Revision Notes on D and F Block Elements Class 12 NEET 2024 - Free PDF Download
Students in class 12 will come across the Chemistry chapter of d and f Block Elements, which talks about the group of 3 to 11 called transition elements and inner transition elements. Students using class 12 Chemistry D and F Block Elements notes will learn about the D block elements having partially filled (N-1) d-orbitals. The position of a part in the periodic table reflects its qualities and nature. The D and F Block Elements class 12 notes created by subject experts of Vedantu help in understanding the topic and methods to study.
Note: 👉Explore Your Medical College Options with the NEET Rank and College Predictor 2024.
The class 12 Chemistry D and F Block Elements notes carry all the elements required for preparing for the NEET exam, which students find pretty tough. They will clearly understand the chapter and work on the revision.
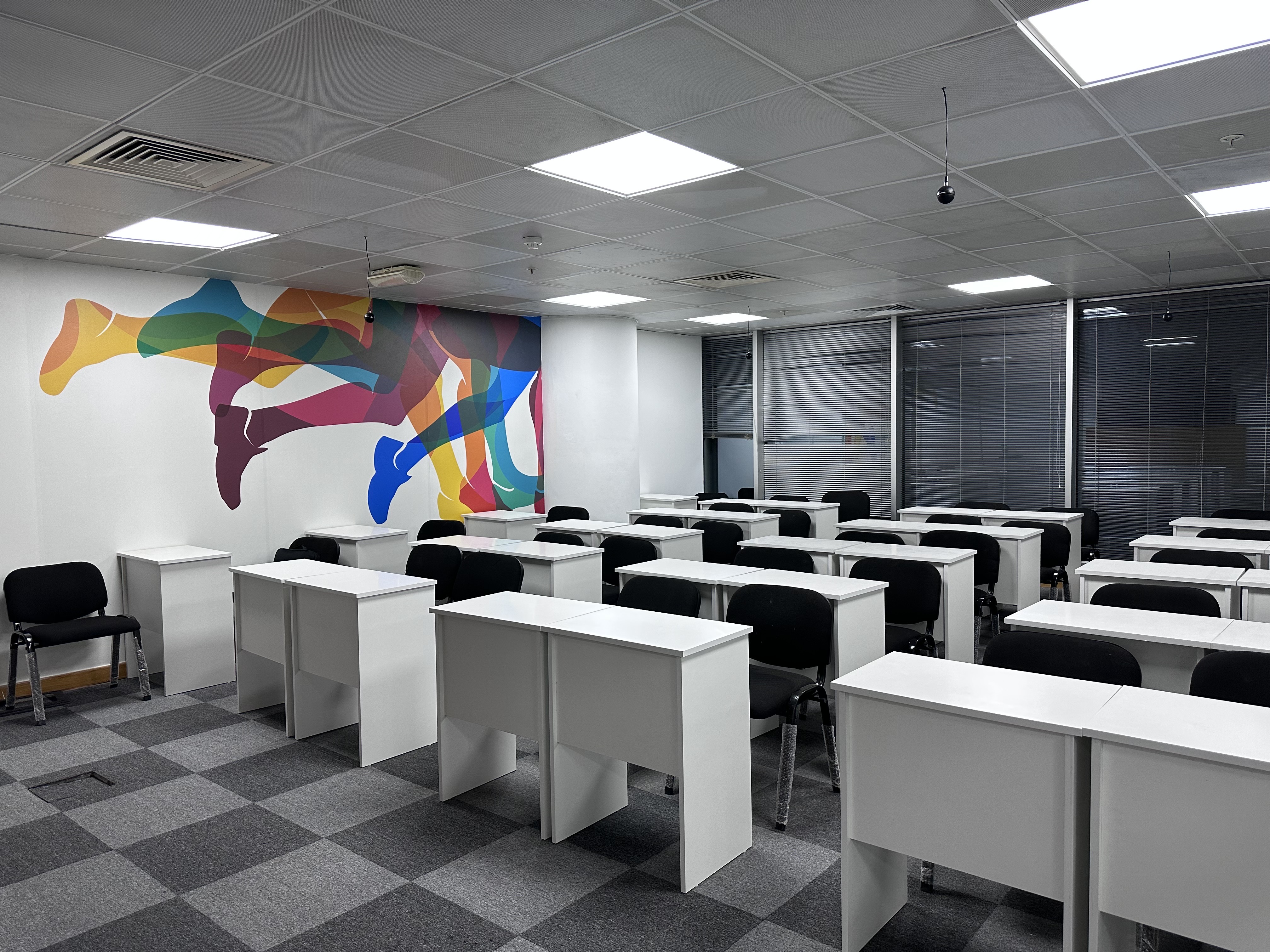
