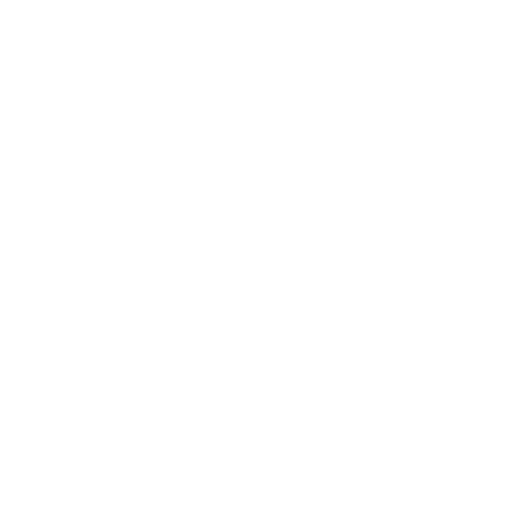

Concepts of Chemical Thermodynamics for JEE Main Chemistry
Thermodynamics is a field of chemistry and physics that studies heat, work, and temperature, as well as their relationships with energy, radiation, and physical properties of matter. The behaviour of these values is governed by the four laws of thermodynamics. It offers a quantitative description using quantifiable macroscopic physical quantities, but statistical mechanics may explain it in terms of tiny elements.
Thus, the study of heat and energy in relation to various physical transformations and chemical processes is known as thermodynamics. It is primarily concerned with a shift in energy, particularly in the context of a system's energy exchange with its surroundings. A reaction can absorb or release energy, and phase changes, such as boiling and melting, can do the same. Entropy is used to determine whether a reaction is favourable or unfavourable, spontaneous or nonspontaneous in nature. It's also used to figure out how much of each product and reactant there is in a full reaction.
Thermodynamics is an important topic in the JEE exam. Open systems, closed systems, isolated systems, rules of thermodynamics, and so on are all significant topics. This article provides an example of the types of questions that might be asked about this subject.
JEE Main Chemistry Chapters 2025
Important Topics of Chemical Thermodynamics Chapter
Thermodynamic Processes
First Law of Thermodynamics
Second Law of Thermodynamics
Third Law of Thermodynamics
Enthalpy and Enthalpy Change
Specific and Molar Heat Capacity
Carnot Cycle
Free Energy
Exothermic Reaction
Endothermic Reaction
Heat of Reaction
Important Definitions of Chemical Thermodynamics
State of a System and State Variables
State variables, state functions, and thermodynamic parameters are macroscopic attributes that specify the state of a system.
The starting and ending states of the system are the sole factors that affect the state attributes.
It is unaffected by the method by which the change was implemented.
Thermodynamic Equilibrium
When a system shows no further tendency to change its attribute with time, it is considered to have reached thermodynamic equilibrium.
The thermodynamic equilibrium criterion states that in a system, the following three equilibrium forms must occur at the same time.
Chemical Equilibrium
A system in which the composition of the system remains constant and definite.
Mechanical Equilibrium
There is no chemical interaction between the various parts of the system or between the system and the environment.
Maintain steady pressure to achieve it.
Thermal Equilibrium
It is possible when the temperature remains constant, i.e. there is no heat movement between the system and the environment.
Thermodynamic Processes
A process is defined as the transition of a thermodynamic system from one state to another.
There are many sorts of processes which are described below.
Isothermal Process
The operation is carried out at a steady temperature in this technique. As dT = 0, ΔE = 0 follows.
Adiabatic Process
There is no heat exchange between the system and its surroundings in this procedure.
The system is thermally isolated, with dQ = 0 and insulated borders.
Isobaric Process
The pressure remains constant throughout the shift in this process, i.e., dP = 0.
For example, when water boils, it turns into steam, and when water freezes, it turns into ice.
Isochoric Process
Volume remains constant throughout the shift in this process, i.e., dV = 0.
For instance, when a gasoline-air combination is burned in an automobile engine, the temperature and pressure of the gas inside the engine rises. In the meantime, the gas volume remains unchanged.
Cyclic Process
A cyclic process occurs when a system passes through a series of processes before returning to its original condition.
dE = 0 and dH = 0 for a cyclic process.
