}^{2}}\theta d\theta }$
$\Rightarrow \int\limits_{{{\sin }^{-1}}\dfrac{1}{3}}^{\dfrac{\pi }{2}}{\dfrac{{{\left( \sin \theta -{{\sin }^{3}}\theta \right)}^{\dfrac{1}{3}}}}{{{\sin }^{4}}\theta }\cos \theta d\theta }=\int\limits_{{{\sin }^{-1}}\dfrac{1}{3}}^{\dfrac{\pi }{2}}{{{\left( \cot \theta \right)}^{\dfrac{5}{3}}}\cos e{{c}^{2}}\theta d\theta }$
Let $\cot \theta =t$
Differentiating it, $-\cos e{{c}^{2}}\theta d\theta =dt$
Therefore, the integral becomes
$\int\limits_{\dfrac{1}{3}}^{1}{\dfrac{{{\left( x-{{x}^{3}} \right)}^{\dfrac{1}{3}}}}{{{x}^{4}}}dx}=\int\limits_{2\sqrt{2}}^{0}{{{t}^{\dfrac{5}{3}}}dt}$
$\Rightarrow \int\limits_{\dfrac{1}{3}}^{1}{\dfrac{{{\left( x-{{x}^{3}} \right)}^{\dfrac{1}{3}}}}{{{x}^{4}}}dx}=\dfrac{3}{8}{{\sqrt{8}}^{\dfrac{8}{3}}}$
$\Rightarrow \int\limits_{\dfrac{1}{3}}^{1}{\dfrac{{{\left( x-{{x}^{3}} \right)}^{\dfrac{1}{3}}}}{{{x}^{4}}}dx}=\dfrac{3}{8}\times 16$
$\therefore \int\limits_{\dfrac{1}{3}}^{1}{\dfrac{{{\left( x-{{x}^{3}} \right)}^{\dfrac{1}{3}}}}{{{x}^{4}}}dx}=6$
Thus, the correct answer is A.
10. If $f\left( x \right)=\int\limits_{0}^{x}{t\sin tdt}$ ,then $f'\left( x \right)$ is
$\cos x+x\sin x$
$x\sin x$
$x\cos x$
$\sin x+x\cos x$
Ans: Given $f\left( x \right)=\int\limits_{0}^{x}{t\sin tdt}$
Using Integration by parts, we get
$f\left( x \right)=t\int\limits_{0}^{x}{\sin tdt}-\int\limits_{0}^{x}{\left\{ \left( \dfrac{d}{dt}t \right)\int{\sin tdt} \right\}dt}$
$\Rightarrow f\left( x \right)=\left[ t\left( -\cos t \right) \right]_{0}^{x}-\int\limits_{0}^{x}{-\cot tdt}$
$\Rightarrow f\left( x \right)=\left[ -t\left( \cos t \right)+\sin t \right]_{0}^{x}$
$\Rightarrow f\left( x \right)=-x\cos x+\sin x$
Therefore,
$f'\left( x \right)=-\left[ -x\sin x+\cos x \right]+\cos x$
$\therefore f'\left( x \right)=x\sin x$
Thus, the correct answer is B.
Exercise 7.10
Solve the following integrals.
1. $\int\limits_{0}^{\dfrac{\pi }{2}}{{{\cos }^{2}}xdx}$
Ans: Given $I=\int\limits_{0}^{\dfrac{\pi }{2}}{{{\cos }^{2}}xdx}$ …(1)
We know that,
$\int\limits_{0}^{a}{f\left( x \right)dx}=\int\limits_{0}^{a}{f\left( a-x \right)dx}$
Therefore, the integral becomes
$I=\int\limits_{0}^{\dfrac{\pi }{2}}{{{\cos }^{2}}\left( \dfrac{\pi }{2}-x \right)dx}$
$\Rightarrow I=\int\limits_{0}^{\dfrac{\pi }{2}}{{{\sin }^{2}}xdx}$ …(2)
Adding equation (1) and (2),
$2I=\int\limits_{0}^{\dfrac{\pi }{2}}{\left( {{\sin }^{2}}x+{{\cos }^{2}}x \right)dx}$
$\Rightarrow 2I=\int\limits_{0}^{\dfrac{\pi }{2}}{1dx}$
$\Rightarrow 2I=\left[ x \right]_{0}^{\dfrac{\pi }{2}}$
$\Rightarrow 2I=\dfrac{\pi }{2}$
$\Rightarrow I=\dfrac{\pi }{4}$
$\therefore \int\limits_{0}^{\dfrac{\pi }{2}}{{{\cos }^{2}}xdx}=\dfrac{\pi }{4}$
2. $\int\limits_{0}^{\dfrac{\pi }{2}}{\dfrac{\sqrt{\sin x}}{\sqrt{\sin x}+\sqrt{\cos x}}dx}$
Ans: Given $I=\int\limits_{0}^{\dfrac{\pi }{2}}{\dfrac{\sqrt{\sin x}}{\sqrt{\sin x}+\sqrt{\cos x}}dx}$ …(1)
We know that,
$\int\limits_{0}^{a}{f\left( x \right)dx}=\int\limits_{0}^{a}{f\left( a-x \right)dx}$
Therefore, the integral becomes
$I=\int\limits_{0}^{\dfrac{\pi }{2}}{\dfrac{\sqrt{\sin \left( \dfrac{\pi }{2}-x \right)}}{\sqrt{\sin \left( \dfrac{\pi }{2}-x \right)}+\sqrt{\cos \left( \dfrac{\pi }{2}-x \right)}}dx}$
$\Rightarrow I=\int\limits_{0}^{\dfrac{\pi }{2}}{\dfrac{\sqrt{\cos x}}{\sqrt{\sin x}+\sqrt{\cos x}}dx}$ …(2)
Adding equation (1) and (2),
$\Rightarrow 2I=\int\limits_{0}^{\dfrac{\pi }{2}}{\dfrac{\sqrt{\sin x}+\sqrt{\cos x}}{\sqrt{\sin x}+\sqrt{\cos x}}dx}$
$\Rightarrow 2I=\int\limits_{0}^{\dfrac{\pi }{2}}{1dx}$
$\Rightarrow 2I=\left[ x \right]_{0}^{\dfrac{\pi }{2}}$
$\Rightarrow 2I=\dfrac{\pi }{2}$
$\Rightarrow I=\dfrac{\pi }{4}$
$\therefore \int\limits_{0}^{\dfrac{\pi }{2}}{\dfrac{\sqrt{\sin x}}{\sqrt{\sin x}+\sqrt{\cos x}}dx}=\dfrac{\pi }{4}$
3. $\int\limits_{0}^{\dfrac{\pi }{2}}{\dfrac{{{\sin }^{\dfrac{3}{2}}}xdx}{{{\sin }^{\dfrac{3}{2}}}x+{{\cos }^{\dfrac{3}{2}}}x}}$
Ans: Given $I=\int\limits_{0}^{\dfrac{\pi }{2}}{\dfrac{{{\sin }^{\dfrac{3}{2}}}xdx}{{{\sin }^{\dfrac{3}{2}}}x+{{\cos }^{\dfrac{3}{2}}}x}}$ …(1)
We know that,
$\int\limits_{0}^{a}{f\left( x \right)dx}=\int\limits_{0}^{a}{f\left( a-x \right)dx}$
Therefore, the integral becomes
$I=\int\limits_{0}^{\dfrac{\pi }{2}}{\dfrac{{{\sin }^{\dfrac{3}{2}}}\left( \dfrac{\pi }{2}-x \right)dx}{{{\sin }^{\dfrac{3}{2}}}\left( \dfrac{\pi }{2}-x \right)+{{\cos }^{\dfrac{3}{2}}}\left( \dfrac{\pi }{2}-x \right)}}$
$\Rightarrow I=\int\limits_{0}^{\dfrac{\pi }{2}}{\dfrac{{{\cos }^{\dfrac{3}{2}}}xdx}{{{\sin }^{\dfrac{3}{2}}}x+{{\cos }^{\dfrac{3}{2}}}x}}$ …(2)
Adding equation (1) and (2),
$\Rightarrow 2I=\int\limits_{0}^{\dfrac{\pi }{2}}{\dfrac{{{\sin }^{\dfrac{3}{2}}}x+{{\cos }^{\dfrac{3}{2}}}x}{{{\sin }^{\dfrac{3}{2}}}x+{{\cos }^{\dfrac{3}{2}}}x}dx}$
$\Rightarrow 2I=\int\limits_{0}^{\dfrac{\pi }{2}}{1dx}$
$\Rightarrow 2I=\left[ x \right]_{0}^{\dfrac{\pi }{2}}$
$\Rightarrow 2I=\dfrac{\pi }{2}$
$\Rightarrow I=\dfrac{\pi }{4}$
$\therefore \int\limits_{0}^{\dfrac{\pi }{2}}{\dfrac{{{\sin }^{\dfrac{3}{2}}}xdx}{{{\sin }^{\dfrac{3}{2}}}x+{{\cos }^{\dfrac{3}{2}}}x}}=\dfrac{\pi }{4}$
4. $\int\limits_{0}^{\dfrac{\pi }{2}}{\dfrac{{{\cos }^{5}}xdx}{{{\sin }^{5}}x+{{\cos }^{5}}x}}$
Ans: Given $I=\int\limits_{0}^{\dfrac{\pi }{2}}{\dfrac{{{\cos }^{5}}xdx}{{{\sin }^{5}}x+{{\cos }^{5}}x}}$ …(1)
We know that,
$\int\limits_{0}^{a}{f\left( x \right)dx}=\int\limits_{0}^{a}{f\left( a-x \right)dx}$
Therefore, the integral becomes
$I=\int\limits_{0}^{\dfrac{\pi }{2}}{\dfrac{{{\cos }^{5}}\left( \dfrac{\pi }{2}-x \right)dx}{{{\sin }^{5}}\left( \dfrac{\pi }{2}-x \right)+{{\cos }^{5}}\left( \dfrac{\pi }{2}-x \right)}}$
$\Rightarrow I=\int\limits_{0}^{\dfrac{\pi }{2}}{\dfrac{{{\sin }^{5}}xdx}{{{\sin }^{5}}x+{{\cos }^{5}}x}}$ …(2)
Adding equation (1) and (2),
$\Rightarrow 2I=\int\limits_{0}^{\dfrac{\pi }{2}}{\dfrac{{{\sin }^{5}}x+{{\cos }^{5}}x}{{{\sin }^{5}}x+{{\cos }^{5}}x}dx}$
$\Rightarrow 2I=\int\limits_{0}^{\dfrac{\pi }{2}}{1dx}$
$\Rightarrow 2I=\left[ x \right]_{0}^{\dfrac{\pi }{2}}$
$\Rightarrow 2I=\dfrac{\pi }{2}$
$\Rightarrow I=\dfrac{\pi }{4}$
$\therefore \int\limits_{0}^{\dfrac{\pi }{2}}{\dfrac{{{\cos }^{5}}xdx}{{{\sin }^{5}}x+{{\cos }^{5}}x}}=\dfrac{\pi }{4}$
5. $\int\limits_{-5}^{5}{\left| x+2 \right|}dx$
Ans: Let $I=\int\limits_{-5}^{5}{\left| x+2 \right|}dx$
Since, $\left( x+2 \right)\le 0$ for interval $\left[ -5,-2 \right]$ .
Therefore, $\left( x+2 \right)\ge 0$ for interval $\left[ -2,5 \right]$.
As, $\int\limits_{a}^{b}{f\left( x \right)}dx=\int\limits_{a}^{c}{f\left( x \right)}dx+\int\limits_{c}^{b}{f\left( x \right)dx}$
Hence, $\int\limits_{-5}^{-2}{-\left( x+2 \right)}dx+\int\limits_{-2}^{5}{\left( x+2 \right)dx}$ .
Thus,
$I=\int\limits_{-5}^{-2}{-\left( x+2 \right)}dx+\int\limits_{-2}^{5}{\left( x+2 \right)dx}$
$=-\left[ \dfrac{{{x}^{2}}}{2}+2x \right]_{-5}^{2}+\left[ \dfrac{{{x}^{2}}}{2}+2x \right]_{-2}^{5}$
$=-\left[ \dfrac{{{\left( 2 \right)}^{2}}}{2}+2\left( 2 \right)-\dfrac{{{\left( -5 \right)}^{2}}}{2}-2\left( -5 \right) \right]+\left[ \dfrac{{{\left( 5 \right)}^{2}}}{2}+2\left( 5 \right)-\dfrac{{{\left( -2 \right)}^{2}}}{2}-2\left( -2 \right) \right]$$=-\left[ 2-4-\dfrac{25}{2}+10 \right]+\left[ \dfrac{25}{2}+10-2+4 \right]$
$=-2+4+\dfrac{25}{2}+10+\dfrac{25}{2}+10-2+4$
$=29$
6. $\int\limits_{2}^{8}{\left| x-5 \right|}dx$
Ans: Let $I=\int\limits_{2}^{8}{\left| x-5 \right|}dx$
Since, $\left( x-5 \right)\le 0$ for interval $\left[ 2,5 \right]$ .
Therefore, $\left( x-5 \right)\ge 0$ for interval $\left[ 5,8 \right]$.
As, $\int\limits_{a}^{b}{f\left( x \right)}dx=\int\limits_{a}^{c}{f\left( x \right)}dx+\int\limits_{c}^{b}{f\left( x \right)dx}$
Hence, $\int\limits_{2}^{5}{-\left( x-5 \right)}dx+\int\limits_{5}^{8}{\left( x-5 \right)dx}$ .
Thus,
$I=\int\limits_{2}^{5}{-\left( x-5 \right)}dx+\int\limits_{5}^{8}{\left( x-5 \right)dx}$
$=-\left[ \dfrac{{{x}^{2}}}{2}-5x \right]_{2}^{5}+\left[ \dfrac{{{x}^{2}}}{2}-5x \right]_{5}^{8}$
$=-\left[ \dfrac{{{\left( 5 \right)}^{2}}}{2}-5\left( 5 \right)-\dfrac{{{\left( 2 \right)}^{2}}}{2}+5\left( 2 \right) \right]+\left[ \dfrac{{{\left( 8 \right)}^{2}}}{2}-5\left( 8 \right)-\dfrac{{{\left( 5 \right)}^{2}}}{2}+5\left( 5 \right) \right]$
$=-\left[ \dfrac{25}{2}-25-2+10 \right]+\left[ 32-40-\dfrac{25}{2}+25 \right]$
$=-\dfrac{25}{2}+25+2-10+32-40-\dfrac{25}{2}+25$
$=9$
7. $\int\limits_{0}^{1}{x{{\left( 1-x \right)}^{n}}dx}$
Ans: Let $I=\int\limits_{0}^{1}{x{{\left( 1-x \right)}^{n}}dx}$
Thus, $I=\int\limits_{0}^{1}{\left( 1-x \right){{\left( 1-\left( 1-x \right) \right)}^{n}}dx}$
Since, $\int\limits_{1}^{a}{f\left( x \right)dx}=\int\limits_{0}^{a}{f\left( a-x \right)dx}$.
Therefore,
$\int\limits_{0}^{1}{\left( 1-x \right){{\left( x \right)}^{n}}}dx$
$=\int\limits_{0}^{1}{\left( {{x}^{n}}-{{x}^{n+1}} \right)dx}$
$=\left[ \dfrac{{{x}^{n+1}}}{n+1}-\dfrac{{{x}^{n+2}}}{n+2} \right]_{0}^{1}$
$=\left[ \dfrac{1}{n+1}-\dfrac{1}{n+2} \right]$
$=\dfrac{\left( n+2 \right)-\left( n+1 \right)}{\left( n+1 \right)\left( n+2 \right)}$
$=\dfrac{1}{\left( n+1 \right)\left( n+2 \right)}$
8. $\int\limits_{0}^{\dfrac{\pi }{4}}{\log \left( 1+\tan x \right)dx}$
Ans: Let $I=\int\limits_{0}^{\dfrac{\pi }{4}}{\log \left( 1+\tan x \right)dx}$
Since, $\int\limits_{0}^{a}{f\left( x \right)dx}=\int\limits_{0}^{a}{f\left( a-x \right)dx}$
Therefore, $I=\int\limits_{0}^{\dfrac{\pi }{4}}{\log \left[ 1+\tan \left( \dfrac{\pi }{4}-x \right) \right]dx}$
$=\int\limits_{0}^{\dfrac{\pi }{4}}{\log \left[ 1+\dfrac{\tan \dfrac{\pi }{4}-\tan x}{1+\tan \dfrac{\pi }{4}\tan x} \right]dx}$
$=\int\limits_{0}^{\dfrac{\pi }{4}}{\log \left\{ 1+\dfrac{1-\tan x}{1+\tan x} \right\}dx}$
$=\int\limits_{0}^{\dfrac{\pi }{4}}{\log \dfrac{2}{1+\tan x}}dx$
$=\int\limits_{0}^{\dfrac{\pi }{4}}{\log 2dx}-\int\limits_{0}^{\dfrac{\pi }{4}}{\log \left( 1+\tan x \right)}dx$
$=\int\limits_{0}^{\dfrac{\pi }{4}}{\log 2dx}-I$
$2I=\left[ x\log 2 \right]_{0}^{\dfrac{\pi }{4}}$
$2I=\dfrac{\pi }{4}\log 2$
$I=\dfrac{\pi }{8}\log 2$
9. $\int\limits_{0}^{2}{x\sqrt{2-x}dx}$
Ans: Let $I=\int\limits_{0}^{2}{x\sqrt{2-x}dx}$
Since, $\int\limits_{0}^{a}{f\left( x \right)}dx=\int\limits_{0}^{a}{f\left( a-x \right)}dx$
Therefore, $I=\int\limits_{0}^{2}{\left( 2-x \right)}\sqrt{x}dx$
$=\int\limits_{0}^{2}{\left\{ 2{{x}^{{1}/{2}\;}}-{{x}^{{3}/{2}\;}} \right\}}dx$
$=\left[ 2\left( \dfrac{{{x}^{{3}/{2}\;}}}{{3}/{2}\;} \right)-\dfrac{{{x}^{{5}/{2}\;}}}{{5}/{2}\;} \right]_{0}^{2}$
$=\left[ \dfrac{4}{3}{{x}^{{3}/{2}\;}}-\dfrac{2}{5}{{x}^{{5}/{2}\;}} \right]_{0}^{2}$
$=\dfrac{4}{3}{{\left( 2 \right)}^{{3}/{2}\;}}-\dfrac{2}{5}{{\left( 2 \right)}^{{5}/{2}\;}}$
$=\dfrac{8\sqrt{2}}{3}-\dfrac{8\sqrt{2}}{5}$
$=\dfrac{40\sqrt{2}-24\sqrt{2}}{15}$
$=\dfrac{16\sqrt{2}}{15}$
10. $\int\limits_{0}^{\dfrac{\pi }{2}}{\left( 2\log \sin x-\log \sin 2x \right)dx}$
Ans: Let $I=\int\limits_{0}^{\dfrac{\pi }{2}}{\left( 2\log \sin x-\log \sin 2x \right)dx}$
$\Rightarrow I=\int\limits_{0}^{\dfrac{\pi }{2}}{\left( 2\log \sin x-\log \left( 2\sin x\cos x \right) \right)dx}$
$\Rightarrow I=\int\limits_{0}^{\dfrac{\pi }{2}}{\left( 2\log \sin x-\log \sin x-\log \cos x-\log 2 \right)dx}$
$\Rightarrow I=\int\limits_{0}^{\dfrac{\pi }{2}}{\left( \log \sin x-\log \cos x-\log 2 \right)dx}$ …….(1)
Since,
$\int\limits_{0}^{a}{f\left( x \right)dx}=\int\limits_{0}^{a}{f\left( a-x \right)dx}$
Therefore,
$\Rightarrow I=\int\limits_{0}^{\dfrac{\pi }{2}}{\left( \log \cos x-\log \sin x-\log 2 \right)dx}$ …….(2)
On adding equation 1 and 2-
$2I=\int\limits_{0}^{\dfrac{\pi }{2}}{\left( -\log 2-\log 2 \right)dx}$
$\Rightarrow 2I=-2\log 2\int\limits_{0}^{\dfrac{\pi }{2}}{dx}$
$\Rightarrow I=-\log 2\left[ \dfrac{\pi }{2} \right]$
$\Rightarrow I=-\dfrac{\pi }{2}\left[ \log 2 \right]$
$\Rightarrow I=\dfrac{\pi }{2}\left[ -\log 2 \right]$
$\Rightarrow I=\dfrac{\pi }{2}\left[ \log \dfrac{1}{2} \right]$
$\Rightarrow I=\dfrac{\pi }{2}\log \dfrac{1}{2}$
11. $\int\limits_{\dfrac{-\pi }{2}}^{\dfrac{\pi }{2}}{{{\sin }^{2}}xdx}$
Ans: Let $I=\int\limits_{\dfrac{-\pi }{2}}^{\dfrac{\pi }{2}}{{{\sin }^{2}}xdx}$
Since, ${{\sin }^{2}}x$ is an even function.