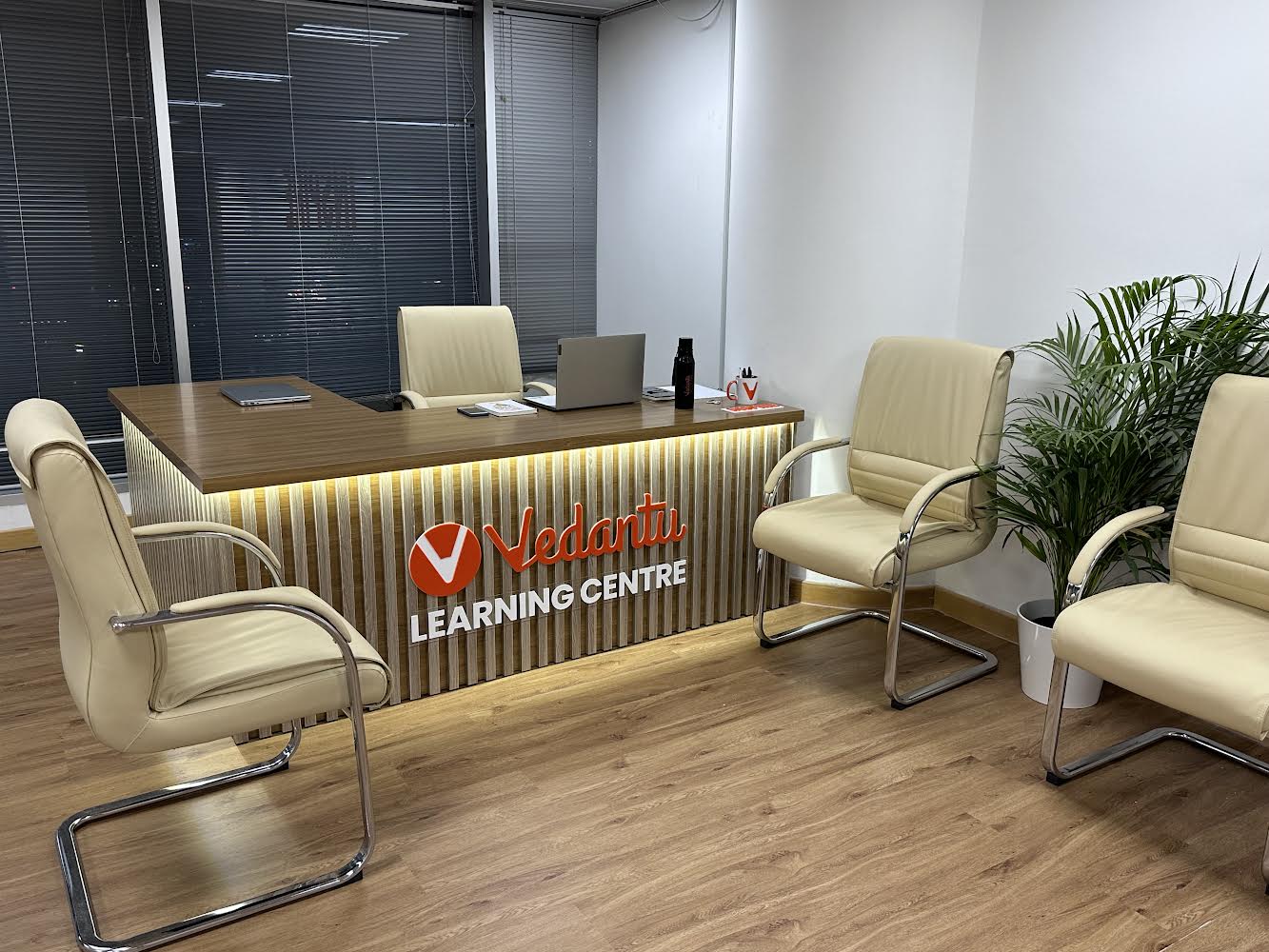
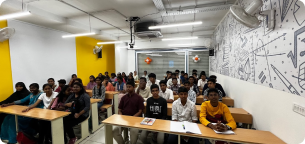
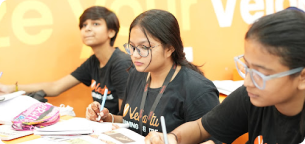
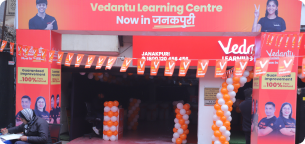
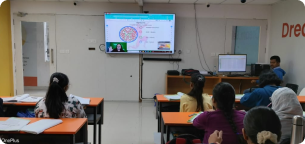
NEET Revision Notes Chemistry d and f Block Elements
Definition
"d-block elements" are those in which the last coming electron enters the d-orbitals of the penultimate shell, i.e. (n–1) d, where n is the last shell. A transition element is defined as an element with a partially filled d-orbital, in its ground state or most stable oxidation state.
These elements have attributes that are halfway between those of s-block and p-block elements.
These elements represent a shift in attributes from more electropositive (s-block) to less electropositive (p-block) elements. As a result, these elements are referred to as transition elements.
Position in the Periodic Table
In the periodic table, the location of the d-block element is as follows.