Reversible Process
A reversible process is one that occurs infinitesimally slowly, that is, when the opposing force is infinitesimally smaller than the driving force and when an infinitesimal increase in the opposing force can reverse the process.
Extending springs, for example. The frictionless motion of solids is electrolysis (with no resistance in the electrolyte).
Irreversible Process
An irreversible process happens when a process moves from its initial state to its final state in a single step over a defined period of time and cannot be reversed.
In an irreversible process, the amount of entropy increases.
Nature is full of irreversible processes.
In nature, all-natural processes are irreversible.
Electric current flow via a conductor with resistance is an example of an irreversible process.
Internal Energy
Every system containing some amount of matter has a specific amount of energy associated with it, hence it is the energy contained inside the system.
This energy is a state function that is the sum of all forms of energies (chemical, mechanical, electrical, etc.).
It is denoted by the symbol or letter E.
First Law of Thermodynamics
This law is also known as the law of energy conservation.
It states that “Energy can neither be created nor destroyed although it can be converted from one form into another.”
Change in internal energy = Heat added to the system +Work done on the system.
E2 - E1 = ΔE = q + wHelmholtz and Robert Mayer proposed it.
When a system performs work (w) on the environment, its internal energy is depleted.
Change in internal energy = Heat added to the system – work done by the system in this example.
ΔE = q + (- w) = q - w
Application of First Law of Thermodynamics
The first law of thermodynamics is the principle that energy can neither be created nor destroyed, only transformed from one form to another. This means that the total energy of a closed system remains constant. Here are some of the application of First Law of Thermodynamics in action:
A Gas Expanding in a Piston: The gas does work on the piston, and the internal energy of the gas decreases. However, the total energy of the system (gas plus piston) remains constant.
A Refrigerator: The refrigerator removes heat from the inside compartment and transfers it to the outside compartment. The work done by the refrigerator increases the internal energy of the outside compartment, but the total energy of the system (refrigerator plus inside compartment plus outside compartment) remains constant.
A Person Exercising: The person's muscles do work, and the chemical energy stored in their food is converted to mechanical energy. However, the total energy of the person's body remains constant, as the chemical energy is stored in a different form (e.g., heat, sweat) after exercise.
Limitations of First Law of Thermodynamics
The first law of thermodynamics states that the total energy of a system is always constant. This means that energy can neither be created nor destroyed, only transferred from one form to another. While this law is fundamental to understanding the behavior of physical systems, it has some limitations.
The first law does not tell us the direction of heat flow. It only tells us that the total amount of energy is conserved. For example, we cannot use the first law to determine whether heat will flow from a hot object to a cold object or vice versa.
The first law does not tell us whether a process is spontaneous or not. A spontaneous process is one that occurs naturally without any external input of energy. The first law cannot tell us whether a process is spontaneous or not, because it does not consider the entropy of the system. Entropy is a measure of the disorder of a system. In general, processes that increase entropy are spontaneous.
The first law does not specify the feasibility of a reaction. The first law cannot tell us whether a chemical reaction is feasible or not. This is because the first law does not consider the activation energy of the reaction. Activation energy is the minimum amount of energy that must be added to the reactants to start the reaction. If the activation energy is too high, the reaction will not occur.
The limitations of the first law of thermodynamics can be overcome by using the second law of thermodynamics. The second law of thermodynamics introduces the concept of entropy, which allows us to determine the direction of heat flow and the feasibility of reactions.
Enthalpy and Enthalpy Change
Enthalpy is the heat content of a system at constant pressure.
The letter 'H' stands for it.
From the first law of thermodynamics,
q = E + W ; q = E + PΔV
Heat change in constant pressure can be given, q = E + PΔV.
At constant pressure, heat can be replaced at enthalpy. ΔH = E + PΔV.
H Is the heat of reaction (in the chemical process) or the heat change at constant pressure.
Specific and Molar Heat Capacity
A substance's specific heat capacity is the amount of heat required to raise the temperature of one gramme of the substance by one degree Celsius.
The quantity of heat required to increase the temperature by 1℃ of 1 g of a substance is known as its specific heat capacity.
q = mcΔT; (cp = constant pressure/cv = constant volume)The quantity of heat required to increase the temperature by 1℃ of 1 mole of a substance is known as its molar heat capacity.
