Answer
385.8k+ views
Hint We can obtain the equation of the resulting current through the wire by adding the direct current to the alternating current. The effective value of current is equal to the root mean square value of the current. So we need to take the square, calculate its mean by integrating it over a time period, and take the square root to obtain the RMS value which will be the required effective current.
Complete step-by-step solution:
Since the direct current is superimposed over the alternating current, the resulting current will be equal to the sum of the direct and the alternating current. So we have
${I_R} = {I_{dc}} + {I_{ac}}$
According to the question, ${I_{dc}} = 5{\text{A}}$ and ${I_{ac}} = 10\sin \omega t$. Substituting these in the above equation, we get the resultant current as
${I_R} = 5 + 10\sin \omega t$
Now, we know that the effective value of the current is nothing but the RMS, or the root mean square value of the current. By the definition of RMS, we have to take the square, then take the mean, and finally take the square root of the current. So on squaring both sides of the above equation, we get
${I_R}^2 = {\left( {5 + 10\sin \omega t} \right)^2}$
We know that ${\left( {a + b} \right)^2} = {a^2} + 2ab + {b^2}$. So we write the above equation as
${I_R}^2 = {5^2} + 2\left( 5 \right)\left( {10\sin \omega t} \right) + {\left( {10\sin \omega t} \right)^2}$
$ \Rightarrow {I_R}^2 = 25 + 100\sin \omega t + 100{\sin ^2}\omega t$ (1)
Now, we know that the mean of a function is given by
$M = \dfrac{1}{T}\int_0^T {f\left( t \right)dt} $
The period of the function ${I_R}^2$ is clearly equal to $\dfrac{{2\pi }}{\omega }$. So we integrate it from $0$ to $\dfrac{{2\pi }}{\omega }$ to get its mean as
\[M = \dfrac{1}{{2\pi /\omega }}\int_0^{\dfrac{{2\pi }}{\omega }} {{I_R}^2dt} \]
\[ \Rightarrow M = \dfrac{\omega }{{2\pi }}\int_0^{\dfrac{{2\pi }}{\omega }} {{I_R}^2dt} \]
Putting (1) above, we get
\[ \Rightarrow M = \dfrac{\omega }{{2\pi }}\int_0^{\dfrac{{2\pi }}{\omega }} {\left( {25 + 100\sin \omega t + 100{{\sin }^2}\omega t} \right)dt} \]
\[ \Rightarrow M = \dfrac{\omega }{{2\pi }}\left( {\int_0^{\dfrac{{2\pi }}{\omega }} {25dt} + \int_0^{\dfrac{{2\pi }}{\omega }} {100\sin \omega tdt} + \int_0^{\dfrac{{2\pi }}{\omega }} {100{{\sin }^2}\omega tdt} } \right)\]
We know that the average value of the sinusoidal functions is equal to zero. So we can put \[\int_0^{\dfrac{{2\pi }}{\omega }} {100\sin \omega tdt} = 0\] in the above equation to get
\[M = \dfrac{\omega }{{2\pi }}\left( {\int_0^{\dfrac{{2\pi }}{\omega }} {25dt} + \int_0^{\dfrac{{2\pi }}{\omega }} {100{{\sin }^2}\omega tdt} } \right)\]
We know that ${\sin ^2}\theta = \dfrac{{1 - \cos 2\theta }}{2}$. So we write the above equation as\[M = \dfrac{\omega }{{2\pi }}\left( {\int_0^{\dfrac{{2\pi }}{\omega }} {25dt} + \int_0^{\dfrac{{2\pi }}{\omega }} {100\left( {\dfrac{{1 - \cos 2\omega t}}{2}} \right)dt} } \right)\]
\[ \Rightarrow M = \dfrac{\omega }{{2\pi }}\left( {25\int_0^{\dfrac{{2\pi }}{\omega }} {dt} + 50\int_0^{\dfrac{{2\pi }}{\omega }} {\left( {1 - \cos 2\omega t} \right)dt} } \right)\]
\[ \Rightarrow M = \dfrac{\omega }{{2\pi }}\left( {25\int_0^{\dfrac{{2\pi }}{\omega }} {dt} + 50\int_0^{\dfrac{{2\pi }}{\omega }} {dt} - \int_0^{\dfrac{{2\pi }}{\omega }} {\cos 2\omega tdt} } \right)\]
We know that $\int {dt} = t$. So we get
\[ \Rightarrow M = \dfrac{\omega }{{2\pi }}\left( {25\left[ t \right]_0^{\dfrac{{2\pi }}{\omega }} + 5025\left[ t \right]_0^{\dfrac{{2\pi }}{\omega }} - 50\int_0^{\dfrac{{2\pi }}{\omega }} {\cos 2\omega tdt} } \right)\]
\[ \Rightarrow M = \dfrac{\omega }{{2\pi }}\left( {25\left( {\dfrac{{2\pi }}{\omega }} \right) + 50\left( {\dfrac{{2\pi }}{\omega }} \right) - \int_0^{\dfrac{{2\pi }}{\omega }} {\cos 2\omega tdt} } \right)\]
Since $\cos 2\omega t$ is also sinusoidal, its average over a cycle will also be zero, that is, \[\int_0^{\dfrac{{2\pi }}{\omega }} {\cos 2\omega tdt} = 0\].
\[ \Rightarrow M = \dfrac{\omega }{{2\pi }}\left( {25\left( {\dfrac{{2\pi }}{\omega }} \right) + 50\left( {\dfrac{{2\pi }}{\omega }} \right)} \right)\]
\[ \Rightarrow M = \left( {25 + 50} \right) = 75{{\text{A}}^2}\]
Now, we take the square root of this mean value to get the final RMS value of the resulting current as
$RMS = \sqrt M $
\[ \Rightarrow RMS = \sqrt {75} {\text{A}} = 5\sqrt 3 {\text{A}}\]
Thus the effective value of the resulting current is equal to \[5\sqrt 3 {\text{A}}\].