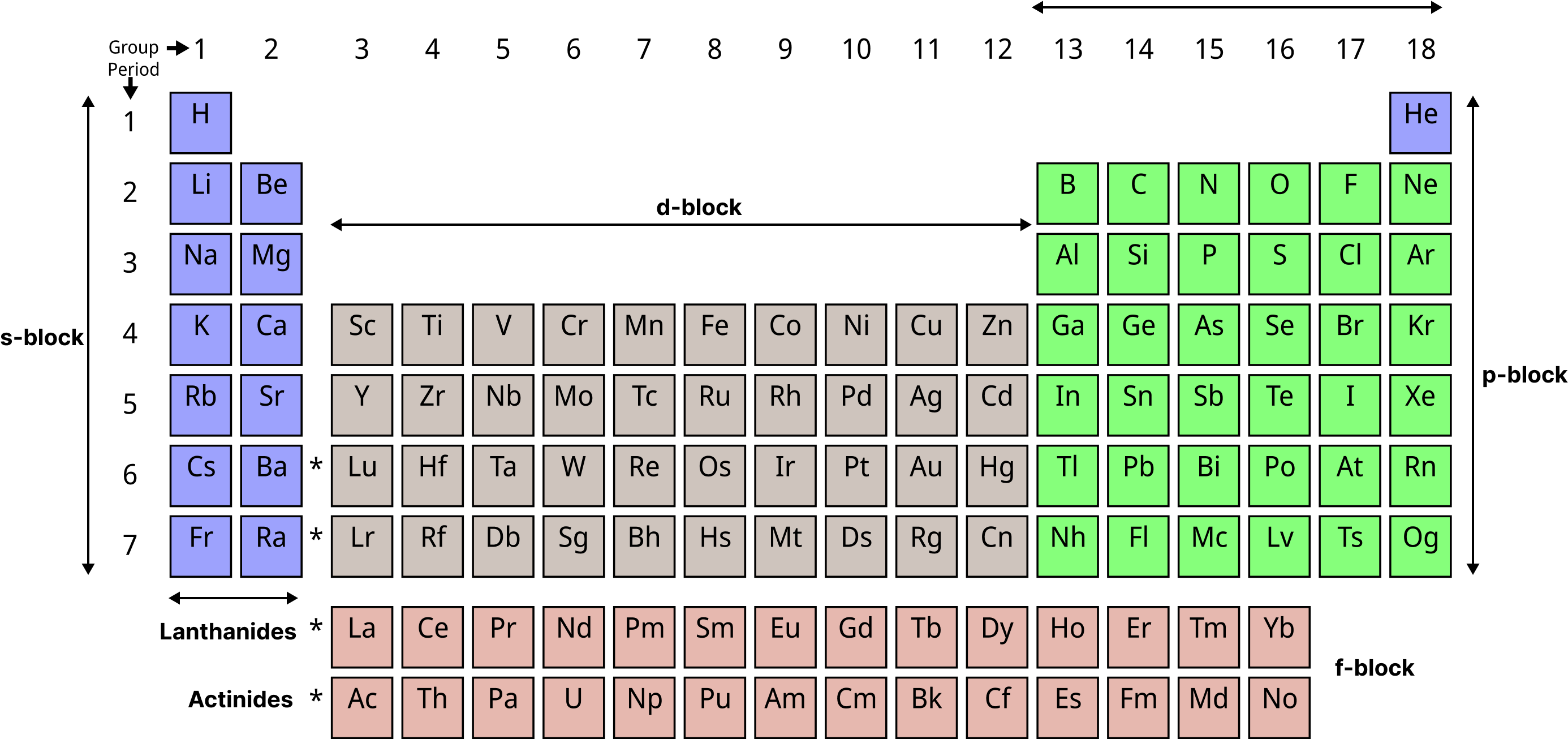
Periodic Table
(1) d-block elements are positioned between the 's' and 'p' block elements in the periodic table, i.e. they are in the middle of the table.
(2) There are d-block elements in
4th period (
5th period (
6th period (
7th period (
Electronic Configuration
(1) As the atomic number of a d-block element increases, electrons fill the dorbitals of the penultimate shell, (n-1) d1-10, ns0-2 is the standard electrical configuration of the d-block element.
(2) Four rows (called series) of 10 elements each are obtained depending on which penultimate shell's d-orbitals are filled, i.e. n = 4, 5, 6, 7. They correspond to the subshells 3d, 4d, 5d, and 6d.
(3) Because the energy of the '(n–1)d' subshell is somewhat higher than that of the 'ns' subshell, the orbital is filled first, followed by the (n – 1) d orbitals.
(4) The following is the general electronic configuration of d-block/d-series elements:
1. First (3d) Transition Series (Sc–Zn)
Atomic no. | 21 | 22 | 23 | 24 | 25 | 26 | 27 | 28 | 29 | 30 |
Element | Sc | Ti | V | Cr | Mn | Fe | Co | Ni | Cu | Zn |
E.C |
2. Second (4d) Transition Series (Y-Cd)
Atomic no. | 39 | 40 | 41 | 42 | 43 | 44 | 45 | 46 | 47 | 48 |
Element | Y | Zr | Nb | Mo | Tc* | Ru | Rh | Pd | Ag | Cd |
E.C |
3. Third (5d) Transition Series (La–Hg)
Atomic no. | 57 | 72 | 73 | 74 | 75 | 76 | 77 | 78 | 79 | 80 |
Element | La | Hf | Ta | W | Re | Os | Ir | Pt | Au | Hg |
E.C |
4. Fourth (6d) Transition Series
Atomic no. | 89 | 104 | 105 | 106 | 107 | 108 | 109 | 110 | 111 | 112 |
Element | Ac | Rf | Db | Sg | Bh | Hs | Mt | Ds | Rg | Uub |
E.C |
Exceptional configuration of Cr and Cu:
The electrical configurations of chromium (Z = 24) and copper (Z = 29) are the exceptions in the first series.
It's worth noting that chromium and copper, unlike other elements, have a single electron in the
orbital.This is due to the atom gaining additonal stability by either having a half-filled configuration (i.e., containing 5 electrons in the d-sublevel) or a totally filled arrangement (i.e., the d-sublevel contains 10 electrons). In the case of chromium, the 3d-level is exactly half-filled with configuration
, but in the case of copper, it is totally filled with configuration .
Based on exchange energy, this can be explained.
Thus, chromium (Z = 24) and copper (Z = 29) have the electrical arrangement.
Properties
1. Atomic Radii
(i) Transition metals have atomic radii that are halfway between those of s- and p-block elements.
(ii) In general, the atomic radii of d-block elements in a series fall as the atomic number increases, but the atomic size reduces only little after midway.
(iii) At the end of the period, the atomic radii show a modest increase.
(iv) As you move down the group, the atomic radii get smaller. As a result, the atomic radii of the second transition series are bigger than those of the first. However, the second and third transition series' atomic radii are nearly identical.
Because of lanthanide contraction (also known as lanthanoid contraction), the atomic radii of the elements of the second and third transition metals are almost identical.
Ionic Radii
(i) The ionic radii follow the same pattern as the atomic radii.
(ii) In different oxidation states, transition metals have variable ionic radii.