When heated under constant pressure settings, gasses on heat have a predisposition to expand, requiring an additional energy supply to raise their temperature 1°C when necessary under constant volume conditions.
Therefore, Cp > Cv. Cp = Cv + Work done in expansion; Work done in expansion is PΔV(=R); Cv = molar heat capacities at constant volume, Cp = molar heat capacities at constant pressure.
Relations between Cp and Cv.
Second Law of Thermodynamics
The second law of thermodynamics eliminates all of the first law's constraints.
This law indicates that the overall energy change for spontaneous processes is positive.
Second Law of Thermodynamics Examples
The second law of thermodynamics governs the direction and efficiency of natural processes. It states that the entropy of an isolated system always increases over time. Here are some examples of the second law in action:
Heat Transfer: Heat always flows from a hotter object to a colder object, never the other way around. This is because the entropy of the system increases as heat spreads out from the hotter object.
Friction: When two objects rub against each other, kinetic energy is converted into heat, increasing the entropy of the system. Friction always opposes motion, slowing down objects over time.
Irreversible Processes: Many natural processes, such as mixing substances or dissolving sugar in water, are irreversible. This means that they cannot be undone without adding energy to the system, increasing its entropy.
Car Engines: Car engines convert chemical energy from fuel into mechanical energy, but some energy is always lost as heat. This is because the second law dictates that no process is 100% efficient.
Limitations of Second Law of Thermodynamics
The second law of thermodynamics imposes limitations on the efficiency of energy conversion processes and the direction of spontaneous processes. Here are some key limitations of Second Law of Thermodynamics:
Irreversibility of Heat Transfer: It is impossible to completely convert heat into work without generating some waste heat.
Directionality of Spontaneous Processes: Spontaneous processes only occur in the direction of decreasing entropy, indicating that order tends to go towards disorder.
Limit to Efficiency of Heat Engines: No heat engine can achieve 100% efficiency, meaning there is always some energy loss as heat.
Absolute Zero Cannot be Reached: The absolute zero of temperature (0 Kelvin) cannot be physically attained, as it would require the complete absence of molecular motion.
Entropy Always Increases: The total entropy of an isolated system always increases over time, indicating a tendency towards disorder and randomness.
The Carnot Cycle
A cyclic process is defined as one in which the net work done equals the heat absorbed.
During a Carnot cycle, this condition is met.
Thus, the bigger the temperature differential between the high and low-temperature reservoirs, the more heat is generated, which is converted into work by the heat engine.
Thermodynamic efficiency = w/q2=(T2-T1)/T1.
Entropy
The unpredictability or disorder of the system's molecules is measured by entropy.
It's a measure of the condition of the thermodynamic system.
Sfinal - Sinitial = ΔS = qrev/T.
If heat is absorbed, then S -ve and if heat is evolved, then, ΔS = -ve.
Free Energy
Gibbs free energy (G) is a measure of maximum work done from a reversible process at constant pressure and temperature, also known as useful work done.
We can find it by subtracting the system's enthalpy from the product of absolute temperature and entropy; i.e., ΔG = ΔH - TΔS.
Third Law of Thermodynamics
The Third law of Thermodynamics states that “The entropy of all perfectly crystalline solids approaches zero at the temperature approaches absolute zero.”
Because entropy is a measure of disorder, it can be deduced that at absolute zero, a perfectly crystalline material has a perfect order of its constituent particles.
Third Law of Thermodynamics Examples
Here are some examples of the 3rd law of thermodynamics for JEE Main:
The entropy of a perfect crystal approaches zero as the temperature approaches absolute zero (0 K). This is because the molecules in a perfect crystal are perfectly ordered, and there is no randomness or disorder.
The heat capacity of a solid approaches zero as the temperature approaches absolute zero (0 K). This is because the molecules in a solid have very little kinetic energy at low temperatures, and they are not able to move around very much.
The entropy change for a chemical reaction approaches zero as the temperature approaches absolute zero (0 K). This is because the products of a reaction are more ordered than the reactants, and the entropy change is therefore negative.