Therefore , $\int\limits_{\dfrac{-\pi }{2}}^{\dfrac{\pi }{2}}{{{\sin }^{2}}xdx}=2\int\limits_{0}^{\dfrac{\pi }{2}}{{{\sin }^{2}}xdx}$
As if $f\left( x \right)$ is an even function, then $\int\limits_{-a}^{a}{f\left( x \right)dx}=2\int\limits_{0}^{a}{f\left( x \right)dx}$.
Hence,
$I=2\int\limits_{0}^{\dfrac{\pi }{2}}{{{\sin }^{2}}xdx}$
$=2\int\limits_{0}^{\dfrac{\pi }{2}}{\dfrac{1-\cos 2x}{2}dx}$
$=\int\limits_{0}^{\dfrac{\pi }{2}}{\left( 1-\cos 2x \right)dx}$
$=\left[ x-\dfrac{sin2x}{2} \right]_{0}^{\dfrac{\pi }{2}}$
$=\dfrac{\pi }{2}$
12. $\int\limits_{0}^{\pi }{\dfrac{x}{1+\sin x}dx}$
Ans: Let $I=\int\limits_{0}^{\pi }{\dfrac{x}{1+\sin x}dx}$ …….(1)
Since, $\int\limits_{0}^{a}{f\left( x \right)dx}=\int\limits_{0}^{a}{f\left( a-x \right)dx}$
Therefore, $I=\int\limits_{0}^{\pi }{\dfrac{\left( \pi -x \right)}{1+\sin x\left( \pi -x \right)}dx}$
$I=\int\limits_{0}^{\pi }{\dfrac{\left( \pi -x \right)}{1+\sin x}dx}$ …..(2)
On adding equation 1 and 2-
$2I=\int\limits_{0}^{\pi }{\dfrac{\pi }{1+\sin x}dx}$
$\Rightarrow 2I=\pi \int\limits_{0}^{\pi }{\dfrac{\left( 1-\sin x \right)}{\left( 1+\sin x \right)+\left( 1-\sin x \right)}dx}$
$\Rightarrow 2I=\pi \int\limits_{0}^{\pi }{\dfrac{\left( 1-\sin x \right)}{{{\cos }^{2}}x}dx}$
$\Rightarrow 2I=\pi \int\limits_{0}^{\pi }{\left\{ {{\sec }^{2}}x-\tan x\sec x \right\}dx}$
$\Rightarrow 2I=\pi \left[ 2 \right]$
$\Rightarrow I=\pi $
13. $\int\limits_{\dfrac{-\pi }{2}}^{\dfrac{\pi }{2}}{{{\sin }^{7}}xdx}$.
Ans: Let $I=\int\limits_{\dfrac{-\pi }{2}}^{\dfrac{\pi }{2}}{{{\sin }^{7}}xdx}$
Since, ${{\sin }^{7}}x$ is an even function.
Therefore , $\int\limits_{\dfrac{-\pi }{2}}^{\dfrac{\pi }{2}}{{{\sin }^{2}}xdx}=0$
As if $f\left( x \right)$ is an odd function, then $\int\limits_{-a}^{a}{f\left( x \right)dx}=0$.
Hence, $I=0$
14. $\int\limits_{0}^{2\pi }{{{\cos }^{5}}xdx}$.
Ans: Let
$I=\int\limits_{0}^{2\pi }{{{\cos }^{5}}xdx}$
${{\cos }^{5}}\left( 2\pi -x \right)={{\cos }^{5}}x$ …..(1)
If $f\left( 2a-x \right)=f\left( x \right)$ then $\int\limits_{0}^{2a}{f\left( x \right)dx}=2\int\limits_{0}^{a}{f\left( x \right)dx}$.
If $f\left( 2a-x \right)=-f\left( x \right)$ then $\int\limits_{0}^{2a}{f\left( x \right)dx}=0$
Since, ${{\cos }^{5}}\left( \pi -x \right)=-{{\cos }^{5}}x$
Therefore,
$I=2\int\limits_{0}^{2\pi }{{{\cos }^{5}}xdx}$
$\Rightarrow I=2\left( 0 \right)$
$\Rightarrow I=0$
15. $\int\limits_{0}^{\dfrac{\pi }{2}}{\dfrac{\sin x-\cos x}{1+\sin x\cos x}dx}$
Ans: Let $I=\int\limits_{0}^{\dfrac{\pi }{2}}{\dfrac{\sin x-\cos x}{1+\sin x\cos x}dx}$ ……(1)
Since,
$\int\limits_{0}^{a}{f\left( x \right)dx}=\int\limits_{0}^{a}{f\left( a-x \right)dx}$
Therefore,
$I=\int\limits_{0}^{\dfrac{\pi }{2}}{\dfrac{\sin \left( \dfrac{\pi }{2}-x \right)-\cos \left( \dfrac{\pi }{2}-x \right)}{1+\sin \left( \dfrac{\pi }{2}-x \right)\cos \left( \dfrac{\pi }{2}-x \right)}dx}$
$\Rightarrow I=\int\limits_{0}^{\dfrac{\pi }{2}}{\dfrac{\cos x-\sin x}{1+\cos x\sin x}dx}$ ……(2)
On adding equation 1 and 2
$\Rightarrow 2I=\int\limits_{0}^{\dfrac{\pi }{2}}{\dfrac{0}{1+\cos x\sin x}dx}$
$\Rightarrow I=0$
16. $\int\limits_{0}^{\pi }{\log \left( 1+\cos x \right)dx}$
Ans: Let
$I=\int\limits_{0}^{\pi }{\log \left( 1+\cos x \right)dx}$ …….(1)
Since,
$\int\limits_{0}^{a}{f\left( x \right)dx}=\int\limits_{0}^{a}{f\left( a-x \right)dx}$
Therefore,
$\Rightarrow I=\int\limits_{0}^{\pi }{\log \left( 1+\cos \left( \pi -x \right) \right)dx}$
$\Rightarrow I=\int\limits_{0}^{\pi }{\log \left( 1-\cos x \right)dx}$ …….(2)
On adding equation 1 and 2-
$2I=\int\limits_{0}^{\pi }{\left\{ \log \left( 1-\cos x \right)+\log \left( 1-\cos x \right) \right\}dx}$
$\Rightarrow 2I=\int\limits_{0}^{\pi }{\log \left( 1-{{\cos }^{2}}x \right)dx}$
$\Rightarrow 2I=\int\limits_{0}^{\pi }{\log {{\sin }^{2}}xdx}$
$\Rightarrow 2I=2\int\limits_{0}^{\pi }{\log \sin xdx}$
$\Rightarrow I=\int\limits_{0}^{\pi }{\log \sin xdx}$ …..(3)
Since, $\sin \left( \pi -x \right)=\sin x$
Therefore, $I=\int\limits_{0}^{\dfrac{\pi }{2}}{\log \sin xdx}$ ……(4)
$\Rightarrow I=2\int\limits_{0}^{\dfrac{\pi }{2}}{\log \sin \left( \dfrac{\pi }{2}-x \right)dx}$
$\Rightarrow I=2\int\limits_{0}^{\dfrac{\pi }{2}}{\log \cos xdx}$ …….(5)
On adding equation 4 and 5-
$2I=\int\limits_{0}^{\dfrac{\pi }{2}}{\left( \log \sin x+\log \cos x \right)dx}$
$\Rightarrow I=\int\limits_{0}^{\dfrac{\pi }{2}}{\left( \log \sin x+\log \cos x+\log 2-\log 2 \right)dx}$
$\Rightarrow I=\int\limits_{0}^{\dfrac{\pi }{2}}{\left( \log 2\sin x\cos x-\log 2 \right)dx}$
$\Rightarrow I=\int\limits_{0}^{\dfrac{\pi }{2}}{\left( \log 2\sin x\cos x \right)dx}-\int\limits_{0}^{\dfrac{\pi }{2}}{\log 2dx}$
Let $2x=t$
On differentiating-
$2dx=dt$
If $x=0$ then $t=0$.
Thus,
$\Rightarrow I=\dfrac{I}{2}-\dfrac{\pi }{2}\log 2$
$\Rightarrow \dfrac{I}{2}=-\dfrac{\pi }{2}\log 2$
$\Rightarrow I=-\pi \log 2$
17. $\int\limits_{0}^{a}{\dfrac{\sqrt{x}}{\sqrt{x}+\sqrt{a-x}}dx}$
Ans: Let $I=\int\limits_{0}^{a}{\dfrac{\sqrt{x}}{\sqrt{x}+\sqrt{a-x}}dx}$ …….(1)
Since,
$\int\limits_{0}^{a}{f\left( x \right)dx}=\int\limits_{0}^{a}{f\left( a-x \right)dx}$
Therefore,
$I=\int\limits_{0}^{a}{\dfrac{\sqrt{a-x}}{\sqrt{a-x}+\sqrt{x}}dx}$ …….(2)
On adding equation 1 and 2-
$2I=\int\limits_{0}^{a}{\dfrac{\sqrt{x}+\sqrt{a-x}}{\sqrt{a-x}+\sqrt{x}}dx}$
$\Rightarrow 2I=\int\limits_{0}^{a}{dx}$
$\Rightarrow 2I=\left[ x \right]_{0}^{a}$
$\Rightarrow 2I=a$
$\Rightarrow I=\dfrac{a}{2}$
18. $\int\limits_{0}^{4}{\left| x-1 \right|dx}$
Ans: Let $I=\int\limits_{0}^{4}{\left| x-1 \right|dx}$
Thus, $\left( x-1 \right)\le 0$ when $0\le x\le 1$ and $\left( x-1 \right)\ge 0$ when $1\le x\le 4$
Since, $\int\limits_{a}^{b}{f\left( x \right)}dx=\int\limits_{a}^{c}{f\left( x \right)dx}+\int\limits_{c}^{b}{f\left( x \right)}$
Therefore, $I=\int\limits_{0}^{1}{\left| x-1 \right|}dx+\int\limits_{1}^{4}{\left| x-1 \right|dx}$
$\Rightarrow I=\int\limits_{0}^{1}{-\left( x-1 \right)}dx+\int\limits_{1}^{4}{\left( x-1 \right)dx}$
$=\left[ x-\dfrac{{{x}^{2}}}{2} \right]_{0}^{1}+\left[ \dfrac{{{x}^{2}}}{2}-x \right]_{1}^{4}$
$=1-\dfrac{1}{2}+\dfrac{{{\left( 4 \right)}^{2}}}{2}-4-\dfrac{1}{2}+1$
$=1-\dfrac{1}{2}+8-4-\dfrac{1}{2}+1$
$=5$
19. Show that $\int\limits_{0}^{a}{f\left( x \right)g\left( x \right)}dx=2\int\limits_{0}^{a}{f\left( x \right)}dx$ , if $f$and $g$ are defined as $f\left( x \right)=f\left( a-x \right)$ and $g\left( x \right)+g\left( a-x \right)=4$ .
Ans: Let $\int\limits_{0}^{a}{f\left( x \right)}g\left( x \right)dx$ …….(1)
Since,
$\int\limits_{0}^{a}{f\left( x \right)dx}=\int\limits_{0}^{a}{f\left( a-x \right)dx}$
Therefore,
$\Rightarrow \int\limits_{0}^{a}{f\left( a-x \right)}g\left( a-x \right)dx$
$\Rightarrow \int\limits_{0}^{a}{f\left( x \right)}g\left( a-x \right)dx$ ……(2)
On adding equation 1 and 2-
$2I=\int\limits_{0}^{a}{\left\{ f\left( x \right)g\left( x \right)+f\left( x \right)g\left( a-x \right) \right\}dx}$
$\Rightarrow 2I=\int\limits_{0}^{a}{f\left( x \right)\left\{ g\left( x \right)+g\left( a-x \right) \right\}dx}$
As, $g\left( x \right)+g\left( a-x \right)=4$.
Thus,
$\Rightarrow 2I=\int\limits_{0}^{a}{4f\left( x \right)dx}$
$\Rightarrow I=2\int\limits_{0}^{a}{f\left( x \right)dx}$
Hence, $\int\limits_{0}^{a}{f\left( x \right)g\left( x \right)}dx=2\int\limits_{0}^{a}{f\left( x \right)}dx$ , if $f$and $g$ are defined as $f\left( x \right)=f\left( a-x \right)$ and $g\left( x \right)+g\left( a-x \right)=4$ .
20. The value of $\int\limits_{\dfrac{-\pi }{2}}^{\dfrac{\pi }{2}}{\left( {{x}^{3}}+x\cos x+{{\tan }^{5}}x+1 \right)dx}$ is
$0$
$2$
$\pi $
$1$
Ans: Let $I=\int\limits_{\dfrac{-\pi }{2}}^{\dfrac{\pi }{2}}{\left( {{x}^{3}}+x\cos x+{{\tan }^{5}}x+1 \right)dx}$
$\Rightarrow I=\int\limits_{\dfrac{-\pi }{2}}^{\dfrac{\pi }{2}}{\left( {{x}^{3}} \right)dx}+\int\limits_{\dfrac{-\pi }{2}}^{\dfrac{\pi }{2}}{\left( x\cos x \right)dx}+\int\limits_{\dfrac{-\pi }{2}}^{\dfrac{\pi }{2}}{\left( {{\tan }^{5}}x \right)dx}+\int\limits_{\dfrac{-\pi }{2}}^{\dfrac{\pi }{2}}{\left( 1 \right)dx}$
If $f\left( x \right)$ is an even function, then $\int\limits_{-a}^{a}{f\left( x \right)dx}=2\int\limits_{0}^{a}{f\left( x \right)}dx$
And $f\left( x \right)$ is an odd function, then $\int\limits_{-a}^{a}{f\left( x \right)dx}=0$
Thus,
$I=0+0+0+2\int\limits_{0}^{\dfrac{\pi }{2}}{dx}$
$=2\left[ x \right]_{0}^{\dfrac{\pi }{2}}$
$=2\left[ \dfrac{\pi }{2} \right]$
$=\pi $
Hence, the correct option is C.
21. The value of $\int\limits_{0}^{\dfrac{\pi }{2}}{\left( \dfrac{4+3\sin x}{4+3\cos x} \right)dx}$ is
$2$
$\dfrac{3}{4}$
$0$
$-2$
Ans: Let $I=\int\limits_{0}^{\dfrac{\pi }{2}}{\left( \dfrac{4+3\sin x}{4+3\cos x} \right)dx}$ …..(1)
Since,
$\int\limits_{0}^{a}{f\left( x \right)dx}=\int\limits_{0}^{a}{f\left( a-x \right)dx}$
Therefore,
$\Rightarrow I=\int\limits_{0}^{\dfrac{\pi }{2}}{\left( \dfrac{4+3\sin \left( \dfrac{\pi }{2}-x \right)}{4+3\cos \left( \dfrac{\pi }{2}-x \right)} \right)dx}$
$\Rightarrow I=\int\limits_{0}^{\dfrac{\pi }{2}}{\left( \dfrac{4+3\cos x}{4+3\sin x} \right)dx}$ …….(2)
On adding equation 1 and 2-
$2I=\int\limits_{0}^{\dfrac{\pi }{2}}{\log 1dx}$
$\Rightarrow 2I=\int\limits_{0}^{\dfrac{\pi }{2}}{0dx}$
$\Rightarrow I=0$
Hence, the correct option is C.