(iii) The transition metals' ionic radii are less than those of typical elements from the same period.
Metallic Character
All the transition elements, except for mercury, which is a liquid, have a conventional metallic structure. They have all the properties of metals.
High melting and boiling temperatures, high thermal and electrical conductivity, and high tensile strength characterise ductile materials.
Transition elements have one or two electrons in their outermost energy level (
) and have low ionisation energies. Metallic bonds are created as a result.As a result, they behave like metals. Due to the overlapping of unpaired electrons between distinct metal atoms, the greater the number of unpaired d electrons, the stronger the bonding.
Melting Point
Because of their strong metallic connection, transition metals have high melting points. The link between metals.
The quantity of unpaired e– determines the metallic bonding. The melting point rises (Sc-Cr), reaches a maximum (Cr), and then falls (Sc-Cr) (Fe-Zn)
Tungsten (W) has the highest melting point, while mercury (Hg) has the lowest.
Ionisation Energies or Ionisation Enthalpies
(i) D-block elements' first ionisation enthalpies are located between s-block and p-block elements. They are higher than s block elements but lower than p-block elements.
Along a particular transition series, the ionisation enthalpy gradually increases with increasing atomic number, albeit there are occasional exceptions.
(ii) The difference in ionisation enthalpies between any two-consecutive d-block elements in a given series is substantially smaller than the difference between successive s-block or p block elements.
(iii) However, because of the fully filled (n-1)
(iv) While second and third ionisation enthalpies increase over time in general, the degree of the increase for consecutive elements is substantially greater.
Oxidation State
"The Oxidation state or Oxidation number refers to the net numerical charge ascribed to an atom of an element in its combined state."
(1) With the exception of a few elements, majority of d-block elements have multiple oxidation states, or varying oxidation states. Because of the following factors, the oxidation status of the elements varies:
(i) Because the energies of the '(n-1) d' and 'ns' orbitals in the atoms of d-block elements are nearly identical, electrons from '(n-1)d' orbitals can be extracted as easily as electrons from 's' orbitals.
(ii) The residue after eliminating the ‘s' electrons is known as the Kernel of the metal cations. The kernel in d-block elements is unstable, therefore one or more electrons from (n – 1)d electrons are lost. As a result, cations with various oxidation states are formed.
(2) Except for the last element in each series, all transition elements have a changeable oxidation state.
(3) The total number of electrons lost in 4s is the minimum oxidation state. (Total number of electrons in 4s + number of unpaired electrons in 3d lost) = maximum oxidation state
All elements in the '3d' series have two electrons in '4s,' hence they all have the same minimum oxidation state of +2.
(With the exception of '
(4) Ruthenium (Ru) and Osmium (Os) have the greatest oxidation state, which is +8.
(5) In metal carbonyl complexes, transition metals also have zero oxidation states. (Nickel tetracarbonyl)
(6) Bonding in lower oxidation state (+2, +3) compounds is primarily ionic, while bonding in higher oxidation state compounds is mostly covalent.
(7) The extra stability rule, according to which
The stability order of various ions, for example, is as follows:
(8) Due to the high heat of hydration,
The
Oxidation States
Electronic Configuration | ||||||||||
+2 | +2 | +2 | +2 | +2 | +2 | +2 | +1 | +2 | ||
+3 | +3 | +3 | +3 | +3 | +3 | +3 | +3 | +2 | ||
+4 | +4 | +4 | +4 | +4 | +4 | +4 | ||||
+5 | +5 | +5 | ||||||||
+6 | +6 | +6 | ||||||||
+7 |
Standard electrode potentials (
The magnitude of ionisation enthalpies of metals can be used to determine the thermodynamic stability of transition element compounds – the smaller the ionisation enthalpy of the metal, the more stable the molecule.
The stability of the compounds in solution is determined by electrode potentials rather than ionisation enthalpies.
Trends in the
(i) The Eo (
(ii) The increase in the sum of first and second ionisation enthalpies is responsible for the general trend towards smaller negative Eo values throughout the run.
(iii) Copper is the only metal in the series with a positive Eo value, making it stand out. This explains why acids do not release hydrogen gas. It only reacts with oxidising acids that have been reduced (
The total of the enthalpies of sublimation and ionisation is not balanced by the hydration enthalpy, which is why copper has a positive Eo value.
Trends in the
(i) The stability of the
(ii) The greatest Zn value is due to the extremely high stability of the
(iii) The relatively high value of Eo(
Values and Chemical Reactivity: The chemical reactivity of transition metals varies greatly. Some are extremely electropositive and dissolve in mineral acids, whereas others are 'noble,' meaning that they do not react with simple acids. The following are some chemical reactivity of transition metals as a function of their
(i) The first transition series metals (excluding copper) are more reactive than the other series metals. As a result, they are oxidised by H+ ions, though at a slow rate; for example, at ambient temperature, Ti and V are passive to dilute non-oxidizing acids.
(ii) As previously stated, as the series progresses, smaller negative Eo values for
(iii) More negative Eo values than expected for Mn, Ni, and Zn indicate that
Catalytic Property
(a) they have a changeable oxidation state, most transition elements and their compounds have high catalytic characteristics.
(b) They give the reactant a lot of surface area to absorb.
Catalysts | Uses |
Ziegler-Natta catalyst, used in polymerisation of ethylene | |
Contact process | |
Haber Bosch process | |
Wacker’s process | |
Hydrogenation of alkene, alkyne etc | |
Pt/PtO2 | Adam’s catalyst for selective reduction |
Catalytic convertor, for cleaning, car exhaust fumes | |
Ostwald’s process | |
Oxidation of alcohols |
Magnetic Behaviour
The orbital angular momentum or orbital spin of the electron is also a factor. It results in the formation of a magnetic moment.