The equilibrium constant for a chemical reaction approaches a constant value as the temperature approaches absolute zero (0 K). This is because the enthalpy change for a reaction is constant at all temperatures, and the entropy change approaches zero as the temperature approaches absolute zero.
Limitations of Third Law of Thermodynamics
The 3rd law of thermodynamics states that the entropy of a perfect crystal approaches zero as the temperature approaches absolute zero. However, there are some limitations of third law of Thermodynamics.
One limitation is that it is not possible to reach absolute zero. The lowest temperature that has been achieved in a laboratory is about 1 picoKelvin, which is still far from absolute zero.
Another limitation is that the 3rd law of thermodynamics does not apply to all substances. Some substances, such as glasses and amorphous solids, do not have a perfect crystal structure and therefore do not have a zero entropy at absolute zero.
Finally, the third law of thermodynamics does not provide any information about the entropy of a substance at a temperature other than absolute zero.
Exothermic and Endothermic Reactions
Exothermic reactions are those that occur as a result of heat energy evolution.
ΔH = Hp - Hr; Hp < Hr; ΔH = -ve.
Examples of Exothermic Reactions
Endothermic reactions are those that take place when heat energy is absorbed.
ΔH = Hp - Hr; Hp < Hr; ΔH = +ve.Examples of Endothermic Reactions
Heat of Reaction or Enthalpy of Reaction
The difference between the products and reactants enthalpies obtained when the reactants reacted completely in a chemical process is known as the heat of reaction or reaction enthalpy.
Enthalpy of reaction (heat of reaction) = ΔH = ΣHp - ΣHr.
Application of Thermodynamics
Every vehicle you drive or see on the road, sky, and even in the sea, all their engines are working based on the second law of thermodynamics. It doesn't matter if they are using petrol or diesel engine the laws will work in the same way.
(Image to be added soon)
(refrigerators usings laws of thermodynamics to keep food fresh during summertime)
Secondly, the refrigerators we have in our homes, deep freezers, and other air conditioning systems also work on the second law of thermodynamics.
Lastly, all the compressors and the blower fans you see around you are using various thermodynamic laws and cycles to keep it working continuously.
JEE Main Chemical Thermodynamics Solved Examples From Chapter
Example 1: Explain how you'd go about calculating w and q throughout the time it takes for the piston velocity to drop to zero.
Solution:
The relationship between V1 and V2 is discovered by equating the piston's effort on the gas.
∴ nRT.ln(V2/V1) = p2(V2-V1); p2(V2-V1) = external work performed on the piston;
pext = 2p1 = 2nRT/V1.
V2 = (0.2032)V1
w = (1.5936)nRT,
q = - w =( -1.5936)nRT.
Key Point to Remember: The relationship between the number of moles of gas, pressure and volume: nRT.ln(V2/V1) = p2(V2-V1) i.e. the external work done. Also, the values of R will be the given values for the constant and the values of n and T will be the ones provided in any question of this type.
Example 2: Liquid nitrogen, with a boiling point of -195.79°C, is utilized as a coolant and biological tissue preservation. Is nitrogen's entropy higher or lower at -200°C than it is at -190°C? Give an explanation for your response. At -210.00°C, liquid nitrogen freezes to a white solid with a fusion enthalpy of 0.71 kJ/mol. What is fusion entropy? Is it possible to freeze living tissue in liquid nitrogen in a reversible or irreversible manner?
Solution: As the temperature decreases the entropy increases, as they are in inverse correlation to each other. Hence, when the temperature of liquid nitrogen decreases to -200°C, its entropy is higher than at -190°C.
Key Point to Remember: Relation between entropy and temperature: ∆S=∆H/T.
Solved Questions From The Previous Year Question Papers
Question 1: If an endothermic reaction is nonspontaneous at the freezing point of water and becomes feasible at its boiling point, then
(a) ∆H is –ve, ∆S is +ve
(b) ∆H and ∆S both are +ve
(c) ∆H and ∆S both are –ve
(d) ∆H is +ve, ∆S is –ve
Solution: For an endothermic reaction, ΔH = positive value. ΔG = ΔH – TΔS
For a non-spontaneous reaction, ΔG should be positive.