Miscellaneous Exercise
1. $\dfrac{1}{x-{{x}^{3}}}$
Ans: Given $\dfrac{1}{x-{{x}^{3}}}$
So, $\dfrac{1}{x-{{x}^{3}}}=\dfrac{1}{x\left( 1-{{x}^{2}} \right)}$
$=\dfrac{1}{x\left( 1-x \right)\left( 1+x \right)}$
Let $\dfrac{1}{x\left( 1-x \right)\left( 1+x \right)}=\dfrac{A}{x}+\dfrac{B}{\left( 1-x \right)}+\dfrac{C}{1+x}$ …….(1)
$\Rightarrow 1=A\left( 1-{{x}^{2}} \right)+Bx\left( 1+x \right)+Cx\left( 1-x \right)$
$\Rightarrow 1=A-A{{x}^{2}}+Bx+B{{x}^{2}}+Cx-C{{x}^{2}}$
On equating the coefficients of ${{x}^{2}},x$ and constant term –
$-A+B-C=0$
$B+C=0$
$A=1$
Thus, we get $A=1,B=\dfrac{1}{2}$ and $C=-\dfrac{1}{2}$
By equation 1-
$\dfrac{1}{x\left( 1-x \right)\left( 1+x \right)}=\dfrac{1}{x}+\dfrac{1}{2\left( 1-x \right)}-\dfrac{1}{2\left( 1+x \right)}$
$\Rightarrow \int{\dfrac{1}{x\left( 1-x \right)\left( 1+x \right)}}dx=\int{\dfrac{1}{x}dx}+\dfrac{1}{2}\int{\dfrac{1}{\left( 1-x \right)}}dx-\dfrac{1}{2}\int{\dfrac{1}{\left( 1+x \right)}dx}$
$=\log x-\dfrac{1}{2}\log \left( 1-x \right)-\dfrac{1}{2}\log \left( 1+x \right)$
$=\log x-\dfrac{1}{2}\log {{\left( 1-x \right)}^{\dfrac{1}{2}}}-\dfrac{1}{2}\log {{\left( 1+x \right)}^{\dfrac{1}{2}}}$
$=\log \left( \dfrac{x}{{{\left( 1-x \right)}^{\dfrac{1}{2}}}{{\left( 1+x \right)}^{\dfrac{1}{2}}}} \right)+C$
$=\log {{\left( \dfrac{{{x}^{2}}}{\left( 1-{{x}^{2}} \right)} \right)}^{\dfrac{1}{2}}}+C$
$=\dfrac{1}{2}\log \left( \dfrac{{{x}^{2}}}{1-{{x}^{2}}} \right)+C$
2. $\dfrac{1}{\sqrt{x+a}+\sqrt{x+b}}$
Ans: Given $\dfrac{1}{\sqrt{x+a}+\sqrt{x+b}}$
$=\dfrac{1}{\sqrt{x+a}+\sqrt{x+b}}\times \dfrac{\sqrt{x+a}-\sqrt{x+b}}{\sqrt{x+a}-\sqrt{x+b}}$
$=\dfrac{\sqrt{x+a}-\sqrt{x+b}}{\left( x+a \right)-\left( x+b \right)}$
$=\dfrac{\sqrt{x+a}-\sqrt{x+b}}{a-b}$
$\Rightarrow \int{\dfrac{1}{\sqrt{x+a}+\sqrt{x+b}}}dx=\dfrac{1}{a-b}\int{\left( \sqrt{x+a}-\sqrt{x+b} \right)}dx$
$=\dfrac{1}{a-b}\left[ \dfrac{{{\left( x+a \right)}^{\dfrac{3}{2}}}}{\dfrac{3}{2}}-\dfrac{{{\left( x+b \right)}^{\dfrac{3}{2}}}}{\dfrac{3}{2}} \right]$
$=\dfrac{2}{3\left( a-b \right)}\left[ {{\left( x+a \right)}^{\dfrac{3}{2}}}-{{\left( x+b \right)}^{\dfrac{3}{2}}} \right]+C$
3. $\dfrac{1}{x\sqrt{ax-{{x}^{2}}}}$ $\left[ \text{Hint: x=}\dfrac{a}{t} \right]$
Ans: Given
$\dfrac{1}{x\sqrt{ax-{{x}^{2}}}}$
Let $x=\dfrac{a}{t}$
On differentiating-
$dx=-\dfrac{a}{{{t}^{2}}}dt$
$\Rightarrow \int{\dfrac{1}{x\sqrt{ax-{{x}^{2}}}}dx=\int{\dfrac{1}{\dfrac{a}{t}\sqrt{a.\dfrac{a}{t}-{{\left( \dfrac{a}{t} \right)}^{2}}}}}\left( -\dfrac{a}{{{t}^{2}}}dt \right)}$
$=-\int{\dfrac{1}{at}.\dfrac{1}{\sqrt{\dfrac{1}{t}-\dfrac{1}{{{t}^{2}}}}}}dt$
$=-\dfrac{1}{a}\int{\dfrac{1}{\sqrt{\dfrac{{{t}^{2}}}{t}-\dfrac{{{t}^{2}}}{{{t}^{2}}}}}}dt$
$=-\dfrac{1}{a}\int{\dfrac{1}{\sqrt{t-1}}}dt$
$=-\dfrac{1}{a}\left[ 2\sqrt{t-1} \right]+C$
Substituting value of t-
$=-\dfrac{1}{a}\left[ 2\sqrt{\dfrac{a}{x}-1} \right]+C$
$=-\dfrac{2}{a}\left[ \dfrac{\sqrt{a-x}}{\sqrt{x}} \right]+C$
$=-\dfrac{2}{a}\left[ \sqrt{\dfrac{a-x}{x}} \right]+C$
4. Integrate the expression: $\dfrac{\text{1}}{{{\text{x}}^{\text{2}}}{{\left( {{\text{x}}^{\text{4}}}\text{+1} \right)}^{\dfrac{\text{3}}{\text{4}}}}}$.
Ans:The given expression is, $\dfrac{1}{{{x}^{2}}{{\left( {{x}^{4}}+1 \right)}^{\dfrac{3}{4}}}}$.
On multiplying and dividing by ${{x}^{-3}}$, the following can be obtained as shown below,
$\dfrac{{{x}^{-3}}}{{{x}^{2}}.{{x}^{-3}}{{\left( {{x}^{4}}+1 \right)}^{\dfrac{3}{4}}}}=\dfrac{{{x}^{-3}}{{\left( {{x}^{4}}+1 \right)}^{\dfrac{-3}{4}}}}{{{x}^{2}}-{{x}^{-3}}}=\dfrac{{{\left( {{x}^{4}}+1 \right)}^{\dfrac{-3}{4}}}}{{{x}^{5}}{{\left( {{x}^{4}} \right)}^{\dfrac{-3}{4}}}}=\dfrac{1}{{{x}^{5}}}{{\left( \dfrac{{{x}^{4}}+1}{{{x}^{4}}} \right)}^{\dfrac{-3}{4}}}=\dfrac{1}{{{x}^{5}}}{{\left( 1+\dfrac{1}{{{x}^{4}}} \right)}^{\dfrac{-3}{4}}}$
Now, consider $\dfrac{1}{{{x}^{4}}}=t$
$\therefore \dfrac{-4}{{{x}^{5}}}=\dfrac{dt}{dx}$
$\Rightarrow \dfrac{dx}{{{x}^{5}}}=\dfrac{-dt}{4}$
$\therefore \int{\dfrac{1}{{{x}^{2}}{{\left( {{x}^{4}}+1 \right)}^{\dfrac{3}{4}}}}dx}=\int{\dfrac{1}{{{x}^{5}}}{{\left( 1+\dfrac{1}{{{x}^{4}}} \right)}^{\dfrac{-3}{4}}}dx}=\dfrac{-1}{4}\int{{{\left( 1+t \right)}^{\dfrac{-3}{4}}}dt}$
$\Rightarrow \int{\dfrac{1}{{{x}^{2}}{{\left( {{x}^{4}}+1 \right)}^{\dfrac{3}{4}}}}dx}=\dfrac{-1}{4}\left[ \dfrac{{{\left( 1+t \right)}^{\dfrac{1}{4}}}}{\dfrac{1}{4}} \right]+C=-{{\left( 1+\dfrac{1}{{{x}^{4}}} \right)}^{\dfrac{1}{4}}}+C$, where $C$ is any arbitrary constant.
5. Integrate the expression: $\dfrac{\text{1}}{{{\text{x}}^{\dfrac{\text{1}}{\text{2}}}}\text{+}{{\text{x}}^{\dfrac{\text{1}}{\text{3}}}}}$. $\text{[}$Hint: $\dfrac{\text{1}}{{{\text{x}}^{\dfrac{\text{1}}{\text{2}}}}\text{+}{{\text{x}}^{\dfrac{\text{1}}{\text{3}}}}}\text{=}\dfrac{\text{1}}{{{\text{x}}^{\dfrac{\text{1}}{\text{3}}}}\left( \text{1+}{{\text{x}}^{\dfrac{\text{1}}{\text{6}}}} \right)}$ Put, $\text{x=}{{\text{t}}^{\text{6}}}$ $\text{]}$
Ans: The given expression is, $\dfrac{1}{{{x}^{\dfrac{1}{2}}}+{{x}^{\dfrac{1}{3}}}}$.
Observe the given hint and obtain as shown below,
$\therefore \dfrac{1}{{{x}^{\dfrac{1}{2}}}+{{x}^{\dfrac{1}{3}}}}=\dfrac{1}{{{x}^{\dfrac{1}{3}}}\left( 1+{{x}^{\dfrac{1}{6}}} \right)}$
Consider $x={{t}^{6}}$
$\therefore x={{t}^{6}}\Rightarrow dx=6{{t}^{5}}$
$\therefore \int{\dfrac{1}{{{x}^{\dfrac{1}{2}}}+{{x}^{\dfrac{1}{3}}}}dx}=\int{\dfrac{1}{{{x}^{\dfrac{1}{3}}}\left( 1+{{x}^{\dfrac{1}{6}}} \right)}dx}=\int{\dfrac{6{{t}^{5}}}{{{t}^{2}}\left( 1+t \right)}dt}=6\int{\dfrac{{{t}^{3}}}{\left( 1+t \right)}dt}$
Now on dividing, we can obtain as shown below,
$\int{\dfrac{1}{{{x}^{\dfrac{1}{2}}}+{{x}^{\dfrac{1}{3}}}}dx}=6\int{\left\{ \left( {{t}^{2}}-t+1 \right)-\dfrac{1}{1+t} \right\}dt}$
$=6\int{\left[ \left( \dfrac{{{t}^{3}}}{3} \right)-\left( \dfrac{{{t}^{2}}}{2} \right)+t-\log \left| 1+t \right| \right]dt}$
$=2{{x}^{\dfrac{1}{2}}}-3{{x}^{\dfrac{1}{3}}}+6{{x}^{\dfrac{1}{6}}}-6\log \left( 1+{{x}^{\dfrac{1}{6}}} \right)+C$
$=2\sqrt{x}-3{{x}^{\dfrac{1}{3}}}+6{{x}^{\dfrac{1}{6}}}-6\log \left( 1+{{x}^{\dfrac{1}{6}}} \right)+C$, where $C$ is any arbitrary constant.
6. Integrate the expression: $\dfrac{\text{5x}}{\text{(x+1)}\left( {{\text{x}}^{\text{2}}}\text{+9} \right)}$.
Ans: The given expression is, $\dfrac{5x}{(x+1)\left( {{x}^{2}}+9 \right)}$.
Now consider it as shown below,
$\therefore \dfrac{5x}{(x+1)\left( {{x}^{2}}+9 \right)}=\dfrac{A}{\left( x+1 \right)}+\dfrac{Bx+C}{\left( {{x}^{2}}+9 \right)}\,\,\,\,\,\,\,\,\,\,\,...\left( 1 \right)$
$\Rightarrow 5x=A\left( {{x}^{2}}+9 \right)+Bx+C\left( x+1 \right)$
$\Rightarrow 5x=A{{x}^{2}}+9A+B{{x}^{2}}+Bx+Cx+C$
On equating the coefficients of ${{x}^{2}},\,x$ and constant term, it can be obtained that,
$A+B=0\,\,\,...\left( 2 \right)$
$B+C=5\,\,\,...\left( 3 \right)$
$9A+C=0\,\,\,...\left( 4 \right)$
And on solving these equations, the values of $A,\,B,\,C$ can be obtained as,
$A=\dfrac{-1}{2},\,B=\dfrac{1}{2},\,C=\dfrac{9}{2}$ respectively.
Now, from equation $\left( 1 \right)$ it can be clearly obtained that,
$\int{\dfrac{5x}{(x+1)\left( {{x}^{2}}+9 \right)}dx}=\int{\left\{ \dfrac{-1}{2\left( x+1 \right)}+\dfrac{x+9}{2\left( {{x}^{2}}+9 \right)} \right\}dx}$
$=\dfrac{-1}{2}\log \left| x+1 \right|+\dfrac{1}{2}\int{\dfrac{x}{{{x}^{2}}+9}dx}+\dfrac{9}{2}\int{\dfrac{1}{{{x}^{2}}+9}dx}$
$=\dfrac{-1}{2}\log \left| x+1 \right|+\dfrac{1}{4}\log \left| {{x}^{2}}+9 \right|+\left( \dfrac{9}{2} \right)\left( \dfrac{1}{3} \right){{\tan }^{-1}}\left( \dfrac{x}{3} \right)$
$=\dfrac{-1}{2}\log \left| x+1 \right|+\dfrac{1}{4}\log \left| {{x}^{2}}+9 \right|+\dfrac{3}{2}{{\tan }^{-1}}\left( \dfrac{x}{3} \right)+C$, where $C$ is any arbitrary constant.
7. Integrate the expression, that is, $\dfrac{\text{sinx}}{\text{sin}\left( \text{x- }\!\!\alpha\!\!\text{ } \right)}$.
Ans: The given expression is, $\dfrac{\sin x}{\sin \left( x-\alpha \right)}$.
Now, substitute $x-\alpha =t$
$\therefore dx=dt$
$\therefore \int{\dfrac{\sin x}{\sin \left( x-\alpha \right)}dx}=\int{\dfrac{\sin \left( t+\alpha \right)}{\sin t}dt}=\int{\dfrac{\sin t\cos \alpha +\cos tsin\alpha }{\sin t}dt}=\int{\cos \alpha +\cot tsin\alpha dt}$$\Rightarrow \int{\dfrac{\sin x}{\sin \left( x-\alpha \right)}dx}=t\cos \alpha +\sin \alpha \log \left| \sin t \right|+{{C}_{1}}=\left( x-\alpha \right)\cos \alpha +\sin \alpha \log \left| \sin \left( x-\alpha \right) \right|+{{C}_{1}}$
$\Rightarrow \int{\dfrac{\sin x}{\sin \left( x-\alpha \right)}dx}=x\cos \alpha +\sin \alpha \log \left| \sin \left( x-\alpha \right) \right|-\alpha \cos \alpha +{{C}_{1}}=x\cos \alpha +\sin \alpha \log \left| \sin \left( x-\alpha \right) \right|+C$
where ${{C}_{1}},\,C$ are any arbitrary constants and $C={{C}_{1}}-\alpha \cos \alpha $.
8. Integrate the expression, that is, $\dfrac{{{\text{e}}^{\text{5logx}}}\text{-}{{\text{e}}^{\text{4logx}}}}{{{\text{e}}^{\text{3logx}}}\text{-}{{\text{e}}^{\text{2logx}}}}$.
Ans: The given expression is, $\dfrac{{{e}^{5\log x}}-{{e}^{4\log x}}}{{{e}^{3\log x}}-{{e}^{2\log x}}}$.
$\therefore \dfrac{{{e}^{5\log x}}-{{e}^{4\log x}}}{{{e}^{3\log x}}-{{e}^{2\log x}}}=\dfrac{{{e}^{4\log x}}\left( {{e}^{\log x}}-1 \right)}{{{e}^{2\log x}}\left( {{e}^{\log x}}-1 \right)}={{e}^{2\log x}}={{x}^{2}}$
Now, integrate the given expression as shown below
$\therefore \int{\dfrac{{{e}^{5\log x}}-{{e}^{4\log x}}}{{{e}^{3\log x}}-{{e}^{2\log x}}}dx}=\int{{{x}^{2}}dx}=\dfrac{{{x}^{3}}}{3}+C$, where $C$ is any arbitrary constant.
9. Integrate the expression, that is, $\dfrac{\text{cosx}}{\sqrt{\text{4-si}{{\text{n}}^{\text{2}}}\text{x}}}$.
Ans: The given expression is, $\dfrac{\cos x}{\sqrt{4-{{\sin }^{2}}x}}$.
Now, substitute $\sin x=t$
$\therefore \cos xdx=dt$
$\therefore \int{\dfrac{\cos x}{\sqrt{4-{{\sin }^{2}}x}}dx}=\int{\dfrac{dt}{\sqrt{{{\left( 2 \right)}^{2}}-{{\left( t \right)}^{2}}}}}={{\sin }^{-1}}\left( \dfrac{t}{2} \right)+C={{\sin }^{-1}}\left( \dfrac{\sin x}{2} \right)+C$, where $C$ is any arbitrary constant.
10. Integrate the expression, that is, $\dfrac{\text{si}{{\text{n}}^{\text{8}}}\text{x-co}{{\text{s}}^{\text{8}}}\text{x}}{\text{1-2si}{{\text{n}}^{\text{2}}}\text{xco}{{\text{s}}^{\text{2}}}\text{x}}$.
Ans: The given expression is, $\dfrac{{{\sin }^{8}}x-{{\cos }^{8}}x}{1-2{{\sin }^{2}}x{{\cos }^{2}}x}$.
$\therefore \dfrac{{{\sin }^{8}}x-{{\cos }^{8}}x}{1-2{{\sin }^{2}}x{{\cos }^{2}}x}=\dfrac{\left( {{\sin }^{4}}x-{{\cos }^{4}}x \right)\left( {{\sin }^{4}}x+{{\cos }^{4}}x \right)}{{{\sin }^{2}}x+{{\cos }^{2}}x-{{\sin }^{2}}x{{\cos }^{2}}x-{{\sin }^{2}}x{{\cos }^{2}}x}$
$=\dfrac{\left( {{\sin }^{2}}x+{{\cos }^{2}}x \right)\left( {{\sin }^{2}}x-{{\cos }^{2}}x \right)\left( {{\sin }^{4}}x+{{\cos }^{4}}x \right)}{{{\sin }^{2}}x\left( 1-{{\cos }^{2}}x \right)+{{\cos }^{2}}x\left( 1-{{\sin }^{2}}x \right)}$
$=\dfrac{-\left( -{{\sin }^{2}}x+{{\cos }^{2}}x \right)\left( {{\sin }^{4}}x+{{\cos }^{4}}x \right)}{{{\sin }^{4}}x+{{\cos }^{4}}x}=-\cos 2x$
Now, integrate the given expression as shown below
$\therefore \int{\dfrac{{{\sin }^{8}}x-{{\cos }^{8}}x}{1-2{{\sin }^{2}}x{{\cos }^{2}}x}dx}=\int{-\cos 2xdx}=\dfrac{-\sin 2x}{2}+C$, where $C$ is any arbitrary constant.