The orbital angular magnetic moment is small in the first transition elements series because the orbital contribution is quenched by the electric fields of the surrounding atoms, therefore the magnetic moment is only equivalent to the spin magnetic moment.
= no. of paired electrons
Because of the presence of unpaired electrons, most transition elements and compounds are paramagnetic.
Complex Formation
Transition metal ions can be found in a wide range of complex molecules. Compounds with a metal ion coupled to several negative ions (anions) or neutral molecules with lone pairs of electrons are known as complex compounds. Ligands are ions or molecules that bind to other ions or molecules. They create coordinate bonds by donating lone pairs of electrons to the core transition metal ion.
Following are a few instances.
s- and p-block components do not create such complex compounds.
Explanation: Because of the following causes, transition elements form complexes:
(i) Their metal ions are smaller in comparison.
(ii) Their ionic charges are extremely high.
(They have a large charge/size ratio because (i) and (ii))
(iii) The presence of unoccupied d-orbitals that can take lone pairs of electrons given by the ligands.
Interstitial Compounds
Small atoms such as hydrogen, carbon, boron, and nitrogen fill the empty spaces (interstitial sites) in the lattices of transition metals, forming a huge number of interstitial compounds.
(i) They are extremely hard and stiff, such as steel, which is a very hard interstitial combination of Fe and C. Some borides are also as hard as diamond.
(ii) They have melting points that are higher than pure metal melting values.
(iii) They have conductivity comparable to that of pure metal.
(iv) They become chemically inert.
Alloy Formation
Alloys are homogenous solid solutions of two or more metals formed by melting and cooling the components. Metals with atomic radii that differ by no more than 15% produce these, allowing the atoms of one metal to easily take up positions in the crystal lattice.
Because transition metals have comparable atomic radii and other properties, they easily combine to create alloys.
Alloys are tougher, have greater melting temperatures, and are more corrosion resistant than individual metals.
The metals chromium, vanadium, molybdenum, tungsten, and manganese are used to make alloy steels and stainless steels, which are the most often utilised.
Alloys with non-transition metals are also quite prevalent. Brass (Cu + Zn) and bronze (Cu + Sn) are two examples.
Compounds of Transition Elements
Oxides
(i) At high temperatures, the metals of the first transition series produce oxides with oxygen.
(ii) Oxides are generated during oxidation in states +1 to +7
(ii) In general, metal oxides in lower oxidation levels are basic, while oxides in higher oxidation states are acidic, and oxides in intermediate oxidation states are amphoteric.
As a result,
The following are the many steps involved in the formation of potassium dichromite:
(i) Sodium chromate preparation:
Chromite ore Sod. chromate Ferric oxide
(ii) Sodium chromate to sodium dichromate conversion.
Sod.chromate (conc.) Sod. Dichromate
(iii) Sodium dichromate to potassium dichromate conversion:
Sodium dichromate Potassium dichromate
The effect of alkalies: When an alkali is added to an orange red dichromate solution, chromate forms, resulting in a yellow solution.
Due to the reformation of dichromate, the colour shifts back to orange red when acidified.
Or
The fact that the
The H+ ions are used up when an alkali (i.e., increasing the pH of the solution) is added, and the reaction proceeds in the forward direction, creating a yellow chromate solution, according to the rule of chemical equilibrium. When an acid is added (i.e., the pH of the solution is reduced), the concentration of H+ ions increases, and the reaction proceeds in the opposite direction, generating an orange red dichromate solution.
Potassium dichromate’s ability to oxidise: It has a strong oxidising effect. One molecule of potassium dichromate provides three atoms of accessible oxygen in the presence of dilute sulfuric acid, as shown by the equation:
Or
It frees
Structures of Chromate and Dichromate Ions.
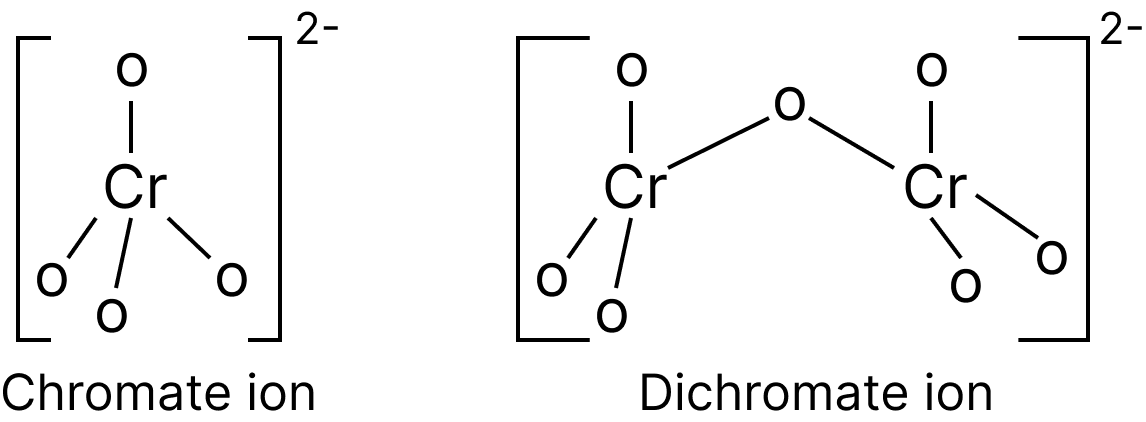
Structure of Chromate and Dichromate Ions
Potassium Permanganate (
Preparation
It is made on a massive scale from the mineral pyrolusite (
(i)
If left to remain for a while, potassium manganate undergoes disproportionation in the neutral or acidic solution as follows:
Potassium permanganate has oxidation properties.