At low temperatures, ΔG is positive if ΔH is positive.
If S is positive, ΔG is negative at high temperatures.
As a result, option (b) is the correct answer.
Trick: Low Temperatures: ΔG is positive if ΔH is positive, High Temperatures: ΔG is negative if S is positive.c
Question 2: The standard enthalpy of formation of NH3 is - 46 kJ mol–1. If the enthalpy of formation of H2 from its atoms is - 436 kJ mol–1 and that of N2 is -712 kJ mol–1, the average bond enthalpy of the N-H bond in NH3 is
(1) - 964 kJ mol–1
(2) + 352 kJ mol–1
(3) + 1056 kJ mol–1
(4) - 1102 kJ mol–1
Solution:
The equation of formation of ammonia using nitrogen and hydrogen is given below:
½ N2 + ½H2 → NH3
The standard enthalpy of formation of ammonia, given by ∆Hf of NH3, can thus be determined as follows:
∆Hf of NH3 = [(1/2)B.E of N2 + (3/2) B.E of H2 – (3) B.E of N-H]
Here, B.E stands for Bond energy and ∆Hf stands for standard enthalpy of formation.
In the above equation the enthalpy of the formation of ammonia is obtained as a summation of the bond energies of the constituent elements and the subtraction of the bond energies of nitrogen and hydrogen bonds.
- 46 = [(1/2)(712) + (3/2) 436 – (3) B.E of N-H]
- 46 = 356 + 654 – 3 B.E of N-H
3 B.E of N-H = 1056
∴ B.E of N-H = 1056/3 = 352 kJ mol–1
Hence, option (2) is the answer.
Trick: Enthalpy of formation can be calculated by subtracting the sum of bond energies of the bonds that are formed in the chemical reaction from the sum of bond energies of the bonds that are broken in the chemical reaction.
Question 3: During compression of a spring, the work done is 10 kJ and 2 kJ escapes to the surroundings as heat. The change in internal energy ∆U (in kJ) is
(a) – 8
(b) 12
(c) 8
(d) – 12
Solution: The work done is given by, w = 10 kJ
The heat that escapes into the surrounding is expressed as q = – 2 kJ
The first law of thermodynamics states that ∆U = q + w
= – 2 + 10 = 8 kJ
As a result, option (c) is the correct answer.
Trick: Work done on the system has a positive magnitude and work done by the system has a negative magnitude. Similarly, the heat released by the system has a negative magnitude and heat supplied by the surroundings to the system has a positive magnitude.
Practice Questions
Question 1: A leak in a Russian spacecraft resulted in a reduction in internal pressure from 1 atm to 0.85 atm. Is this a reversible expansion scenario? Has any work been completed?
Answer: It is irreversible.
Question 2: From the following thermochemical data, calculate the enthalpy of production of OH– ions:
H2O → H+(aq) + OH-(aq); ΔH+298K = +57.32 kJ
H2 + 1/2O2 → H2O(l); ΔH+298K = -285.83 kJ
Answer: - 228.51 kJ.
JEE Main Chemistry Chemical Thermodynamics Study Materials
Here, you'll find a comprehensive collection of study resources for Chemical Thermodynamics designed to help you excel in your JEE Main preparation. These materials cover various topics, providing you with a range of valuable content to support your studies. Simply click on the links below to access the study materials of Chemical Thermodynamics and enhance your preparation for this challenging exam.
JEE Main Chemistry Study and Practice Materials
Explore an array of resources in the JEE Main Chemistry Study and Practice Materials section. Our practice materials offer a wide variety of questions, comprehensive solutions, and a realistic test experience to elevate your preparation for the JEE Main exam. These tools are indispensable for self-assessment, boosting confidence, and refining problem-solving abilities, guaranteeing your readiness for the test. Explore the links below to enrich your Chemistry preparation.
Conclusion
Thus, the study of how one body interacts with another in terms of heat and work is called thermodynamics. A quantity of substance or a location in space under inquiry is defined as a system in this case. The environment is anything that exists outside of the system.
A variety of concepts are involved around these conditions and calculations using these concepts help understand the changes in the environment, surrounding or experiment and further understand a phenomenon in detail. Hence, this is important not only for competitive exams like JEE or NEET but also for a better understanding of the thermodynamics of nature.