11. Integrate the expression, that is, $\dfrac{\text{1}}{\text{cos}\left( \text{x+a} \right)\text{cos}\left( \text{x+b} \right)}$.
Ans: The given expression is, $\dfrac{1}{\cos \left( x+a \right)\cos \left( x+b \right)}$.
On multiplying and dividing by $\sin \left( \alpha -\beta \right)$, the following can be obtained as shown below,
$\dfrac{1}{\sin \left( a-b \right)}\left[ \dfrac{\sin \left( a-b \right)}{\cos \left( x+a \right)\cos \left( x+b \right)} \right]$
$\Rightarrow \dfrac{1}{\sin \left( a-b \right)}\left[ \dfrac{\sin \left[ \left( x+a \right)-\left( x+b \right) \right]}{\cos \left( x+a \right)\cos \left( x+b \right)} \right]$
$\Rightarrow \dfrac{1}{\sin \left( a-b \right)}\left[ \dfrac{\sin \left( x+a \right)\cos \left( x+b \right)-\cos \left( x+a \right)\sin \left( x+b \right)}{\cos \left( x+a \right)\cos \left( x+b \right)} \right]$
$\Rightarrow \dfrac{1}{\sin \left( a-b \right)}\left[ \dfrac{\sin (x+a)}{\cos (x+a)}-\dfrac{\sin \left( x+b \right)}{\cos (x+b)} \right]=\dfrac{1}{\sin (a-b)}\left( \tan (x+a)-\tan (x+b) \right)$
$\therefore \int{\dfrac{1}{\cos \left( x+a \right)\cos \left( x+b \right)}dx}=\dfrac{1}{\sin (a-b)}\int{\left( \tan (x+a)-\tan (x+b) \right)dx}$
$\Rightarrow \int{\dfrac{1}{\cos \left( x+a \right)\cos \left( x+b \right)}dx}=\dfrac{1}{\sin (a-b)}\left[ \log \left| \dfrac{\cos (x+b)}{\cos (x-a)} \right| \right]+C$, where $C$ is any arbitrary constant.
12. Integrate the expression, that is, $\dfrac{{{\text{x}}^{\text{3}}}}{\sqrt{\text{1-}{{\text{x}}^{\text{8}}}}}$.
Ans: The given expression is, $\dfrac{{{x}^{3}}}{\sqrt{1-{{x}^{8}}}}$.
Now, substitute ${{x}^{4}}=t$
$\therefore 4{{x}^{3}}dx=dt$
$\therefore\int{\dfrac{{{x}^{3}}}{\sqrt{1-{{x}^{8}}}}dx}=\dfrac{1}{4}\int{\dfrac{dt}{\sqrt{1-{{\left( t \right)}^{2}}}}}=\dfrac{1}{4}{{\sin }^{-1}}t+C=\dfrac{1}{4}{{\sin }^{-1}}\left( {{x}^{4}} \right)+C$, where $C$ is any arbitrary constant.
13. Integrate the expression, that is, $\dfrac{{{\text{e}}^{\text{x}}}}{\left( \text{1+}{{\text{e}}^{\text{x}}} \right)\left( \text{2+}{{\text{e}}^{\text{x}}} \right)}$.
Ans: The given expression is, $\dfrac{{{e}^{x}}}{\left( 1+{{e}^{x}} \right)\left( 2+{{e}^{x}} \right)}$.
Now, substitute ${{e}^{x}}=t$
$\therefore {{e}^{x}}dx=dt$
$\therefore \int{\dfrac{{{e}^{x}}}{\left( 1+{{e}^{x}} \right)\left( 2+{{e}^{x}} \right)}dx}=\int{\dfrac{dt}{\left( t+1 \right)\left( t+2 \right)}}=\int{\left[ \dfrac{1}{\left( t+1 \right)}-\dfrac{1}{\left( t+2 \right)} \right]dt}$
$\Rightarrow \int{\dfrac{{{e}^{x}}}{\left( 1+{{e}^{x}} \right)\left( 2+{{e}^{x}} \right)}dx}=\log \left| t+1 \right|-\log \left| t+2 \right|+C=\log \left| \dfrac{{{e}^{x}}+1}{{{e}^{x}}+2} \right|+C$, where $C$ is any arbitrary constant.
14. Integrate the expression, $\dfrac{\text{1}}{\text{(}{{\text{x}}^{\text{2}}}\text{+1)}\left( {{\text{x}}^{\text{2}}}\text{+4} \right)}$.
Ans: The given expression is, $\dfrac{1}{({{x}^{2}}+1)\left( {{x}^{2}}+4 \right)}$.
Now consider it as shown below,
$\therefore \dfrac{1}{({{x}^{2}}+1)\left( {{x}^{2}}+4 \right)}=\dfrac{Ax+B}{\left( {{x}^{2}}+1 \right)}+\dfrac{Cx+D}{\left( {{x}^{2}}+4 \right)}\,\,\,\,\,\,\,\,\,\,\,...\left( 1 \right)$
$\Rightarrow 1=\left( Ax+B \right)\left( {{x}^{2}}+4 \right)+\left( Bx+C \right)\left( {{x}^{2}}+9 \right)$
$\Rightarrow 1=A{{x}^{3}}+4Ax+B{{x}^{2}}+4B+C{{x}^{3}}+Cx+D{{x}^{2}}+D$
On equating the coefficients of ${{x}^{3}},\,{{x}^{2}},\,x$ and constant term, it can be obtained that,
$A+C=0\,\,\,...\left( 2 \right)$
$B+D=0\,\,\,...\left( 3 \right)$
$4A+C=0\,\,\,...\left( 4 \right)$
$4B+D=1\,\,\,...\left( 5 \right)$
And on solving these equations, the values of $A,\,B,\,C,D$ can be obtained as,
$A=0,\,B=\dfrac{1}{3},\,C=0,\,D=\dfrac{1}{3}$ respectively.
Now, from equation $\left( 1 \right)$ it can be clearly obtained that,
$\int{\dfrac{1}{({{x}^{2}}+1)\left( {{x}^{2}}+4 \right)}dx}=\dfrac{1}{3}\int{\left\{ \dfrac{1}{\left( {{x}^{2}}+1 \right)}-\dfrac{1}{\left( {{x}^{2}}+4 \right)}\, \right\}dx}$
$=\dfrac{1}{3}{{\tan }^{-1}}x-\dfrac{1}{\left( 3 \right)\left( 2 \right)}{{\tan }^{-1}}\dfrac{x}{2}+C$
$=\dfrac{1}{3}{{\tan }^{-1}}x-\dfrac{1}{6}{{\tan }^{-1}}\dfrac{x}{2}+C$, where $C$ is any arbitrary constant.
15. Integrate the expression, that is, $\text{co}{{\text{s}}^{\text{3}}}\text{x}{{\text{e}}^{\text{logsinx}}}$.
Ans: The given expression is, ${{\cos }^{3}}x{{e}^{\log \sin x}}$.
Observe as shown below,
$\therefore {{\cos }^{3}}x{{e}^{\log \sin x}}={{\cos }^{3}}x\sin x$
Now, consider $cosx=t$
$\therefore -\sin xdx=dt$
$\therefore \int{{{\cos }^{3}}x{{e}^{\log \sin x}}dx}=\int{{{\cos }^{3}}x\sin xdx}=-\int{{{t}^{3}}dt}=\dfrac{-{{t}^{4}}}{4}+C=\dfrac{-{{\cos }^{4}}x}{4}+C$, where $C$ is any arbitrary constant.
16. Integrate the expression, that is, ${{\text{e}}^{\text{3logx}}}{{\left( {{\text{x}}^{\text{4}}}\text{+1} \right)}^{\text{-1}}}$.
Ans: The given expression is, ${{e}^{3\log x}}{{\left( {{x}^{4}}+1 \right)}^{-1}}$.
Observe as shown below,
$\therefore {{e}^{3\log x}}{{\left( {{x}^{4}}+1 \right)}^{-1}}=\dfrac{{{x}^{3}}}{\left( {{x}^{4}}+1 \right)}$
Now, consider ${{x}^{4}}+1=t$
$\therefore 4{{x}^{3}}dx=dt$
$\therefore \int{{{e}^{3\log x}}{{\left( {{x}^{4}}+1 \right)}^{-1}}dx}=\int{\dfrac{{{x}^{3}}}{{{x}^{4}}+1}dx}=\dfrac{1}{4}\int{\dfrac{dt}{t}}=\dfrac{1}{4}\log \left| t \right|+C=\dfrac{\log \left| {{x}^{4}}+1 \right|}{4}+C$, where $C$ is any arbitrary constant.
17. Integrate the expression, that is, $\text{f }\!\!'\!\!\text{ }\left( \text{ax+b} \right){{\left[ \text{f(ax+b)} \right]}^{\text{n}}}$.
Ans: The given expression is, $f'\left( ax+b \right){{\left[ f(ax+b) \right]}^{n}}$.
Now, consider $\left[ f(ax+b) \right]=t$
$\therefore af'(ax+b)dx=dt$
$\therefore \int{f'\left( ax+b \right){{\left[ f(ax+b) \right]}^{n}}dx}=\dfrac{1}{a}\int{{{t}^{n}}dt}=\dfrac{{{t}^{n+1}}}{a\left( n+1 \right)}+C=\dfrac{{{\left[ f(ax+b) \right]}^{n+1}}}{a\left( n+1 \right)}+C$, where $C$ is any arbitrary constant.
18. Integrate the expression, that is, $\dfrac{\text{1}}{\sqrt{\text{si}{{\text{n}}^{\text{3}}}\text{xsin}\left( \text{x+ }\!\!\alpha\!\!\text{ } \right)}}$.
Ans: The given expression is, $\dfrac{1}{\sqrt{{{\sin }^{3}}x\sin \left( x+\alpha \right)}}$.
$\therefore \dfrac{1}{\sqrt{{{\sin }^{3}}x\sin \left( x+\alpha \right)}}=\dfrac{1}{\sqrt{{{\sin }^{3}}x\sin x\cos \alpha +\cos \alpha \sin x}}$
$=\dfrac{1}{\sqrt{{{\sin }^{4}}x\cos \alpha +{{\sin }^{3}}x\sin \alpha \cos x}}$
$=\dfrac{1}{{{\sin }^{2}}x\sqrt{\cos \alpha +\sin \alpha \cot x}}=\dfrac{\cos e{{c}^{2}}x}{\sqrt{\cos \alpha +\sin \alpha \cot x}}$
Now, substitute $\cos \alpha +\sin \alpha \cot x=t$
$\therefore -\cos e{{c}^{2}}x\sin \alpha dx=dt$
$ \therefore \int{\dfrac{1}{\sqrt{{{\sin }^{3}}x\sin \left( x+\alpha \right)}}dx}=\int{\dfrac{\cos e{{c}^{2}}x}{\sqrt{\cos \alpha +\sin \alpha \cot x}}}=\dfrac{-1}{\sin \alpha }\int{\dfrac{dt}{\sqrt{t}}}=\dfrac{-2\sqrt{t}}{\sin \alpha }+C $
$ \Rightarrow \int{\dfrac{1}{\sqrt{{{\sin }^{3}}x\sin \left( x+\alpha \right)}}dx}=\dfrac{-2\sqrt{\cos \alpha +\sin \alpha \cot x}}{\sin \alpha }+C=\dfrac{-2}{\sin \alpha }\sqrt{\cos \alpha +\dfrac{\sin \alpha \cos x}{\sin x}}+C $
, where $C$ is any arbitrary constant.
19. Integrate the expression, that is, $\sqrt{\dfrac{\text{1-}\sqrt{\text{x}}}{\text{1+}\sqrt{\text{x}}}}$.
Ans: The given expression is, $\sqrt{\dfrac{1-\sqrt{x}}{1+\sqrt{x}}}$.
Assume, $I=\int{\sqrt{\dfrac{1-\sqrt{x}}{1+\sqrt{x}}}dx}$
Now, substitute $x={{\cos }^{2}}\theta $
$\therefore dx=-2\sin \theta \cos \theta dt$
$\therefore I=\int{\sqrt{\dfrac{1-\cos \theta }{1+\cos \theta }}\left( -2\sin \theta \cos \theta \right)d\theta }=-\int{\sqrt{\dfrac{2{{\sin }^{2}}\dfrac{\theta }{2}}{2{{\cos }^{2}}\dfrac{\theta }{2}}}\sin 2\theta d\theta }=-\int{\tan \dfrac{\theta }{2}2\sin \theta \cos \theta d\theta }$
$\Rightarrow I=-2\int{\dfrac{\sin \dfrac{\theta }{2}}{\cos \dfrac{\theta }{2}}2\sin \dfrac{\theta }{2}\cos \dfrac{\theta }{2}\cos \theta d\theta }=-4\int{{{\sin }^{2}}\dfrac{\theta }{2}(2{{\cos }^{2}}\dfrac{\theta }{2}-1)d\theta }$
$\Rightarrow I=-8\int{{{\sin }^{2}}\dfrac{\theta }{2}{{\cos }^{2}}\dfrac{\theta }{2}d\theta }+4\int{{{\sin }^{2}}\dfrac{\theta }{2}d}\theta =2\int{{{\sin }^{2}}\dfrac{\theta }{2}d\theta }+4\int{{{\sin }^{2}}\dfrac{\theta }{2}d}\theta $
$\Rightarrow I=-2\int{\left( \dfrac{1-\cos 2\theta }{2} \right)d\theta }+4\int{\left( \dfrac{1-\cos \theta }{2} \right)d}\theta =-2\left[ \dfrac{\theta }{2}-\dfrac{\sin 2\theta }{2} \right]+4\left[ \dfrac{\theta }{2}-\dfrac{\sin \theta }{2} \right]+C$$\Rightarrow I=-\theta +\dfrac{\sin 2\theta }{2}+2\sin \theta +C=\theta +\sqrt{1-{{\cos }^{2}}\theta }\cos \theta -2\sqrt{1-{{\cos }^{2}}\theta }+C$
$\Rightarrow I={{\cos }^{-1}}\sqrt{x}+\sqrt{x(1-x)}-2\sqrt{1-x}+C=-2\sqrt{1-x}+{{\cos }^{-1}}\sqrt{x}+\sqrt{x-{{x}^{2}}}+C$, where $C$ is any arbitrary constant.
20. Integrate the expression, that is, $\dfrac{\text{2+sin2x}}{\text{1+cos2x}}{{\text{e}}^{\text{x}}}$.
Ans: The given expression is, $\dfrac{2+\sin 2x}{1+\cos 2x}{{e}^{x}}$.
Assume, $I=\int{\dfrac{2+\sin 2x}{1+\cos 2x}{{e}^{x}}dx}$
$\Rightarrow I=\int{\dfrac{2+2\sin x\cos x}{2{{\cos }^{2}}x}{{e}^{x}}dx}=\int{\left( \dfrac{1+\sin x\cos x}{{{\cos }^{2}}x} \right){{e}^{x}}dx}=\int{\left( {{\sec }^{2}}x+\tan x \right){{e}^{x}}dx}$
Now, consider $f\left( x \right)=\tan x$
$\therefore f'\left( x \right)={{\sec }^{2}}xdx$
$\therefore I=\int{\dfrac{2+\sin 2x}{1+\cos 2x}{{e}^{x}}}dx=\int{\left( f\left( x \right)+f'\left( x \right) \right){{e}^{x}}dx}={{e}^{x}}f\left( x \right)+C={{e}^{x}}\tan x+C$, where $C$ is any arbitrary constant.
21. Integrate the expression: $\dfrac{{{\text{x}}^{\text{2}}}\text{+x+1}}{{{\text{(x+1)}}^{\text{2}}}\left( \text{x+2} \right)}$.
Ans: The given expression is, $\dfrac{{{x}^{2}}+x+1}{{{(x+1)}^{2}}\left( x+2 \right)}$.
Now consider it as shown below,
$\therefore \dfrac{{{x}^{2}}+x+1}{{{(x+1)}^{2}}\left( x+2 \right)}=\dfrac{A}{\left( x+1 \right)}+\dfrac{B}{\left( x+1 \right)}+\dfrac{C}{\left( x+2 \right)}\,\,\,\,\,\,\,\,\,\,\,...\left( 1 \right)$
$\Rightarrow {{x}^{2}}+x+1=A\left( x+1 \right)\left( x+2 \right)+B\left( x+2 \right)+C{{\left( x+1 \right)}^{2}}$
$\Rightarrow {{x}^{2}}+x+1=A\left( {{x}^{2}}+3x+2 \right)+B\left( x+2 \right)+C\left( {{x}^{2}}+2x+1 \right)$
$\Rightarrow {{x}^{2}}+x+1=\left( A+C \right){{x}^{2}}+x\left( 3A+B+2C \right)+\left( 2A+2B+C \right)$
On equating the coefficients of ${{x}^{2}},\,x$ and constant term, it can be obtained that,
$A+C=1\,\,\,...\left( 2 \right)$
$3A+B+2C=1\,\,\,...\left( 3 \right)$
$2A+2B+C=1\,\,\,...\left( 4 \right)$
And on solving these equations, the values of $A,\,B,\,C$ can be obtained as,
$A=-2,\,B=1,\,C=3$ respectively.
Now, from equation $\left( 1 \right)$ it can be clearly obtained that,
$\int{\dfrac{{{x}^{2}}+x+1}{{{(x+1)}^{2}}\left( x+2 \right)}dx}=\int{\left\{ \dfrac{-2}{\left( x+1 \right)}+\dfrac{1}{\left( x+1 \right)}+\dfrac{3}{\left( x+2 \right)} \right\}dx}$
$=-2\int{\dfrac{1}{x+1}dx}+\int{\dfrac{1}{{{\left( x+1 \right)}^{2}}}dx}+3\int{\dfrac{1}{x+2}dx}$
$=-2\log \left| x+1 \right|+3\log \left| x+2 \right|-\dfrac{1}{(x+1)}+C$, where $C$ is any arbitrary constant.
22. Integrate the expression, that is, $\text{ta}{{\text{n}}^{\text{-1}}}\sqrt{\dfrac{\text{1-x}}{\text{1+x}}}$.
Ans: The given expression is, ${{\tan }^{-1}}\sqrt{\dfrac{1-x}{1+x}}$.