In Acid Solutions:
Iodine is liberated from potassium iodide :
10I– + 2MnO4– + 16H+ → 2Mn2+ + 8H2O + 5I2
In neutral or faintly alkaline solutions:
A notable reaction is the oxidation of iodide to iodate:
2MnO4– + H2O + I– → 2MnO2 + 2OH– + IO3–
Lanthanoide Series
1. Introduction
The filling up of the antipenultimate 4f energy levels classifies these components.
2. Physical Properties
Electronic Configuration and oxidation states
Element | Electronic Configuration | Electronic Configuration of | Oxidation States |
Lanthanum La | 5d1 6s2 | 4f 0 | + 3 |
Cerium Ce | 4f15d16s2 | 4f 1 | + 3 (+ 4) |
Praseodymium Pr | 4f 3 6s2 | 4f 2 | + 3 (+ 4) |
Neodymium Nd | 4f 4 6s2 | 4f 3 | (+2) + 3 |
Promethium Pm | 4f56s2 | 4f 4 | (+2) + 3 |
Samarium Sm | 4f 6 6s2 | 4f 5 | (+2) + 3 |
Europium Eu | 4f 76s2 | 4f 6 | (+ 2) + 3 |
Gadolinium Gd | 4f 7 5d1 6s2 | 4f 7 | + 3 |
Terbium Tb | 4f9 6s2 | 4f 8 | + 3 (+ 4) |
Dysprosium Dy | 4f106s2 | 4f 9 | + 3 (+ 4) |
Holmium Ho | 4f116s2 | 4f 10 | + 3 |
Erbium Er | 4f126s2 | 4f 11 | + 3 |
Thulium Tm | 4f136s2 | 4f 12 | (+ 2) + 3 |
Ytterbium Yb | 4f146s2 | 4f 13 | (+ 2) + 3 |
Lutetium Lu | 4f145d1 6s2 | 4f 14 | + 3 |
Oxidation State
The total of each element's first three ionisation energies is low. As a result, the oxidation state (+III) is ionic, and the chemistry of these elements is dominated by
Numbers of oxidation (+II) and (+IV) do occur, especially when they lead to:
1. a noble gas configuration (for example,
2. a partially filled f shell, such as
3. a fully filled f level, such as
Atomic Radius
The atomic and ionic radii of trivalent ions decrease with increasing atomic number in the lanthanide series, from
The nuclear charge grows by one unit every element as we progress through the lanthanide series. The new electron is inserted into the same subshell as the previous one (viz., 4f).
As a result, the nucleus's attraction to electrons increases, and the size tends to shrink. Furthermore, because to the geometries of these f-orbitals, there is incomplete shielding of one electron by another in the f-subshell as a new electron is added.
This insufficient shielding is inadequate to counteract the increasing nuclear charge's effect.
As a result, despite the minor decrease in size, the total result is a reduction in size.
It's worth noting that the drop in atomic radius for 14 elements in the lanthanides (Ce (58) to Lu (71)) is only 11pm (from 183 to 172 pm).
Similarly, the ionic radius drops from
Atomic and Ionic radii (pm) of lanthanum and lanthanoids.
Element | La | Ce | Pr | Nd | Pm | Sm | Eu | Gd | Tb | Dy | Ho | Er | Tm | Yb | Lu |
Atomic Radii (Ln) | 187 | 183 | 182 | 181 | 181 | 180 | 199 | 180 | 178 | 177 | 176 | 175 | 174 | 173 | 172 |
Ionic Radii (Ln3+) | 106 | 103 | 101 | 99 | 98 | 96 | 95 | 94 | 92 | 91 | 90 | 89 | 88 | 87 | 86 |
Magnetic Behaviour
Except for
The presence of unpaired electrons in the incomplete 4f subshell gives lanthanides this feature.
The magnetic moments of lanthanides differ from those of transition elements in that they do not satisfy the spin only formula, namely
As a result, their magnetic moment is estimated by considering both spin and orbital contribution.
The spin quantum number is S, while the orbital quantum number is L.
Colour
The Lanthanides are a group of metals that are silvery white in colour. In the solid form and in aqueous solution, however, most trivalent metal ions are coloured. This is due to partially filled f orbitals, which allow for f-f transitions.
Actinide Series
Elements and their Oxidation States
Atomic | Element | Symbol | Outer electronic structure | Oxidation states |
89 | Actinium | Ac | +3 | |
90 | Thorium | Th | +3, +4 | |
91 | Protactinium | Pa | +3, +4, +5 | |
92 | Uranium | U | +3, +4, +5, +6 | |
93 | Neptunium | Np | +3, +4, +5,+ 6, +7 | |
94 | Plutonium | Pu | +3, +4, +5,+ 6, +7 | |
95 | Americium | Am | +2, +3, +4, +5, +6 | |
96 | Curium | Cm | +3, +4 | |
97 | Berkelium | Bk | +3, +4 | |
98 | Californium | Cf | +3 | |
99 | Einsteinium | Es | +3 | |
100 | Fermium | Fm | +3 | |
101 | Mendelevium | Md | +3 | |
102 | Nobelium | No | +3 | |
103 | Lawrencium | Lr | +3 | |
104 | Rutherfordium | Rf | +3 |
1. Oxidation State
Actinides, unlike Lanthanides, have a vast variety of oxidation states. This is due to the minor energy difference between the 5f, 6d, and 7s subshells. As a result, all their electrons can participate in the production of bonds.
These elements have a prevailing oxidation state of +3. (like Lanthanides). Actinides have an oxidation state of +4 in addition to the +3 state.
The oxidation states of some Actinides are even higher. The maximal oxidation state increases until the middle of the series, then falls; for example, it increases from + 4 for Th to +5, +6, and +7 for Pa, U, and Np, then declines for the following elements.