Chemical Thermodynamics Chapter - Chemistry JEE Main

FAQs on Chemical Thermodynamics Chapter - Chemistry JEE Main
1. What is the meaning of thermodynamics in chemistry?
The study of the relationships between heat, work, temperature, and energy is known as thermodynamics. The rules of thermodynamics define how energy evolves in a system and whether it can do beneficial work on its surroundings.
2. What is the difference between chemical thermodynamics and physical thermodynamics?
Chemical thermodynamics is concerned with the relationship between heat and work in chemical reactions, as well as the change of state. Physical thermodynamics, on the other hand, is concerned with physical quantities like temperature, pressure, volume, entropy, and enthalpy.
3. What is thermodynamics and chemical energetics?
Aspects of Energy (Thermodynamics) The discipline of chemistry that examines the energetics of chemical reactions is known as thermodynamics (from the Greek roots for heat and force, power, or energy). There are a variety of energy-related quantities to consider.
4. What is First Law of Thermodynamics for class 11?
The First Law of Thermodynamics, also known as the Law of Conservation of Energy, states that energy cannot be created or destroyed, only transformed from one form to another. In a closed system, the total energy remains constant. This means that heat energy can be converted into mechanical energy, and vice versa.
The First Law is expressed mathematically as,
\Delta U = Q + W
Where,
\Delta U is the change in internal energy
Q is the heat added to the system
W is the work done by the system
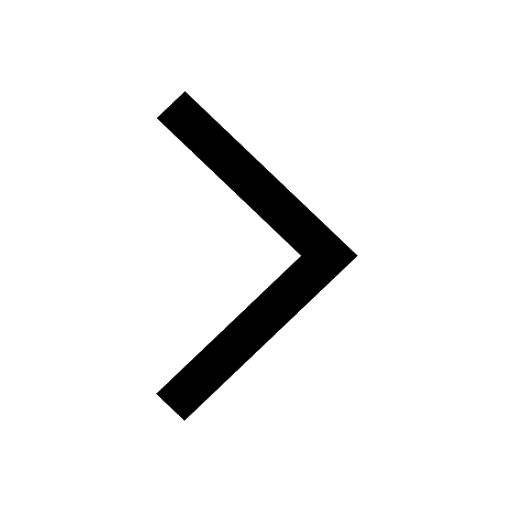
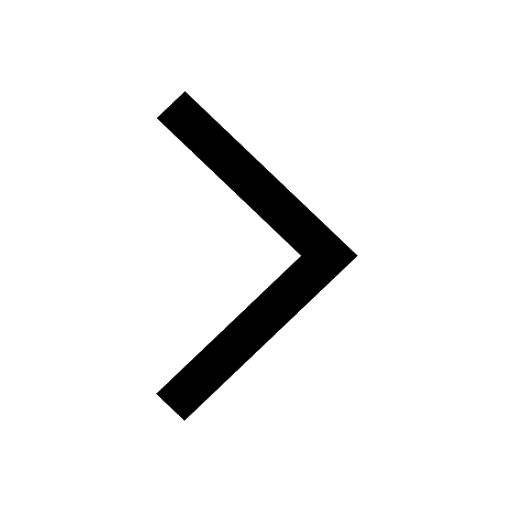
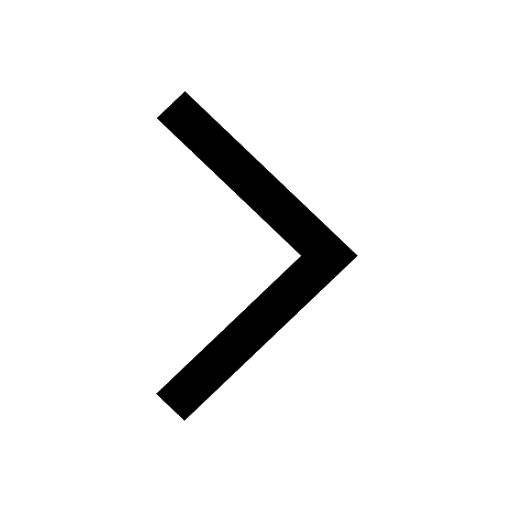
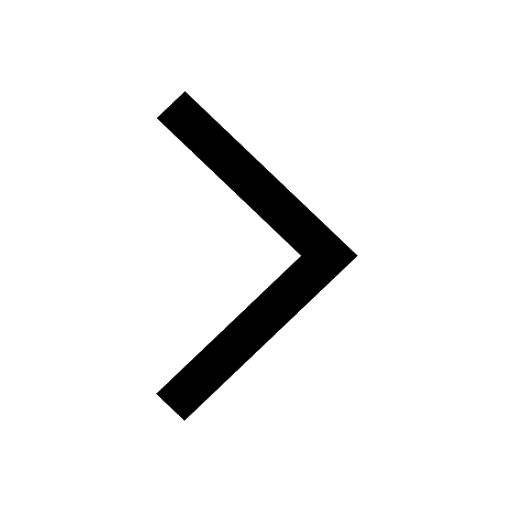
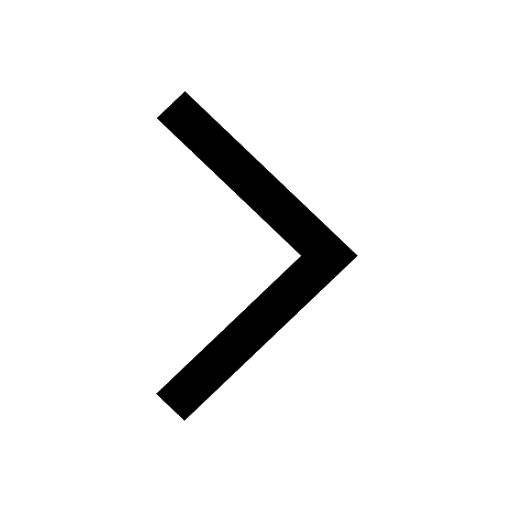
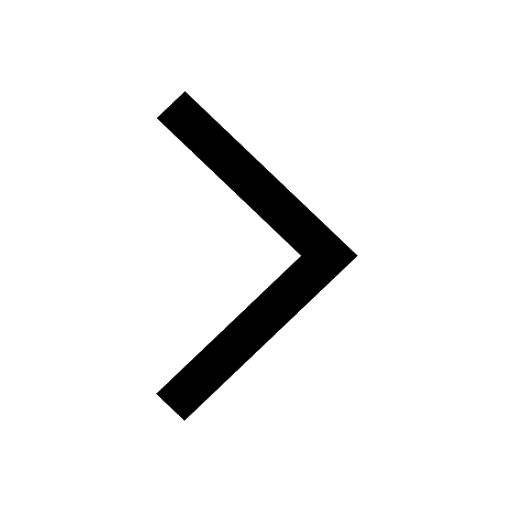
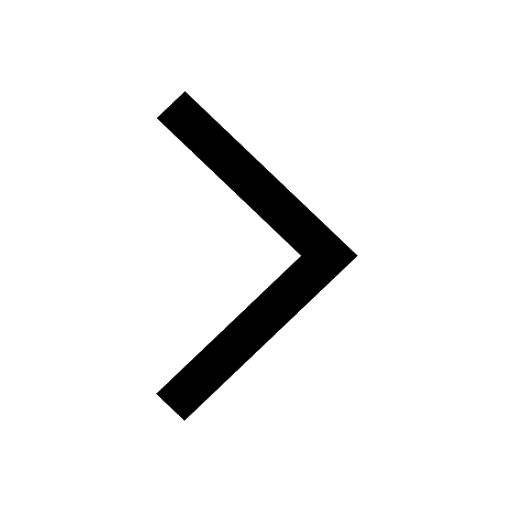
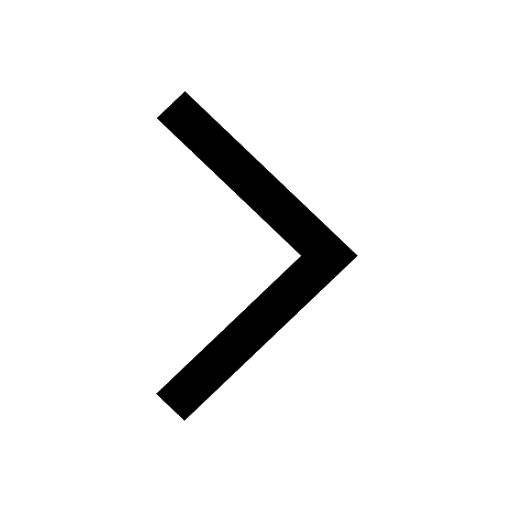
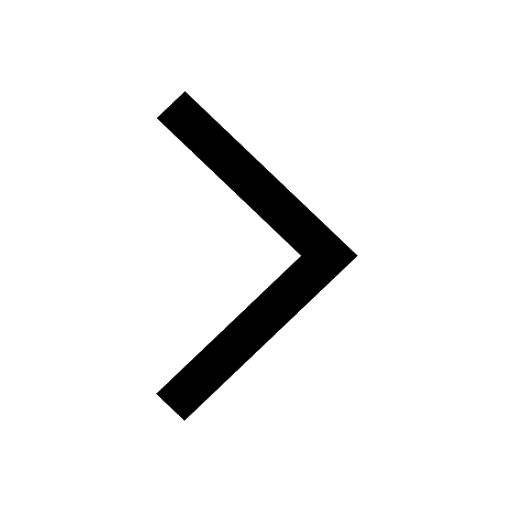
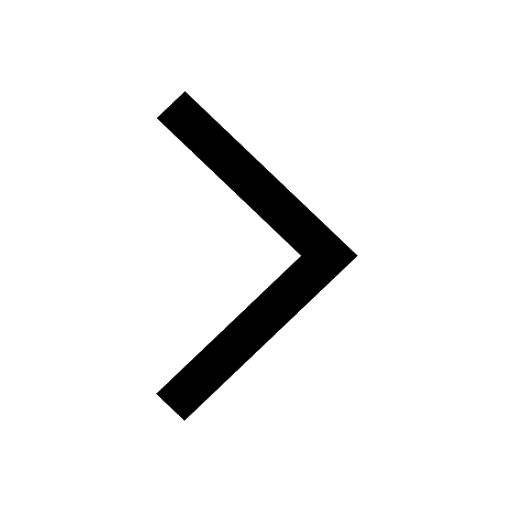
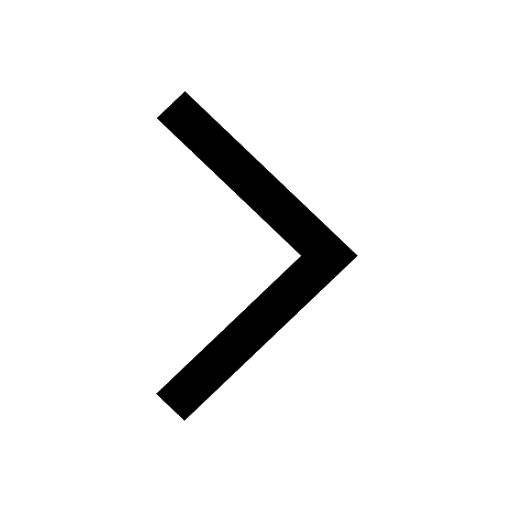
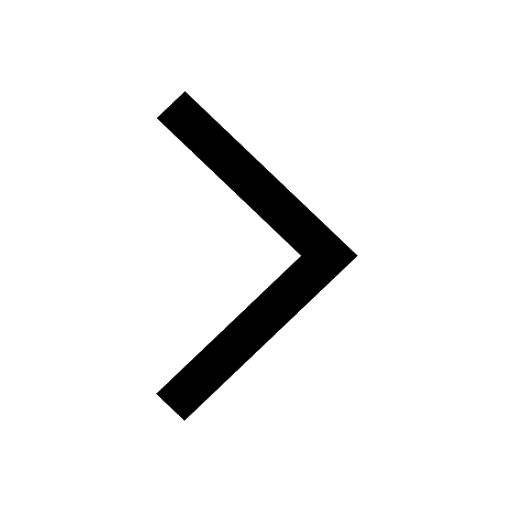