Assume, $I=\int{{{\tan }^{-1}}\sqrt{\dfrac{1-x}{1+x}}dx}$
Now, consider $x=\cos \theta $
$\therefore dx=-\sin \theta d\theta $
$\therefore I=\int{{{\tan }^{-1}}\sqrt{\dfrac{1-\cos \theta }{1+\cos \theta }}\left( -\sin \theta \right)d\theta }=-\int{{{\tan }^{-1}}\sqrt{\dfrac{2{{\sin }^{2}}\dfrac{\theta }{2}}{2{{\cos }^{2}}\dfrac{\theta }{2}}}\sin \theta d\theta }=-\int{{{\tan }^{-1}}\tan \dfrac{\theta }{2}\sin \theta d\theta }$ $\Rightarrow I=-\dfrac{1}{2}\int{\theta \sin \theta d\theta }=-\dfrac{1}{2}\left[ \theta \left( -\cos \theta \right)-\int{1.(-\cos \theta )d\theta } \right]=-\dfrac{1}{2}\left[ \theta \left( \cos \theta \right)+\sin \theta \right]$$\Rightarrow I=\dfrac{x}{2}{{\cos }^{-1}}x-\dfrac{1}{2}\sqrt{1-{{x}^{2}}}+C=\dfrac{1}{2}\left( x{{\cos }^{-1}}x-\sqrt{1-{{x}^{2}}} \right)+C$, where $C$ is any arbitrary constant.
23. Integrate the expression, that is, $\dfrac{\sqrt{{{\text{x}}^{\text{2}}}\text{+1}}\left[ \text{log}\left( {{\text{x}}^{\text{2}}}\text{+1} \right)\text{-2logx} \right]}{{{\text{x}}^{\text{4}}}}$.
Ans: The given expression is, $\dfrac{\sqrt{{{x}^{2}}+1}\left[ \log \left( {{x}^{2}}+1 \right)-2\log x \right]}{{{x}^{4}}}$.
Assume, $I=\int{\dfrac{\sqrt{{{x}^{2}}+1}\left[ \log \left( {{x}^{2}}+1 \right)-2\log x \right]}{{{x}^{4}}}dx}$ $\Rightarrow I=\dfrac{\sqrt{{{x}^{2}}+1}}{{{x}^{4}}}\left[ \log \left( {{x}^{2}}+1 \right)-\log {{x}^{2}} \right]=\dfrac{\sqrt{{{x}^{2}}+1}}{{{x}^{4}}}\left[ \log \left( \dfrac{{{x}^{2}}+1}{{{x}^{2}}} \right) \right]=\dfrac{1}{{{x}^{3}}}\sqrt{\dfrac{{{x}^{2}}+1}{{{x}^{2}}}}\left[ \log \left( 1+\dfrac{1}{{{x}^{2}}} \right) \right]$Consider $1+\dfrac{1}{{{x}^{2}}}=t$
$\therefore \dfrac{-2}{{{x}^{3}}}dx=dt$
Now, integrate the given expression as shown below $\therefore I=\int{\dfrac{\sqrt{{{x}^{2}}+1}\left[ \log \left( {{x}^{2}}+1 \right)-2\log x \right]}{{{x}^{4}}}dx}=\int{\dfrac{1}{{{x}^{3}}}\sqrt{\dfrac{{{x}^{2}}+1}{{{x}^{2}}}}\left[ \log \left( 1+\dfrac{1}{{{x}^{2}}} \right) \right]dx}=\dfrac{-1}{2}\int{{{t}^{\dfrac{1}{2}}}\log tdt}+C$Using integration by parts, it can be obtained that,
$I=\dfrac{-1}{2}\left[ \log t.\int{{{t}^{\dfrac{1}{2}}}dt-\left\{ \left( \dfrac{d}{dt}\log t \right)\int{{{t}^{\dfrac{1}{2}}}dt} \right\}dt} \right]=\dfrac{-1}{2}\left[ \log t.\dfrac{{{t}^{\dfrac{3}{2}}}}{\dfrac{3}{2}}-\int{\dfrac{1}{t}.\dfrac{{{t}^{\dfrac{3}{2}}}}{\dfrac{3}{2}}dt} \right]$
$\Rightarrow I=\dfrac{-1}{2}\left[ \dfrac{2}{3}{{t}^{\dfrac{3}{2}}}\log t-\dfrac{2}{3}\int{{{t}^{\dfrac{1}{2}}}dt} \right]=\dfrac{-1}{3}{{t}^{\dfrac{3}{2}}}\log t+\dfrac{2}{9}{{t}^{\dfrac{3}{2}}}=\dfrac{-1}{3}{{t}^{\dfrac{3}{2}}}\left[ \log t-\dfrac{2}{3} \right]$
$\Rightarrow I=\dfrac{-1}{2}\left[ \dfrac{2}{3}{{t}^{\dfrac{3}{2}}}\log t-\dfrac{2}{3}\int{{{t}^{\dfrac{1}{2}}}dt} \right]=\dfrac{-1}{3}{{t}^{\dfrac{3}{2}}}\log t+\dfrac{2}{9}{{t}^{\dfrac{3}{2}}}=\dfrac{-1}{3}{{t}^{\dfrac{3}{2}}}\left[ \log t-\dfrac{2}{3} \right]$
$\Rightarrow I=\dfrac{-1}{2}\left[ 1+\dfrac{1}{{{x}^{2}}} \right]\left( \log \left( 1+\dfrac{1}{{{x}^{2}}} \right)-\dfrac{2}{3} \right)+C$, where $C$ is any arbitrary constant.
24. Find the value of the expression, that is, $\int_{\dfrac{\text{ }\!\!\pi\!\!\text{ }}{\text{2}}}^{\text{ }\!\!\pi\!\!\text{ }}{{{\text{e}}^{\text{x}}}\left( \dfrac{\text{1-sinx}}{\text{1-cosx}} \right)\text{dx}}$.
Ans: The given expression is, $\int_{\dfrac{\pi }{2}}^{\pi }{{{e}^{x}}\left( \dfrac{1-\sin x}{1-\cos x} \right)dx}$.
Assume, $I=\int_{\dfrac{\pi }{2}}^{\pi }{{{e}^{x}}\left( \dfrac{1-\sin x}{1-\cos x} \right)dx}$
$\Rightarrow I=\int_{\dfrac{\pi }{2}}^{\pi }{{{e}^{x}}\left( \dfrac{1-2\sin \dfrac{x}{2}\cos \dfrac{x}{2}}{+2{{\sin }^{2}}\dfrac{x}{2}} \right)dx=}\int_{\dfrac{\pi }{2}}^{\pi }{{{e}^{x}}\left( \dfrac{\cos e{{c}^{2}}\dfrac{x}{2}}{2}-\cot \dfrac{x}{2} \right)dx}$
Now, substitute $f\left( x \right)=-\cot \dfrac{x}{2}$
$\therefore f'\left( x \right)=-\left( -\dfrac{1}{2}\cos e{{c}^{2}}\dfrac{x}{2} \right)dx=\dfrac{1}{2}\cos e{{c}^{2}}\dfrac{x}{2}dx$
$\therefore I=\int_{\dfrac{\pi }{2}}^{\pi }{{{e}^{x}}\left( f(x)+f'(x) \right)dx}=\left[ {{e}^{x}}f(x) \right]_{\dfrac{\pi }{2}}^{\pi }=\left[ {{e}^{x}}\cot \dfrac{x}{2} \right]_{\dfrac{\pi }{2}}^{\pi }$
$\Rightarrow I=\left[ {{e}^{\pi }}\cot \dfrac{\pi }{2}-{{e}^{\dfrac{\pi }{2}}}\cot \dfrac{\pi }{4} \right]=\left[ 0-{{e}^{\dfrac{\pi }{2}}} \right]=-{{e}^{\dfrac{\pi }{2}}}$
25. Find the value of the expression, that is, $\int_{\text{0}}^{\dfrac{\text{ }\!\!\pi\!\!\text{ }}{\text{4}}}{\dfrac{\text{sinxcosx}}{\text{si}{{\text{n}}^{\text{4}}}\text{x+co}{{\text{s}}^{\text{4}}}\text{x}}\text{dx}}$.
Ans: The given expression is, $\int_{0}^{\dfrac{\pi }{4}}{\dfrac{\sin x\cos x}{{{\sin }^{4}}x+{{\cos }^{4}}x}dx}$.
Assume, $I=\int_{0}^{\dfrac{\pi }{4}}{\dfrac{\sin x\cos x}{{{\sin }^{4}}x+{{\cos }^{4}}x}dx}$
$\Rightarrow I=\int_{0}^{\dfrac{\pi }{4}}{\dfrac{\dfrac{\sin x\cos x}{{{\cos }^{4}}x}}{\dfrac{{{\sin }^{4}}x+{{\cos }^{4}}x}{{{\cos }^{4}}x}}dx}=\int_{0}^{\dfrac{\pi }{4}}{\dfrac{\tan x{{\sec }^{2}}x}{1+{{\tan }^{4}}x}dx}$
Now, substitute ${{\tan }^{2}}x=t$
$\therefore 2\tan x{{\sec }^{2}}xdx=dt$
And also when $x=0,\,t=0$ and when $x=\dfrac{\pi }{4},\,t=1$.
$\therefore I=\dfrac{1}{2}\int_{0}^{1}{\dfrac{dt}{1+{{t}^{2}}}}=\dfrac{1}{2}\left[ {{\tan }^{-1}}t \right]_{0}^{1}=\dfrac{1}{2}\left[ {{\tan }^{-1}}\left( 1 \right)-{{\tan }^{-1}}\left( 0 \right) \right]=\dfrac{1}{2}\left( \dfrac{\pi }{4} \right)=\dfrac{\pi }{8}$
26. Find the value of the expression, that is, $\int_{\text{0}}^{\dfrac{\text{ }\!\!\pi\!\!\text{ }}{\text{2}}}{\dfrac{\text{co}{{\text{s}}^{\text{2}}}\text{x}}{\text{co}{{\text{s}}^{\text{2}}}\text{x+4si}{{\text{n}}^{\text{2}}}\text{x}}\text{dx}}$.
Ans: The given expression is, $\int_{0}^{\dfrac{\pi }{2}}{\dfrac{{{\cos }^{2}}x}{{{\cos }^{2}}x+4{{\sin }^{2}}x}dx}$.
Assume, $I=\int_{0}^{\dfrac{\pi }{2}}{\dfrac{{{\cos }^{2}}x}{{{\cos }^{2}}x+4{{\sin }^{2}}x}dx}$
$\Rightarrow I=\int_{0}^{\dfrac{\pi }{2}}{\dfrac{{{\cos }^{2}}x}{{{\cos }^{2}}x+4\left( 1-{{\cos }^{2}}x \right)}dx}=\dfrac{-1}{3}\int_{0}^{\dfrac{\pi }{2}}{\dfrac{4-4-3{{\cos }^{2}}x}{-3{{\cos }^{2}}x+4}dx}$
$\Rightarrow I=-\dfrac{1}{3}\int_{0}^{\dfrac{\pi }{2}}{\dfrac{4-3{{\cos }^{2}}x}{4-3{{\cos }^{2}}x}dx}+\dfrac{1}{3}\int_{0}^{\dfrac{\pi }{2}}{\dfrac{4}{4-3{{\cos }^{2}}x}dx}=-\dfrac{1}{3}\int_{0}^{\dfrac{\pi }{2}}{dx}+\dfrac{1}{3}\int_{0}^{\dfrac{\pi }{2}}{\dfrac{4se{{c}^{2}}x}{4{{\sec }^{2}}x-3}dx}$
$\Rightarrow I=\dfrac{-1}{3}\left[ x \right]_{0}^{\dfrac{\pi }{2}}+\dfrac{1}{3}\int_{0}^{\dfrac{\pi }{2}}{\dfrac{4se{{c}^{2}}x}{4\left( 1+{{\tan }^{2}}x \right)-3}dx}=\dfrac{-\pi }{6}+\dfrac{2}{3}\int_{0}^{\dfrac{\pi }{2}}{\dfrac{2se{{c}^{2}}x}{\left( 1+4{{\tan }^{2}}x \right)}dx}\,\,\,...\left( 1 \right)$
Observe, $\int_{0}^{\dfrac{\pi }{2}}{\dfrac{2se{{c}^{2}}x}{\left( 1+4{{\tan }^{2}}x \right)}dx}$
Now, substitute $2\tan x=t$
$\therefore 2{{\sec }^{2}}xdx=dt$
And also when $x=0,\,t=0$ and when $x=\dfrac{\pi }{2},\,t=\infty $.
$\therefore \int_{0}^{\dfrac{\pi }{2}}{\dfrac{2se{{c}^{2}}x}{\left( 1+4{{\tan }^{2}}x \right)}dx}=\int_{0}^{\infty }{\dfrac{dt}{\left( 1+{{t}^{2}} \right)}dx}=\left[ {{\tan }^{-1}}\left( t \right) \right]_{0}^{\infty }=\left[ {{\tan }^{-1}}\left( \infty \right)-{{\tan }^{-1}}\left( 0 \right) \right]=\dfrac{\pi }{2}$Henceforth from equation $\left( 1 \right)$, it can be obtained that,
$I=-\dfrac{\pi }{6}+\dfrac{2}{3}\left( \dfrac{\pi }{2} \right)=-\dfrac{\pi }{6}+\dfrac{2\pi }{6}=\dfrac{\pi }{6}$
27. Find the value of the expression, that is, $\int_{\dfrac{\text{ }\!\!\pi\!\!\text{ }}{\text{6}}}^{\dfrac{\text{ }\!\!\pi\!\!\text{ }}{\text{2}}}{\dfrac{\text{sinx+cosx}}{\sqrt{\text{sin2x}}}\text{dx}}$.
Ans: The given expression is, $\int_{\dfrac{\pi }{6}}^{\dfrac{\pi }{2}}{\dfrac{\sin x+\cos x}{\sqrt{\sin 2x}}dx}$.
Assume, $I=\int_{\dfrac{\pi }{6}}^{\dfrac{\pi }{2}}{\dfrac{\sin x+\cos x}{\sqrt{\sin 2x}}dx}$
$\Rightarrow I=\int_{0}^{\dfrac{\pi }{2}}{\dfrac{\sin x+\cos x}{\sqrt{-\left( -1+1-2\sin x\cos x \right)}}dx}=\int_{0}^{\dfrac{\pi }{2}}{\dfrac{\sin x+\cos x}{\sqrt{1-\left( {{\sin }^{2}}x+{{\cos }^{2}}x-2\sin x\cos x \right)}}dx}$
$\Rightarrow I=\int_{0}^{\dfrac{\pi }{2}}{\dfrac{\sin x+\cos x}{\sqrt{1-{{\left( \sin x-\cos x \right)}^{2}}}}dx}$
Now, substitute $\left( \sin x-\cos x \right)=t$
$\therefore (\sin x+\cos x)dx=dt$
And also when $x=\dfrac{\pi }{6},\,t=\left( \dfrac{1-\sqrt{3}}{2} \right)$ and when $x=\dfrac{\pi }{3},\,t=\left( \dfrac{\sqrt{3}-1}{2} \right)$.
$\therefore I=\int_{\dfrac{1-\sqrt{3}}{2}}^{\dfrac{\sqrt{3}-1}{2}}{\dfrac{dt}{\sqrt{1-{{t}^{2}}}}}=\int_{-\left( \dfrac{-1+\sqrt{3}}{2} \right)}^{\dfrac{\sqrt{3}-1}{2}}{\dfrac{dt}{\sqrt{1-{{t}^{2}}}}}$
As $\dfrac{1}{\sqrt{1-{{\left( -t \right)}^{2}}}}=\dfrac{1}{\sqrt{1-{{t}^{2}}}}$, it can be thus obtained that $\dfrac{1}{\sqrt{1-{{t}^{2}}}}$ is an even function,
$\therefore \int_{-a}^{a}{f\left( x \right)dx}=2\int_{0}^{a}{f\left( x \right)dx}$
$\therefore I=2\int_{0}^{\dfrac{\sqrt{3}-1}{2}}{\dfrac{dt}{\sqrt{1-{{t}^{2}}}}}=\left[ 2{{\sin }^{-1}}t \right]_{0}^{\dfrac{\sqrt{3}-1}{2}}=2{{\sin }^{-1}}\left( \dfrac{\sqrt{3}-1}{2} \right)$ $x=\dfrac{\pi }{6},\,t=\left( \dfrac{1-\sqrt{3}}{2} \right)$ and when $x=\dfrac{\pi }{3},\,t=\left( \dfrac{\sqrt{3}-1}{2} \right)$.
28. Find the value of the expression, that is, $\int_{\text{0}}^{\text{1}}{\dfrac{\text{dx}}{\sqrt{\text{1+x}}\text{-}\sqrt{\text{x}}}}$.
Ans: The given expression is, $\int_{0}^{1}{\dfrac{dx}{\sqrt{1+x}-\sqrt{x}}}$.
Assume, $I=\int_{0}^{1}{\dfrac{dx}{\sqrt{1+x}-\sqrt{x}}}$
$\Rightarrow I=\int_{0}^{1}{\dfrac{1}{\sqrt{1+x}-\sqrt{x}}\times \dfrac{\sqrt{1+x}+\sqrt{x}}{\sqrt{1+x}+\sqrt{x}}dx}=\int_{0}^{1}{\dfrac{\sqrt{1+x}+\sqrt{x}}{1+x-x}dx}$
$\Rightarrow I=\int_{0}^{1}{\sqrt{1+x}dx}+\int_{0}^{1}{\sqrt{x}dx}=\dfrac{2}{3}\left[ {{\left( 1+x \right)}^{\dfrac{2}{3}}} \right]_{0}^{1}+\dfrac{2}{3}\left[ {{\left( x \right)}^{\dfrac{3}{2}}} \right]_{0}^{1}=\dfrac{2}{3}\left[ {{\left( 2 \right)}^{\dfrac{2}{3}}}-1 \right]+\dfrac{2}{3}=\dfrac{4\sqrt{2}}{3}$
29. Find the value of the expression, that is, $\int_{\text{0}}^{\dfrac{\text{ }\!\!\pi\!\!\text{}}{\text{4}}}{\dfrac{\text{sinx+cosx}}{\text{9+16sin2x}}\text{dx}}$.
Ans: The given expression is, $\int_{0}^{\dfrac{\pi }{4}}{\dfrac{\sin x+\cos x}{9+16\sin 2x}dx}$.