Actinides are like Lanthanides in that they have more compounds in the +3 state than the +4 state. The compounds in the +3 and +4 states, on the other hand, are more likely to be hydrolyzed.
It should also be emphasised that the distribution of oxidation states in Actinides is so unequal that discussing their chemistry in terms of oxidation states is pointless.
2. Atomic radius
Due to the poor shielding effect of the 5f-electrons, the Actinides show Actinide contraction (which is very similar to Lanthanide contraction).
As a result, the radii of these metals' atoms or ions drop in a predictable pattern across the series. Due to inferior shielding by 5f electrons, the shrinkage is stronger from element to element in this sequence.
This is since 5f orbitals extend beyond 6s and 6p orbitals in space, but 4f orbitals are buried deep within the atom.
3. Magnetic behaviour
The Actinides elements, like Lanthanides, are extremely paramagnetic. The variation in magnetic susceptibility of Actinides as the number of unpaired electrons increases is comparable to that of Lanthanides, however the values for Actinides are larger than those for Lanthanides.
4. Colour
These metals have a silvery white colour to them. Actinide cations, on the other hand, are usually coloured. The number of 5f-electrons determines the cation's colour. Colourless cations have no 5f-electron or have seven 5f-electrons (i.e., exactly half-filled f-subshell). Both in the crystalline state and in aqueous solution, cations with 2 to 6 electrons in the 5f-subshell are coloured. The colour is caused by the f–f transition.
Comparison of Lanthanides and Actinides
Similarities:
Because both Lanthanides and Actinides involve the filling of f-orbitals, they are comparable in many ways:
(i) Both have a predominantly +3 oxidation state.
(ii) They're both electropositive and incredibly reactive.
(iii) They have magnetic and spectral features in common.
(iv) Actinides have contractile properties like Lanthanide
The Following are Some of The Distinctions in Their Characteristics:
Lanthanides | Actinides |
(i) They only exhibit + 2 and +4 oxidation states in a few circumstances, in addition to a + 3 oxidation state. | (i) They also display higher oxidation states of +4, +5, +6, + 7 in addition to the +3 oxidation state. |
(ii) The majority of their ions are colorless; | (ii) The majority of their ions are coloured |
(iii) They have a lower tendency for complex formation | (iii) They have a higher tendency for complex creation. |
(iv) Lanthanide compounds have lower basicity | (iv) Actinides are more basic |
(v) Avoid forming oxocation | (v) They are made up of oxocations. Eg. |
(vi) They are non-radioactive, except for promethium | (vi) They are radioactive. |
(vii) Their magnetic properties are simple to explain. | (vii) Because their magnetic properties are more complex, they are difficult to explain. |
Importance of Class 12 Chemistry D and F Block Elements Notes
The D and F Block Elements Class 12 notes consist of the Position of Periodic table. Studying which elements are in which group position in the periodic table is vital for comprehending such elements. The D and F block components have certain features that qualify them for this category. Students can use D and F Block Elements notes.
The periodic table's d block position comprises elements ranging from 3 to 12. The d- orbitals in this group are gradually filled. The transition elements are the elements found in the contemporary periodic table between the S and P block elements. Students are introduced to different physical properties; this includes Metallic Character. This involves many physical and chemical features that are shared. As a result, all transition elements are metals. As a result, they conduct electricity and heat well, have a metallic lustre, and are complex, robust, and ductile. The D and F Block Elements NEET notes PDF covers all aspects of the chapter.
Notes carry all the tables and formulas presented in an easy way to learn and understand. In these D and F block Elements Class 12 notes, students can understand the transition series of D Block Elements. This includes the First Transition Series, Second Transition, Third Transition, and Fourth Transition.
Benefits of Class 12 Chemistry D and F Block Elements Notes
Students can use D and F Block Elements Class 12 notes for NEET preparation, created by subject matter experts of Vedantu. Students using D and F Block Elements notes can benefit in many ways, including:
They can use it for the last-minute revision and enjoy the process of learning through simple words.
By practising the chapter, students can comprehend differential graphs and tables, which are essential to this chapter.
Vedantu specialists have taken extra care in crafting class 12 Chemistry D and F Block Element notes and differentiating different properties to ensure that students are well prepared for their exams.
If there are any unclear portions of the chapter, students can refer to the solutions supplied along with the important questions to remove all of their worries.
Download Notes of D and F Block Elements Class 12 PDF
If you are looking forward to preparing for the NEET exam and learning the Chemistry chapter, download notes of D and F Block Elements class 12 PDF, which is free. Use d and f block elements neet revision notes pdf that is pretty easy to download and use for preparation. The ultimate aim of using Class 12 Chemistry D and F Block Elements notes is to score well in the NEET exam.
NEET Chemistry Revision Notes - Chapter Pages
NEET Chemistry Chapter-wise Revision Notes | |
Classification of Elements and Periodicity in Properties Notes | |
D and F Block Elements Notes | |
Other Important Links Related to NEET D and F Block Elements
Other Important Links for NEET D and F Block Elements |
FAQs on Revision Notes on D and F Block Elements Class 12 NEET 2024
1. What exactly are D and F-Block Elements 12?
The D block and F Block Elements in groups 3 to 11 are also known as transition elements and inner transition elements, respectively.
2. What are d-block and f-block elements?
Transition metals are d-block elements with valence electrons in d orbitals. Inner transition metals are the f-block elements, which are found in the two rows at the bottom of the periodic table and have valence electrons in the f-orbitals.
3. Is the D and F-Block Easy?
It is divided into two series: lanthanides and actinides. It is challenging to study the characteristics of lanthanides and actinides combined. It is difficult to draw a clear line between the two without causing misunderstanding.