Assume, $I=\int_{0}^{\dfrac{\pi }{4}}{\dfrac{\sin x+\cos x}{9+16\sin 2x}dx}$
Now, substitute $\sin x-\cos x=t$
$\therefore \left( \cos x+\sin x \right)dx=dt$
And also when $x=0,\,t=-1$ and when $x=\dfrac{\pi }{4},\,t=0$.
$\therefore {{\left( \sin x-\cos x \right)}^{2}}={{t}^{2}}$
$\Rightarrow 1-2\sin x\cos x={{t}^{2}}$
$\Rightarrow 1-\sin 2x={{t}^{2}}$
$\Rightarrow \sin 2x=1-{{t}^{2}}$
$\therefore I=\int_{-1}^{0}{\dfrac{dt}{9+16\left( 1-{{t}^{2}} \right)}}=\int_{-1}^{0}{\dfrac{dt}{25-16{{t}^{2}}}}=\int_{-1}^{0}{\dfrac{dt}{{{\left( 5 \right)}^{2}}-{{\left( 4t \right)}^{2}}}}$
$\Rightarrow I=\dfrac{1}{4}\left[ \dfrac{1}{2\left( 5 \right)}\log \left| \dfrac{5+4t}{5-4t} \right| \right]_{-1}^{0}=\dfrac{1}{40}\left[ \log \left| 1 \right|-\log \left| \dfrac{1}{9} \right| \right]=\dfrac{1}{40}\log \left| 9 \right|$
30. Find the value of the expression, that is, $\int_{\text{0}}^{\dfrac{\text{ }\!\!\pi\!\!\text{ }}{\text{2}}}{\text{sin2xta}{{\text{n}}^{\text{-1}}}\left( \text{sinx} \right)\text{dx}}$.
Ans: The given expression is, $\int_{0}^{\dfrac{\pi }{2}}{\sin 2x{{\tan }^{-1}}\left( \sin x \right)dx}$.
Assume, $I=\int_{0}^{\dfrac{\pi }{2}}{\sin 2x{{\tan }^{-1}}\left( \sin x \right)dx}$
$\Rightarrow I=\int_{0}^{\dfrac{\pi }{2}}{\sin 2x{{\tan }^{-1}}\left( \sin x \right)dx}=\int_{0}^{\dfrac{\pi }{2}}{2\sin x\cos x{{\tan }^{-1}}\left( \sin x \right)dx}$
Now, substitute $\sin x=t$
$\therefore \cos xdx=dt$
And also when $x=0,\,t=0$ and when $x=\dfrac{\pi }{2},\,t=1$.
$\therefore I=2\int_{0}^{1}{t{{\tan }^{-1}}\left( t \right)dt}$
Observe, $\int{t{{\tan }^{-1}}\left( t \right)dt}$
$\therefore \int{t{{\tan }^{-1}}\left( t \right)dt}={{\tan }^{-1}}t\int{tdt}-\int{\left\{ \dfrac{d\left( {{\tan }^{-1}}t \right)}{dt}\int{tdt} \right\}dt}={{\tan }^{-1}}t.\dfrac{{{t}^{2}}}{2}-\int{\dfrac{1}{1+{{t}^{2}}}.\dfrac{{{t}^{2}}}{2}dt}$
$=\dfrac{{{t}^{2}}{{\tan }^{-1}}t}{2}-\int{\dfrac{{{t}^{2}}+1-1}{1+{{t}^{2}}}dt}=\dfrac{{{t}^{2}}{{\tan }^{-1}}t}{2}-\int{1dt}+\int{\dfrac{1}{1+{{t}^{2}}}dt}=\dfrac{{{t}^{2}}{{\tan }^{-1}}t}{2}-\dfrac{1}{2}t+\dfrac{1}{2}{{\tan }^{-1}}t$
$\therefore \int_{0}^{1}{t.{{\tan }^{-1}}tdt}=\left[ \dfrac{{{t}^{2}}{{\tan }^{-1}}t}{2}-\dfrac{1}{2}t+\dfrac{1}{2}{{\tan }^{-1}}t \right]_{0}^{1}=\dfrac{1}{2}\left[ \dfrac{\pi }{4}-1+\dfrac{\pi }{4} \right]=\dfrac{\pi }{4}-\dfrac{1}{2}$
Henceforth from equation $\left( 1 \right)$, it can be obtained that,
$I=2\left[ \dfrac{\pi }{2}-\dfrac{1}{2} \right]=\dfrac{\pi }{2}-1$
31. Find the value of the expression, that is, $\int_{\text{1}}^{\text{4}}{\left[ \left| \text{x-1} \right|\text{+}\left| \text{x-2} \right|\text{+}\left| \text{x-3} \right| \right]\text{dx}}$.
Ans: The given expression is, $\int_{1}^{4}{\left[ \left| x-1 \right|+\left| x-2 \right|+\left| x-3 \right| \right]dx}$.
Assume, $\int_{1}^{4}{\left[ \left| x-1 \right|+\left| x-2 \right|+\left| x-3 \right| \right]dx}$
$\Rightarrow I=\int_{1}^{4}{\left| x-1 \right|dx}+\int_{1}^{4}{\left| x-2 \right|dx}+\int_{1}^{4}{\left| x-3 \right|dx}$
$\therefore I={{I}_{1}}+{{I}_{2}}+{{I}_{3}}\,\,\,...\left( 1 \right)$
where, ${{I}_{1}}=\int_{1}^{4}{\left| x-1 \right|dx},\,{{I}_{2}}=\int_{1}^{4}{\left| x-2 \right|dx},\,{{I}_{3}}=+\int_{1}^{4}{\left| x-3 \right|dx}$
Now, consider, ${{I}_{1}}=\int_{1}^{4}{\left| x-1 \right|dx}$, where $\left( x-1 \right)\ge 0\,\forall \,1\le x\le 4$ $\therefore {{I}_{1}}=\int_{1}^{4}{\left( x-1 \right)dx}=\left[ \dfrac{{{x}^{2}}}{2}-x \right]_{1}^{4}=\left[ 8-4-\dfrac{1}{2}+1 \right]=\dfrac{9}{2}\,\,\,...\left( 2 \right)\,$
Again, consider, ${{I}_{2}}=\int_{1}^{4}{\left| x-2 \right|dx}$, where $\left( x-2 \right)\ge 0\,\forall \,2\le x\le 4$ and $\left( x-2 \right)\le 0\,\forall \,1\le x\le 2$.
$\therefore {{I}_{2}}=\int_{1}^{2}{\left( 2-x \right)dx}+\int_{2}^{4}{\left( x-2 \right)dx}=\left[ 2x-\dfrac{{{x}^{2}}}{2} \right]_{1}^{4}+\left[ \dfrac{{{x}^{2}}}{2}-2x \right]_{2}^{4}$
$\Rightarrow {{I}_{2}}=\left[ 4-2-2+\dfrac{1}{2} \right]+\left[ 8-8-2+4 \right]=\dfrac{1}{2}+2=\dfrac{5}{2}\,\,\,...\left( 3 \right)\,$
Also, consider, ${{I}_{3}}=\int_{1}^{4}{\left| x-3 \right|dx}$, where $\left( x-3 \right)\ge 0\,\forall \,3\le x\le 4$ and $\left( x-3 \right)\le 0\,\forall \,1\le x\le 3$.
$\therefore {{I}_{3}}=\int_{1}^{3}{\left( 3-x \right)dx}+\int_{3}^{4}{\left( x-3 \right)dx}=\left[ 3-\dfrac{{{x}^{2}}}{2} \right]_{1}^{3}+\left[ \dfrac{{{x}^{2}}}{2}-3x \right]_{3}^{4}$
$\Rightarrow {{I}_{3}}=\left[ 9-\dfrac{9}{2}-3+\dfrac{1}{2} \right]+\left[ 8-12-\dfrac{9}{2}+9 \right]=2+\dfrac{1}{2}=\dfrac{5}{2}\,\,\,...\left( 4 \right)\,$
Now, from equations $\left( 1 \right)$, $\left( 2 \right)$, $\left( 3 \right)$ and $\left( 4 \right)$ it can be obtained that,
$I=\dfrac{9}{2}+\dfrac{5}{2}+\dfrac{5}{2}=\dfrac{19}{2}$
$\Rightarrow 2I=\pi \int_{0}^{\pi }{\dfrac{\sin x+1-1}{1+\sin x}dx}=\pi \int_{0}^{\pi }{dx}-\pi \int_{0}^{\pi }{\dfrac{1}{1+\sin x}dx}=\pi \left[ x \right]_{0}^{\pi }-\pi \int_{0}^{\pi }{\dfrac{1-\sin x}{{{\cos }^{2}}x}dx}$
$\Rightarrow 2I=\pi \left[ x \right]_{0}^{\pi }-\pi \int_{0}^{\pi }{\left( {{\sec }^{2}}x-\tan x\sec x \right)dx}={{\pi }^{2}}-\pi \left[ \tan x-\sec x \right]_{0}^{\pi }$
$\Rightarrow 2I={{\pi }^{2}}-\pi \left[ 0-\left( -1 \right)-0+1 \right]={{\pi }^{2}}-2\pi $
$\Rightarrow I=\dfrac{\pi \left( \pi -2 \right)}{2}$
32. Prove the following equation: $\int_{\text{1}}^{\text{3}}{\dfrac{\text{dx}}{{{\text{x}}^{\text{2}}}\left( \text{x+1} \right)}}\text{=}\dfrac{\text{2}}{\text{3}}\text{+log}\dfrac{\text{2}}{\text{3}}$.
Ans: The given equation is, $\int_{1}^{3}{\dfrac{dx}{{{x}^{2}}\left( x+1 \right)}}=\dfrac{2}{3}+\log \dfrac{2}{3}$.
Assume, $\int_{1}^{3}{\dfrac{dx}{{{x}^{2}}\left( x+1 \right)}}$
Now consider it as shown below,
$\therefore \dfrac{1}{{{x}^{2}}\left( x+1 \right)}=\dfrac{A}{x}+\dfrac{B}{{{x}^{2}}}+\dfrac{C}{\left( x+1 \right)}\,\,\,\,\,\,\,\,\,\,\,...\left( 1 \right)$
$\Rightarrow 1=Ax\left( x+1 \right)+B\left( x+1 \right)+C\left( {{x}^{2}} \right)$
$\Rightarrow 1=A{{x}^{2}}+Ax+Bx+B+C{{x}^{2}}$
On equating the coefficients of ${{x}^{2}},\,x$ and constant term, it can be obtained that,
$A+C=0\,\,\,...\left( 2 \right)$
$A+B=0\,\,\,...\left( 3 \right)$
$B=1\,\,\,...\left( 4 \right)$
And on solving these equations, the values of $A,\,B,\,C$ can be obtained as,
$A=-1,\,B=1,\,C=1$ respectively.
Now, from equation $\left( 1 \right)$ it can be clearly obtained that,
$I=\int_{1}^{3}{\dfrac{dx}{{{x}^{2}}\left( x+1 \right)}}=\int_{1}^{3}{\left\{ \dfrac{-1}{x}+\dfrac{1}{{{x}^{2}}}+\dfrac{1}{\left( x+1 \right)} \right\}dx}$
$\Rightarrow I=\left[ -\log x-\dfrac{1}{x}+\log \left( x+1 \right) \right]_{1}^{3}=\left[ \log \left( \dfrac{x+1}{x} \right)-\dfrac{1}{x} \right]_{1}^{3}=\log \left( \dfrac{4}{3} \right)-\dfrac{1}{3}-\log \left( 2 \right)+1$
$\Rightarrow I=\log 4-\log 3-\log 2+\dfrac{2}{3}=\log 2-\log 3+\dfrac{2}{3}=\log \left( \dfrac{2}{3} \right)+\dfrac{2}{3}$
Henceforth, it can be clearly proved.
33. Prove the following equation: $\int_{\text{0}}^{\text{4}}{\text{x}{{\text{e}}^{\text{x}}}\text{dx}}\text{=1}.
Ans: The given equation is, $\int_{0}^{4}{x{{e}^{x}}dx}=1$.
Assume, $I=\int_{0}^{4}{x{{e}^{x}}dx}$
Using integration by parts, it can be obtained that,
$I=x\int_{0}^{4}{{{e}^{x}}dx}-\int_{0}^{4}{\left\{ \left( \dfrac{d\left( x \right)}{dx} \right)\int{{{e}^{x}}} \right\}}=\left[ x{{e}^{x}} \right]_{0}^{1}-\left[ {{e}^{x}} \right]_{0}^{1}=e-e+1=1$
Henceforth, it can be clearly proved.
34. Prove the following equation: $\int_{\text{1}}^{\text{-1}}{{{\text{x}}^{\text{17}}}\text{co}{{\text{s}}^{\text{4}}}\text{xdx}}\text{=0}$.
Ans: The given equation is, $\int_{1}^{-1}{{{x}^{17}}{{\cos }^{4}}xdx}=0$.
Assume, $I=\int_{1}^{-1}{{{x}^{17}}{{\cos }^{4}}xdx}$
Now, consider $f(x)={{x}^{17}}{{\cos }^{4}}x$
$\therefore f\left( -x \right)={{\left( -x \right)}^{17}}{{\cos }^{4}}\left( -x \right)=-{{x}^{17}}{{\cos }^{4}}x=-f\left( x \right)$
$\Rightarrow f\left( x \right)$ is an odd function and henceforth it is clearly known to us that when $f(x)$ is an odd function, then $\int_{-a}^{a}{f\left( x \right)dx}=0$.
$\therefore I=\int_{1}^{-1}{{{x}^{17}}{{\cos }^{4}}xdx}=0$
Henceforth, it can be clearly proved.
35. Prove the following equation: $\int_{\text{0}}^{\dfrac{\text{ }\!\!\pi\!\!\text{ }}{\text{2}}}{\text{si}{{\text{n}}^{\text{3}}}\text{xdx}}\text{=}\dfrac{\text{2}}{\text{3}}$.
Ans: The given equation is, $\int_{0}^{\dfrac{\pi }{2}}{{{\sin }^{3}}xdx}=\dfrac{2}{3}$.
Assume, $I=\int_{0}^{\dfrac{\pi }{2}}{{{\sin }^{3}}xdx}$
$\Rightarrow I=\int_{0}^{\dfrac{\pi }{2}}{{{\sin }^{2}}x\sin xdx}=\int_{0}^{\dfrac{\pi }{2}}{\left( 1-{{\cos }^{2}}x \right)\sin xdx}=\int_{0}^{\dfrac{\pi }{2}}{\sin xdx}-\int_{0}^{\dfrac{\pi }{2}}{{{\cos }^{2}}x\sin xdx}$
$\Rightarrow I=\left[ -\cos x \right]_{0}^{\dfrac{\pi }{2}}+\left[ \dfrac{co{{s}^{3}}x}{3} \right]_{0}^{\dfrac{\pi }{2}}=1-\dfrac{1}{3}=\dfrac{2}{3}$
Henceforth, it can be clearly proved.
36. Prove the following equation: $\int_{\text{0}}^{\dfrac{\text{ }\!\!\pi\!\!\text{ }}{\text{4}}}{\text{2ta}{{\text{n}}^{\text{3}}}\text{xdx}}\text{=1-log2}$.
Ans: The given equation is, $\int_{0}^{\dfrac{\pi }{4}}{2{{\tan }^{3}}xdx}=1-\log 2$.
Assume, $\int_{0}^{\dfrac{\pi }{4}}{2{{\tan }^{3}}xdx}$
$\Rightarrow I=\int_{0}^{\dfrac{\pi }{4}}{2{{\tan }^{2}}x\tan xdx}=\int_{0}^{\dfrac{\pi }{4}}{\left( 1-{{\sec }^{2}}x \right)\tan xdx}=\int_{0}^{\dfrac{\pi }{4}}{\tan xdx}-\int_{0}^{\dfrac{\pi }{4}}{{{\sec }^{2}}x\tan xdx}$
$\Rightarrow I=2\left[ \dfrac{{{\tan }^{2}}x}{2} \right]_{0}^{\dfrac{\pi }{4}}+2\left[ \log \cos x \right]_{0}^{\dfrac{\pi }{4}}=1+2\left[ \log \cos \dfrac{\pi }{4}-\log \cos 0 \right]_{0}^{\dfrac{\pi }{2}}=1-\log 2-\log 1$
$\Rightarrow I=1-\log 2$
Henceforth, it can be clearly proved.
37. Prove the following equation: $\int_{\text{0}}^{\text{1}}{\text{si}{{\text{n}}^{\text{-1}}}\text{xdx}}\text{=}\dfrac{\text{ }\!\!\pi\!\!\text{ }}{\text{2}}\text{-1}$.
Ans: The given equation is, $\int_{0}^{1}{{{\sin }^{-1}}xdx}=\dfrac{\pi }{2}-1$.
Assume, $I=\int_{0}^{1}{{{\sin }^{-1}}xdx}$
$\Rightarrow I=\int_{0}^{1}{{{\sin }^{-1}}x.1dx}$
Using integration by parts, it can be obtained that,
$I=\left[ {{\sin }^{-1}}x.x \right]_{0}^{1}-\int_{0}^{1}{\dfrac{1}{\sqrt{1-{{x}^{2}}}}xdx}=\left[ x{{\sin }^{-1}}x \right]_{0}^{1}+\dfrac{1}{2}\int_{0}^{1}{\dfrac{\left( -2x \right)}{\sqrt{1-{{x}^{2}}}}dx}$
Now, substitute $1-{{x}^{2}}=t$
$\therefore \left( -2x \right)dx=dt$
And also when $x=0,\,t=1$ and when $x=1,\,t=0$.
$\therefore I=\left[ x{{\sin }^{-1}}x \right]_{0}^{1}+\dfrac{1}{2}\int_{0}^{1}{\dfrac{dt}{\sqrt{t}}}=\left[ x{{\sin }^{-1}}x \right]_{0}^{1}+\dfrac{1}{2}\left[ 2\sqrt{t} \right]_{1}^{0}={{\sin }^{-1}}1-\sqrt{1}=\dfrac{\pi }{2}-1$ Henceforth, it can be clearly proved.
38. The expression, that is, $\int{\dfrac{\text{dx}}{{{\text{e}}^{\text{x}}}\text{+}{{\text{e}}^{\text{-x}}}}}$ is equal to
A. $\text{ta}{{\text{n}}^{\text{-1}}}\left( {{\text{e}}^{\text{x}}} \right)\text{+C}$
B. $\text{ta}{{\text{n}}^{\text{-1}}}\left( {{\text{e}}^{\text{-x}}} \right)\text{+C}$
C. $\text{log}\left( {{\text{e}}^{\text{x}}}\text{-}{{\text{e}}^{\text{-x}}} \right)\text{+C}$
D. $\text{log}\left( {{\text{e}}^{\text{x}}}\text{+}{{\text{e}}^{\text{-x}}} \right)\text{+C}$
Ans: The given expression is, $\int{\dfrac{dx}{{{e}^{x}}+{{e}^{-x}}}}$.
Assume, $I=\int{\dfrac{dx}{{{e}^{x}}+{{e}^{-x}}}}$
Now, consider ${{e}^{x}}=t$
$\therefore {{e}^{x}}dx=dt$
$\therefore I=\int{\dfrac{dx}{{{e}^{x}}+{{e}^{-x}}}}=\int{\dfrac{1}{1+{{t}^{2}}}dt}=\int{{{\tan }^{-1}}\operatorname{t}dt}+C$, where $C$ is any arbitrary constant.
Hence, the correct answer is option (A).
39. The expression, that is, $\int{\dfrac{\text{cos2x}}{{{\left( \text{sinx+cosx} \right)}^{\text{2}}}}\text{dx}}$ is
A. $\dfrac{\text{-1}}{\text{sinx+cosx}}\text{+C}$
B. $\text{log}\left| \text{sinx+cosx} \right|\text{+C}$
C. $\text{log}\left| \text{sinx-cosx} \right|\text{+C}$
D. $\dfrac{\text{1}}{{{\left( \text{sinx+cosx} \right)}^{\text{2}}}}\text{+C}$
Ans: The given expression is, $\int{\dfrac{\cos 2x}{{{\left( \sin x+\cos x \right)}^{2}}}dx}$.
Assume, $I=\int{\dfrac{\cos 2x}{{{\left( \sin x+\cos x \right)}^{2}}}dx}$
$\Rightarrow I=\int{\dfrac{(\sin x+\cos x)(\cos x-\sin x)}{{{\left( \sin x+\cos x \right)}^{2}}}dx}=\int{\dfrac{(\cos x-\sin x)}{\left( \sin x+\cos x \right)}dx}$
Now, substitute $\left( \sin x+\cos x \right)=t$
$\therefore (\cos x-\sin x)dx=dt$
$\therefore I=I=\int{\dfrac{1}{t}dt=\log \left| t \right|+C=\log \left| \cos x+\sin x \right|+C}$, where $C$ is any arbitrary constant.
Hence, the correct answer is option (B).
40. If $\text{f}\left( \text{a+b-x} \right)\text{=f}\left( \text{x} \right)\text{,}\,$then $\int_{\text{a}}^{\text{b}}{\text{xf}\left( \text{x} \right)\text{dx}}$ is equal to
A. $\dfrac{\text{a+b}}{\text{2}}\int_{\text{a}}^{\text{b}}{\text{f}\left( \text{b-x} \right)\text{dx}}$
B. $\dfrac{\text{a+b}}{\text{2}}\int_{\text{a}}^{\text{b}}{\text{f}\left( \text{b+x} \right)\text{dx}}$
C. $\dfrac{\text{b-a}}{\text{2}}\int_{\text{a}}^{\text{b}}{\text{f}\left( \text{x} \right)\text{dx}}$
D. $\dfrac{\text{a+b}}{\text{2}}\int_{\text{a}}^{\text{b}}{\text{f}\left( \text{x} \right)\text{dx}}$
Ans: Assume, $I=\int_{a}^{b}{xf\left( x \right)dx}\,\,\,...\left( 1 \right)$
$\Rightarrow I=\int_{a}^{b}{\left( a+b-x \right)f\left( a+b-x \right)dx}\,\,\,\left[ \because \int_{a}^{b}{f\left( x \right)dx}=\int_{a}^{b}{f\left( a+b-x \right)dx} \right]$
$\Rightarrow I=\int_{a}^{b}{\left( a+b-x \right)f\left( x \right)dx}=\left( a+b \right)\int_{a}^{b}{f\left( x \right)dx}-I$ using $\left( 1 \right)$
$\Rightarrow 2I=\left( a+b \right)\int_{a}^{b}{f\left( x \right)dx}$
$\Rightarrow I=\dfrac{\left( a+b \right)}{2}\int_{a}^{b}{f\left( x \right)dx}$
Hence, the correct answer is option (D).
NCERT Solutions for Class 12 Integration Maths
7.1 Introduction
In Chapter 7 Maths Class 12, the introduction part delves into the history of differential calculus and how integral calculus came into existence. You would learn how the original idea of derivative came about to solve the problem of defining tangent lines to the graphs of functions. It also deals with calculating the slope of these tangent lines.
This chapter will introduce the prime concept of the integral calculus, which involves trying to find the area bounded by the graph of these functions and learn how integration and differentiation are interrelated, and integration is the reverse process of differentiation. In differentiation, we are given a function, and we need to figure out the differentiation of that function. Conversely, in integration, you are given the differential of a function, and we need to find the function.
7.2 Integration as a Reverse Process of Differentiation
In NCERT Solutions for Class 12 Chapter 7 Applications of Derivatives, you would learn how one can find the integral of a function given its derivative. The other name of integral is also anti-differentiation. You would understand terms like “arbitrary constant” which are varied to get different integrals of any given function. This “arbitrary constant” is also known as “constant of integration” and for any arbitrary real number K, ∫f(x) dx = F(x) + K. This is an indefinite integral. You will get acquainted with a lot of basic formulas and properties of the indefinite integral in this section and how it is interpreted geometrically.
This chapter would take you through a comparison between integration and differentiation and you will realize a few basics about these 2 parts of calculus like:
Differentiation and integration are operations on functions
They both satisfy linearity properties.
There could be functions that are neither differentiable nor integrable.
They differ in the sense that differentiation of a function (wherever it exists) is a unique function while integral is not.
7.3 Methods of Integration
In this part of Integration NCERT Integrals Class 12 Solutions, you will gain knowledge on other methods of finding integral apart from the method of inspection discussed in the above part. You would realize how this method is useful when an inspection is not suitable for many different kinds of functions. Here you will understand 3 different ways of integration i.e.:
By Substitutions
Using Partial Functions
By Parts
You would further learn how to integrate using trigonometric identities for trigonometric functions with the use of many known trigonometric identities like:
$Sin^2 \theta + Cos^2 \theta = 1$
$2 Sin \theta Cos \theta = Sin 2 \theta$
$2 Sin \theta_1 Cos \theta_2 = Sin (\theta_1 + \theta_2) + Sin (\theta_1 - \theta_2)$
7.4 Integrals of Some Particular Functions
In this part of NCERT Integrals Class 12 Solutions, you would learn many important integral formulas and how to apply them for many other standard integrals that are related to them. These short cut techniques provided in our solutions to problems on Integration Class 12 will help immensely in tackling many types of questions really fast. Understand how to prove these standard integrals, some of those are mentioned below:
∫ dx/ (x2 – b2) = 1/2b log |x – b/x + a| + C
∫ dx/ (x2 + b2) = 1/b tan-1 x/b + C
∫ dx/ (√x2 + b2) = log |x + √x2 + b2| + C
7.5 Integration by Partial Functions
This unit of Chapter 7 Class 12 Maths NCERT solutions further expands what you learned in unit 7.3 about integration by partial functions. In this section, you would learn how an improper rational function can be converted into a proper rational function by a long division process. An improper rational function is the one where for 2 polynomials p1(x) and p2(x) on x, p2(x) <>0 and degrees of p1(x) > p2(x) t.
Understand here how to disintegrate an equation into parts and find the integral of each of the parts. This is known as partial fraction decomposition.
7.6 Integration by Parts
This part would teach you methods on how to integrate products of functions. This method is also called partial integration. If for a single variable a, there are 2 differentiable functions f1 and f2, then apply the product rule of differentiation.
And apply integration on both sides and conclude that integral of the product of 2 functions is given by:
(1st function) * (integral of the 2nd function) – Integral of ((differential coefficient of 1st function) * (integral of the 2nd function))
7.7 Definite Integral
In this section, you will closely learn about what a definite integral is. You would learn how to denote a definite integral and its notation.
$\int_{a}{b} f(x) dx$
In this, a is the lower limit and b is the upper limit of the integral.
You can learn how to represent a definite integral either as a limit of a sum or an antiderivative in the interval (a, b).
7.8 Fundamental Theorem of Calculus
In this chapter, you would learn how concepts of integration and differentiation of a function are linked. This is done by calculating the anti-derivative difference at the upper and lower limits of the integration process.
7.9 Evaluation of Definite Integrals by Substitution
In this chapter, you would learn steps to evaluate definite integrals, by substitution as described below:
Reduce the given integral to a known form by replacing y = f(x) and x = q(y) (considering the integral without limits).
Now integrate the new integrand without mentioning the constant of integration.
7.10 Some Properties of Definite Integral
Here you will learn many properties of definite integrals which will simplify the process of evaluating definite integrals.
Overview of Deleted Syllabus for CBSE Class 12 Maths Chapter 7 Integrals
Chapter | Dropped Topics |
Integrals | Points (xi)–(xiii) in the List of Derivatives |
Exercise 7.2.1 : Geometrical Interpretation of Indefinite Integral | |
Exercise 7.2.3 : Comparison between Differentiation and Integration | |
Exercise 7.6.3 : Type of Integral | |
Exercise 7.7.1 : Definite Integral as the Limit of a Sum | |
Question Number 19, 32, 40 and 44 | |
Point 2 in the Summary | |
(xiv) and (xv) in Some Standard Integrals |
Class 12 Maths Chapter 7: Exercises Breakdown
Exercise | Number of Questions |
Exercise 7.1 | 22 Questions and Solutions |
Exercise 7.2 | 39 Questions and Solutions |
Exercise 7.3 | 24 Questions and Solutions |
Exercise 7.4 | 25 Questions and Solutions |
Exercise 7.5 | 23 Questions and Solutions |
Exercise 7.6 | 24 Questions and Solutions |
Exercise 7.7 | 11 Questions and Solutions |
Exercise 7.8 | 22 Questions and Solutions |
Exercise 7.9 | 10 Questions and Solutions |
Exercise 7.10 | 21 Questions and Solutions |
Miscellaneous Exercise | 40 Questions and Solutions |
Conclusion
The NCERT Solutions for Integration in Class 12 Math, provided by Vedantu, offer a comprehensive understanding of this crucial topic. These solutions simplify complex integration concepts into easy-to-understand language, making learning smoother. The key focus should be on grasping the fundamental concepts of integration and mastering problem-solving techniques. Understanding the various methods of integration, such as substitution, integration by parts, and partial fractions, is essential. Additionally, practising a variety of problems is crucial to reinforce learning. Looking at previous-year question papers, integration questions typically range from 6 to 8 in number, emphasizing the importance of thorough preparation in this area. Overall, utilizing these Integrals Class 12 Solutions effectively can greatly enhance one's proficiency in integration.
CBSE Class 12 Maths Chapter 7 Other Study Materials
S.No. | Important Links for Chapter 7 Integrals |
1 | |
2 | |
3 | |
4 |
NCERT Solutions for Class 12 Maths | Chapter-wise List
Given below are the chapter-wise NCERT 12 Maths solutions PDF. Using these chapter-wise class 12th maths ncert solutions, you can get clear understanding of the concepts from all chapters.
S.No. | NCERT Solutions Class 12 Maths Chapter-wise List |
1 | |
2 | |
3 | |
4 | |
5 | |
6 | |
7 | |
8 | |
9 | |
10 | |
11 | |
12 |
Related Links for NCERT Class 12 Maths in Hindi
Explore these essential links for NCERT Class 12 Maths in Hindi, providing detailed solutions, explanations, and study resources to help students excel in their mathematics exams.
S.No. | Related NCERT Solutions for Class 12 Maths |
1 | |
2 |
Important Related Links for NCERT Class 12 Maths
S.No | Important Resources Links for Class 12 Maths |
1 | |
2 | |
3 | |
4 | |
5 | |
6 | |
7 | |
8 | |
9 | |
10 |
FAQs on NCERT Solutions for Class 12 Maths Chapter 7 Integrals
1. What is integral in simple terms?
In Calculus, an integral is often referred to as the area under a curve. As mathematical functions can be represented on a graph paper, the area enclosed between the curve of a function and the x-axis is the integral value of that particular equation. You will come across a list of formulas for calculating the integration of various functions, in class 12 calculus. Integral is also known as anti-derivative and you may observe that the integration formulas for some functions are just the reverse of that of their differentiation formulas. Integral calculus is one of the most important topics of Class 12 Mathematics.
2. What is the difference between definite and indefinite integrals?
When the upper and lower limits are given for an integral, it is called a definite integral. While calculating the definite integration of a given function f(x), for upper limit a, and lower limit b, it must be noted that you are calculating the area under the curve f(x) from x=a to x=b. Unlike definite integrals, upper and lower limits are not provided for indefinite integrals. So, you calculate a generic integral value for a family of similar functions f(x), in indefinite integral, where ‘x’ can have a range of solutions. Also, there is a notation for additive constant, C, written along with the indefinite integral value of any function.
3. Is Class 12 integral calculus difficult to understand?
No, Class 12 integral calculus is not much difficult to understand, instead it is one of the most interesting topics in the Maths syllabus 12th boards. The basic sums of integration can be solved if you have a good knowledge of the integration formulas for various types of functions. Also, understanding the concepts of integral calculus becomes easier if you have proper knowledge of derivatives. Integration is also termed as the antiderivative. A good understanding of all the concepts of integral calculus introduced in Class 12 is very essential. These concepts lay the foundation of the theories of advanced mathematics and statistical studies.
4. Where can I find reliable NCERT Solutions for Class 12 Maths Chapter 7- Integrals online?
You can find reliable NCERT Solutions for Class 12 Maths Chapter 7 - Integrals on Vedantu. The NCERT solutions on Vedantu are prepared by our team of subject matter experts. All the sums of NCERT Class 12 Maths Chapter 7 are worked out in a stepwise manner so that students can verify their own solutions. Our subject matter experts have followed the updated CBSE guidelines for Class 12, for preparing the NCERT Maths solutions for integrals. You can download these NCERT solutions for free and you can refer to them for practice purposes.
5. What is the meaning of integrals in NCERT Class 12 Maths Chapter 7?
Integrals in Mathematical language can be described as a generalization of area or area under a curve of a function. They come under the topic of calculus along with derivatives. It can also be represented as a numerical value. Learning about integrals and derivatives can be fun and interesting but at the same time, thorough practice is needed as integrals are often very tricky and there can be multiple ways of solving the same problem.
6. Where can I download the NCERT Solutions for Class 12 Maths Chapter 7 PDF online?
To download the solutions for Class 12 Maths Chapter 7, follow the steps mentioned below:
Visit the page-NCERT Solutions for Class 12 Maths.
Choose the chapter of your choice, here, it is Chapter 7
The webpage with Vedantu’s solutions for Class 12 Maths Chapter 7 will open.
To download this, click on the Download PDF button and you can view the solutions offline free of cost.
For more efficient doubt clearing sessions or other help, one can visit the Vedantu site and avail their help for cracking exams and achieving good marks. The students are advised to first try out the exercises based on their knowledge and practice before referring to the solution so that they can get a clear idea as to where they are lacking behind.
7. Why are integrals so hard?
If any student skips any topic or finds it hard to grasp the basic idea of any topic, integrals could be a nightmare for that student. Hence, it is crucial that you practice all different types of sums and get used to the different difficulty levels of the questions. Practice as many sums as you can to completely understand any topic.
8. What do you mean by differentiation?
Differentiation is a technique for determining a function's derivative. Differentiation is a mathematical procedure for determining the instantaneous rate of change of a function depending on one of its variables. The most prominent example is velocity, which is the rate of change of displacement with respect to time. It is an important topic and hence must be practised judiciously.
9. What are definite and indefinite integrals?
In cases when the lower and upper bounds are constants, the number can be represented or solved by a definite integral. There are several functions whose derivatives are represented by the indefinite integral, and they are called f's. Any two functions in the same family will always be distinct from each other. For a detailed explanation of the same, you can visit Vedantu website and the app.
10. How many types of integration are there in Chapter 7 Maths Class 12?
The types of integration are:
Integration by Parts
Method of Integration Using Partial Fractions
Integration by Substitution Method
Integration by Decomposition
Reverse Chain Rule.
11. What is the integral symbol called (Chapter 7 Maths Class 12)?
The integral symbol is the elongated "S" which you may have seen or represented as ∫.
12. Who is the father of integration?
Isaac Newton: He basically invented integration alongside differentiation (think opposite processes).
Gottfried Wilhelm Leibniz: He created a widely used notation system for writing integrals.
13. What is the rule of Integral Class 12 Maths Chapter 7?
The integration rule is defined in four types.
The basic rules are:
Constant
Variable
Square
Reciprocal
Exponential
Trigonometry
14. What is Integration all about?
Area under a curve is called Integration. Imagine a curvy line on a graph. Integration helps you find the exact area under that curve, like measuring the exact area of a weirdly shaped field which represents the Integration.
15. Are there any formulas to learn in Integration Class 12 NCERT Solutions?
Yes! Integration involves various formulas, kind of like having a toolbox with different tools for different problems. You will learn formulas for different functions and techniques.
16. Are there any tricks involved?
Yes , to learn special techniques like substitution or using trigonometric identities to solve trickier integral problems.
17. What is covered in Class 12 Maths Chapter 7?
The definition of integration, its relationship to differentiation, integration techniques, substitution methodology, integration of specific functions, integration of partial functions, and definite and indefinite integrals are the main aspects of this chapter.
18. Are there different types of integration problems?
There are two main types in class 12 integration
Indefinite Integrals: Finding a general expression for the area under the curve (like estimating total distance traveled).
Definite Integrals: Calculating the exact area within specific boundaries (like the amount of juice in a weird bottle).
19. Do I need to memorize a bunch of formulas in Integrals class 12 solutions?
Integration uses different approaches depending on the problem, like having a toolbox with various tools. NCERT solutions can help you grasp these techniques and their applications.
20. Are there any tricks to solving integration problems?
Absolutely, there are few special techniques like:
Substitution: Replacing parts of the integral with new variables.
Integration by Parts: A specific method for integrating certain functions.
Trigonometric Identities: Using trigonometric properties to simplify integrals.