4. What does F-Block Element mean?
Elements with an electron-filled f orbital are referred to as f block elements. These elements carry electrons (1 to 14) in the f orbital, (0 to 1) in the penultimate energy level's d orbital, and (0 to 1) in the outermost orbital.
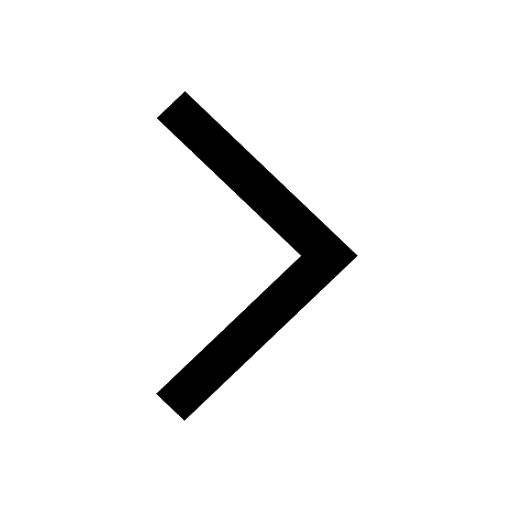
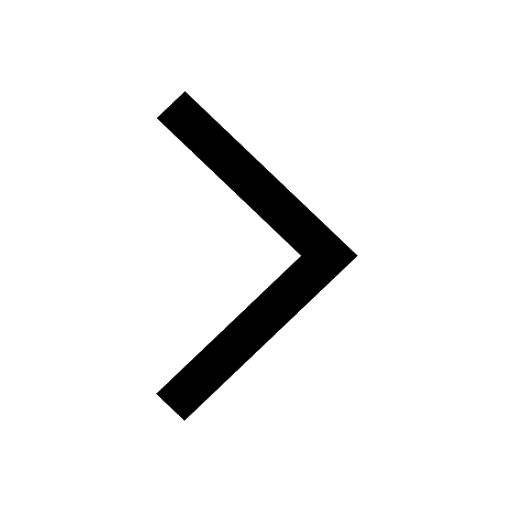
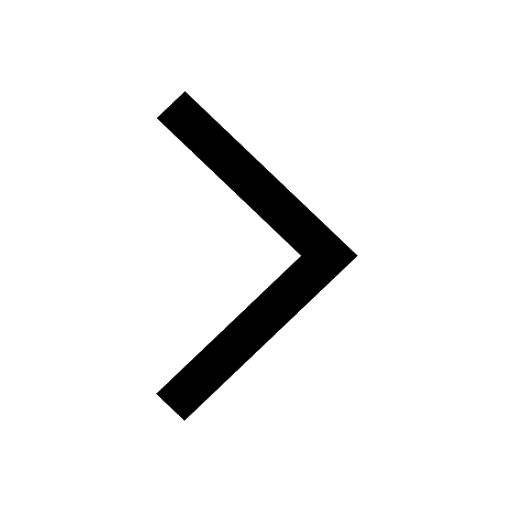
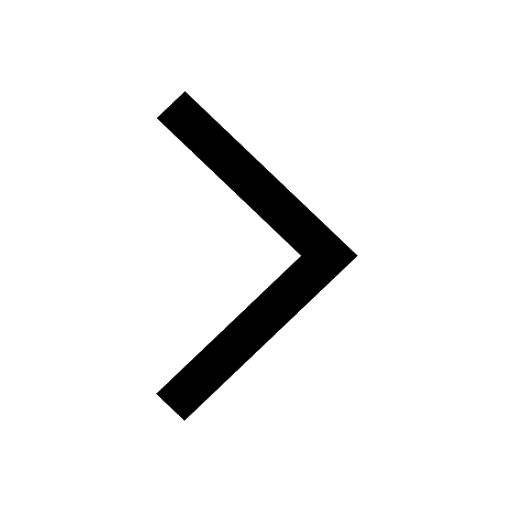
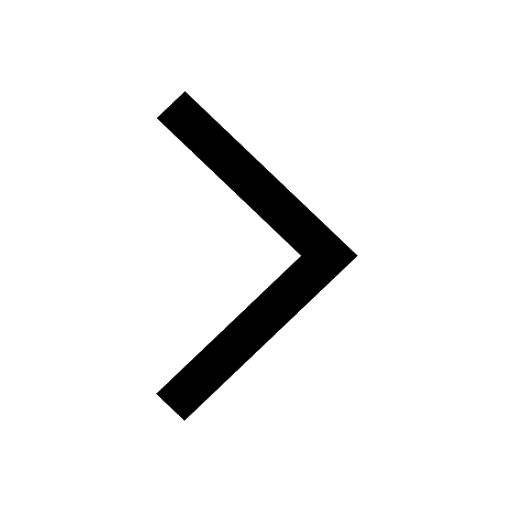
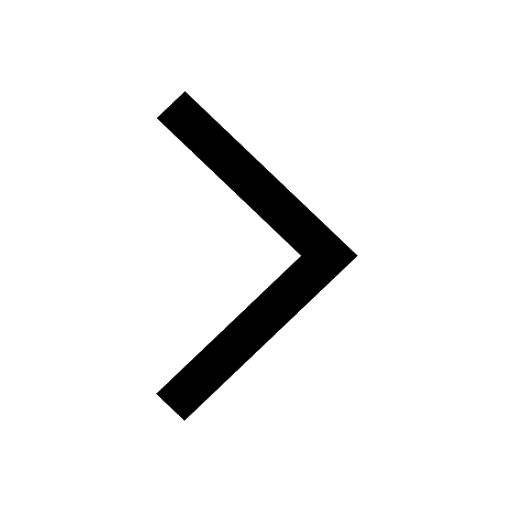