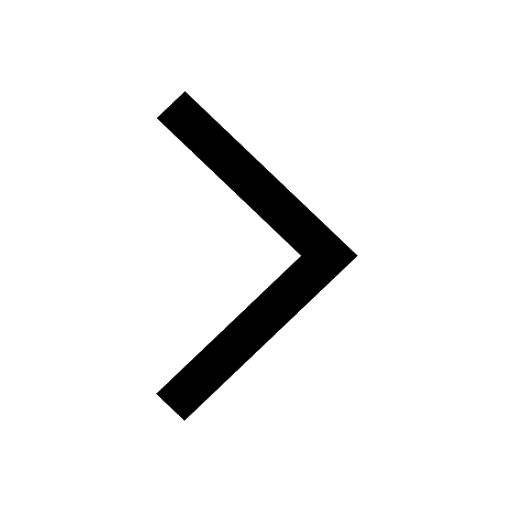
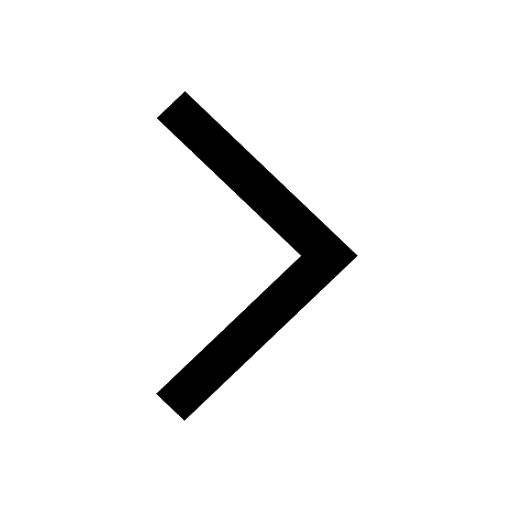
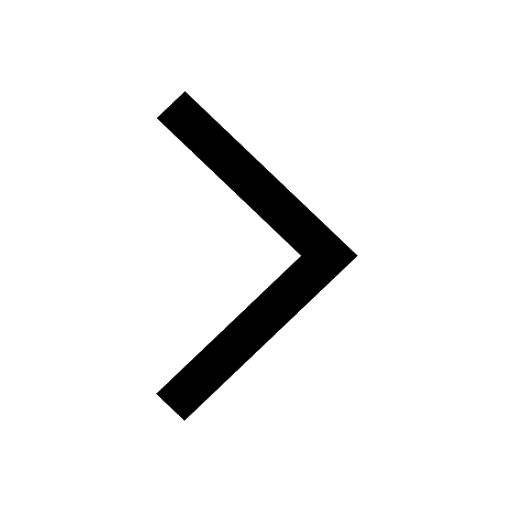
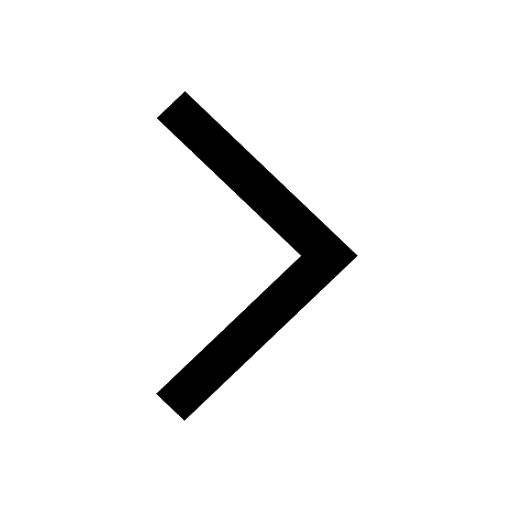
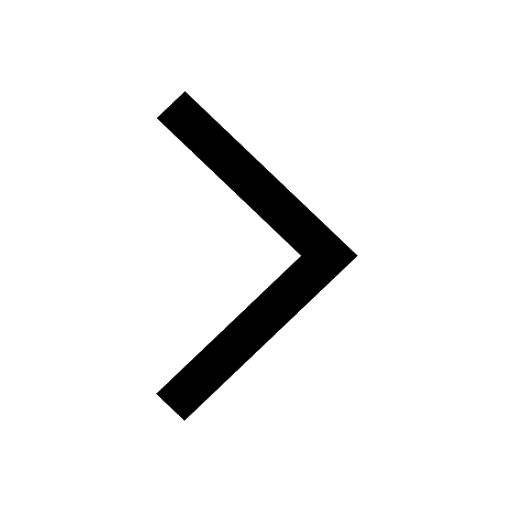
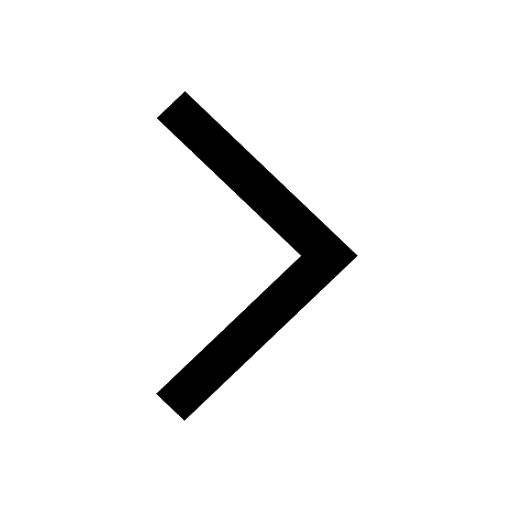
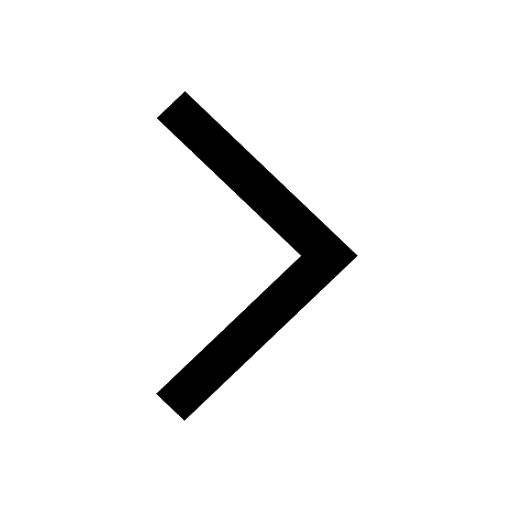
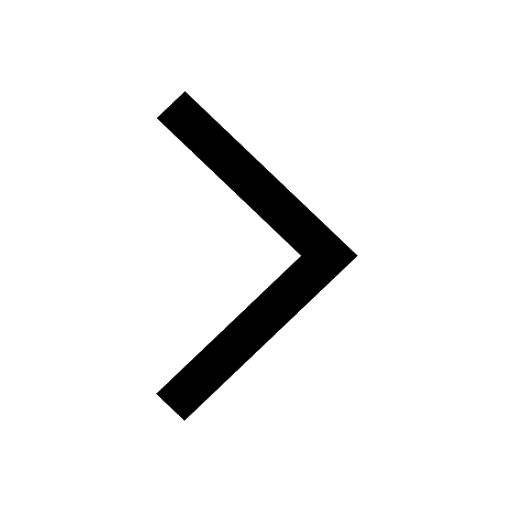
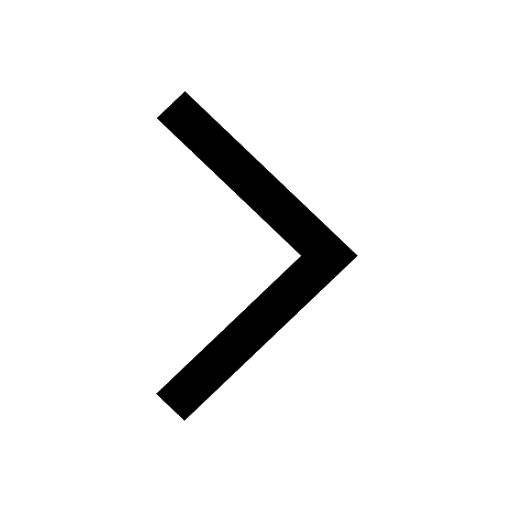
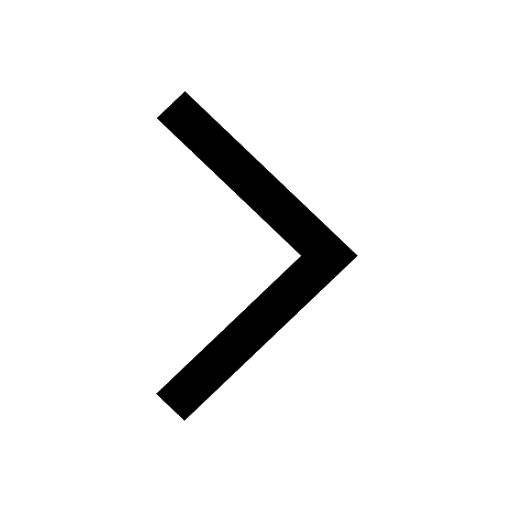
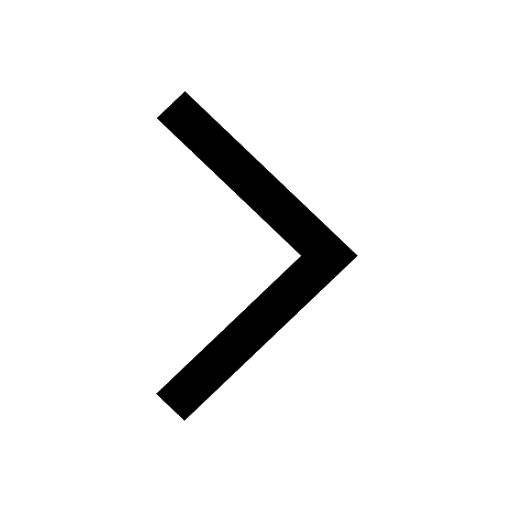
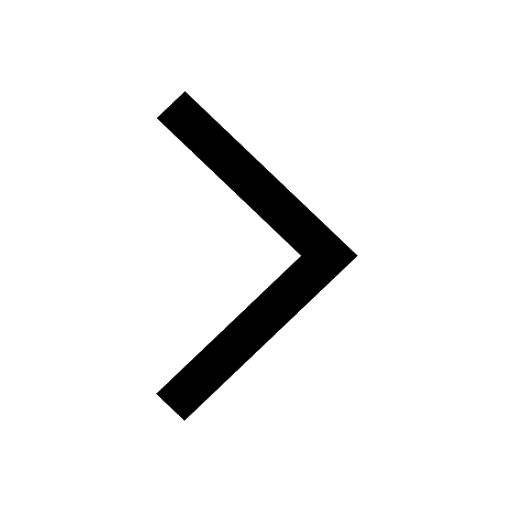
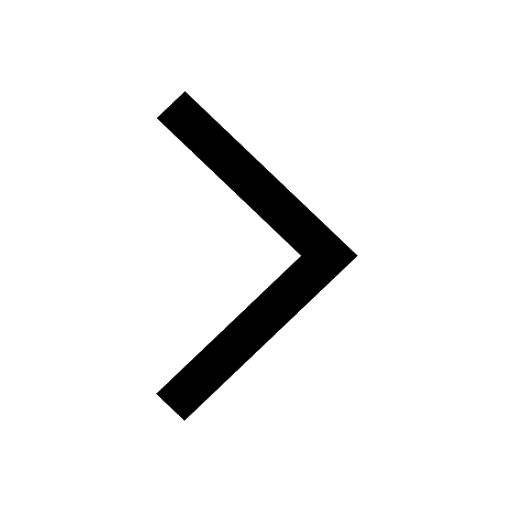
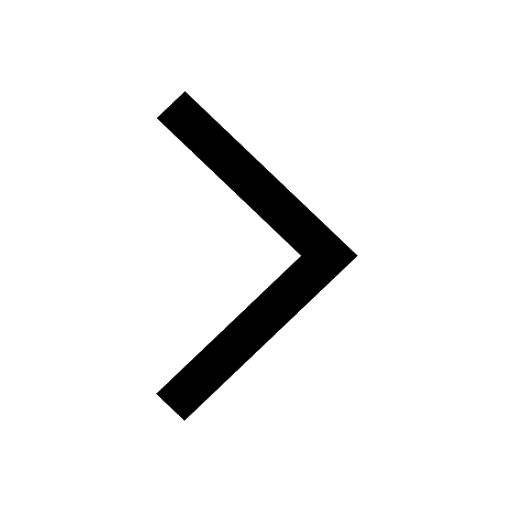
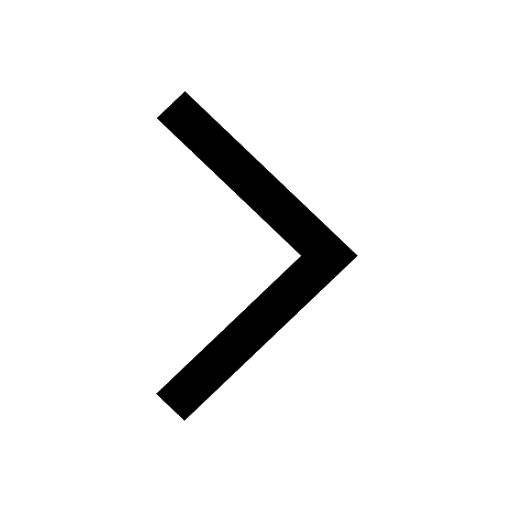
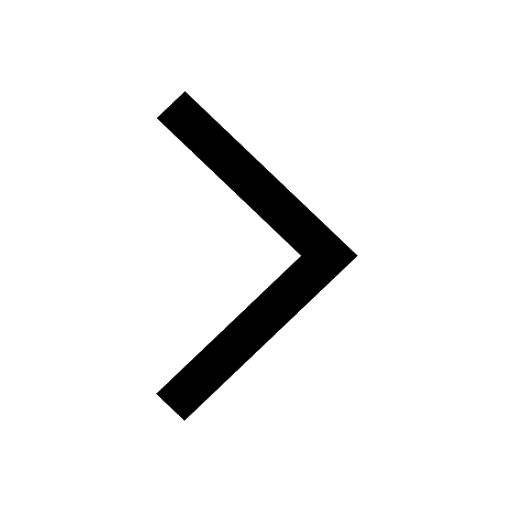
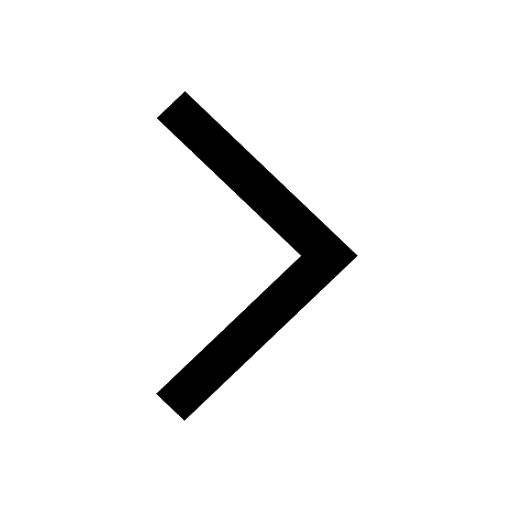
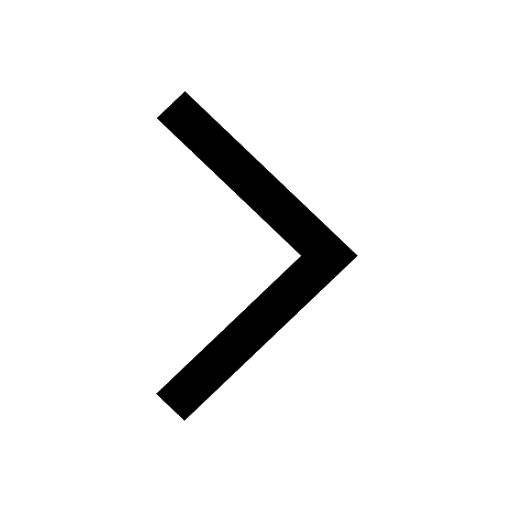
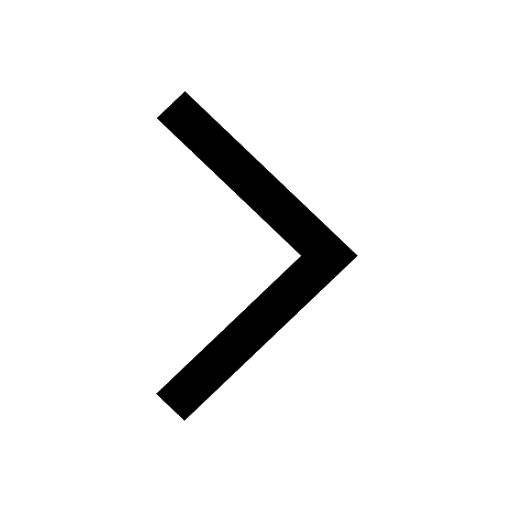
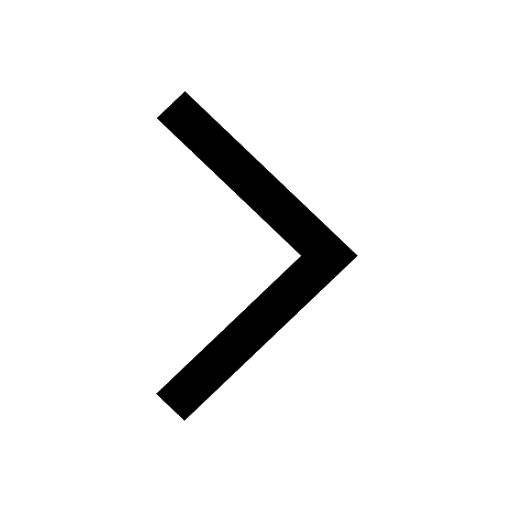
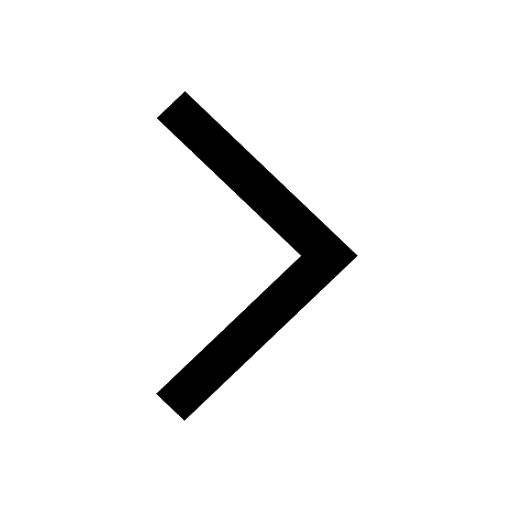