Hence, the correct answer is option B.
Note: We can integrate the square of the current between any time interval which makes a complete time period. But it will be convenient to set the lower limit of the integral equal to zero. So we chose the period from $0$ to $2\pi $.
Complete step-by-step solution:
Since the direct current is superimposed over the alternating current, the resulting current will be equal to the sum of the direct and the alternating current. So we have
${I_R} = {I_{dc}} + {I_{ac}}$
According to the question, ${I_{dc}} = 5{\text{A}}$ and ${I_{ac}} = 10\sin \omega t$. Substituting these in the above equation, we get the resultant current as
${I_R} = 5 + 10\sin \omega t$
Now, we know that the effective value of the current is nothing but the RMS, or the root mean square value of the current. By the definition of RMS, we have to take the square, then take the mean, and finally take the square root of the current. So on squaring both sides of the above equation, we get
${I_R}^2 = {\left( {5 + 10\sin \omega t} \right)^2}$
We know that ${\left( {a + b} \right)^2} = {a^2} + 2ab + {b^2}$. So we write the above equation as
${I_R}^2 = {5^2} + 2\left( 5 \right)\left( {10\sin \omega t} \right) + {\left( {10\sin \omega t} \right)^2}$
$ \Rightarrow {I_R}^2 = 25 + 100\sin \omega t + 100{\sin ^2}\omega t$ (1)
Now, we know that the mean of a function is given by
$M = \dfrac{1}{T}\int_0^T {f\left( t \right)dt} $
The period of the function ${I_R}^2$ is clearly equal to $\dfrac{{2\pi }}{\omega }$. So we integrate it from $0$ to $\dfrac{{2\pi }}{\omega }$ to get its mean as
\[M = \dfrac{1}{{2\pi /\omega }}\int_0^{\dfrac{{2\pi }}{\omega }} {{I_R}^2dt} \]
\[ \Rightarrow M = \dfrac{\omega }{{2\pi }}\int_0^{\dfrac{{2\pi }}{\omega }} {{I_R}^2dt} \]
Putting (1) above, we get
\[ \Rightarrow M = \dfrac{\omega }{{2\pi }}\int_0^{\dfrac{{2\pi }}{\omega }} {\left( {25 + 100\sin \omega t + 100{{\sin }^2}\omega t} \right)dt} \]
\[ \Rightarrow M = \dfrac{\omega }{{2\pi }}\left( {\int_0^{\dfrac{{2\pi }}{\omega }} {25dt} + \int_0^{\dfrac{{2\pi }}{\omega }} {100\sin \omega tdt} + \int_0^{\dfrac{{2\pi }}{\omega }} {100{{\sin }^2}\omega tdt} } \right)\]
We know that the average value of the sinusoidal functions is equal to zero. So we can put \[\int_0^{\dfrac{{2\pi }}{\omega }} {100\sin \omega tdt} = 0\] in the above equation to get
\[M = \dfrac{\omega }{{2\pi }}\left( {\int_0^{\dfrac{{2\pi }}{\omega }} {25dt} + \int_0^{\dfrac{{2\pi }}{\omega }} {100{{\sin }^2}\omega tdt} } \right)\]
We know that ${\sin ^2}\theta = \dfrac{{1 - \cos 2\theta }}{2}$. So we write the above equation as\[M = \dfrac{\omega }{{2\pi }}\left( {\int_0^{\dfrac{{2\pi }}{\omega }} {25dt} + \int_0^{\dfrac{{2\pi }}{\omega }} {100\left( {\dfrac{{1 - \cos 2\omega t}}{2}} \right)dt} } \right)\]
\[ \Rightarrow M = \dfrac{\omega }{{2\pi }}\left( {25\int_0^{\dfrac{{2\pi }}{\omega }} {dt} + 50\int_0^{\dfrac{{2\pi }}{\omega }} {\left( {1 - \cos 2\omega t} \right)dt} } \right)\]
\[ \Rightarrow M = \dfrac{\omega }{{2\pi }}\left( {25\int_0^{\dfrac{{2\pi }}{\omega }} {dt} + 50\int_0^{\dfrac{{2\pi }}{\omega }} {dt} - \int_0^{\dfrac{{2\pi }}{\omega }} {\cos 2\omega tdt} } \right)\]
We know that $\int {dt} = t$. So we get
\[ \Rightarrow M = \dfrac{\omega }{{2\pi }}\left( {25\left[ t \right]_0^{\dfrac{{2\pi }}{\omega }} + 5025\left[ t \right]_0^{\dfrac{{2\pi }}{\omega }} - 50\int_0^{\dfrac{{2\pi }}{\omega }} {\cos 2\omega tdt} } \right)\]
\[ \Rightarrow M = \dfrac{\omega }{{2\pi }}\left( {25\left( {\dfrac{{2\pi }}{\omega }} \right) + 50\left( {\dfrac{{2\pi }}{\omega }} \right) - \int_0^{\dfrac{{2\pi }}{\omega }} {\cos 2\omega tdt} } \right)\]
Since $\cos 2\omega t$ is also sinusoidal, its average over a cycle will also be zero, that is, \[\int_0^{\dfrac{{2\pi }}{\omega }} {\cos 2\omega tdt} = 0\].
\[ \Rightarrow M = \dfrac{\omega }{{2\pi }}\left( {25\left( {\dfrac{{2\pi }}{\omega }} \right) + 50\left( {\dfrac{{2\pi }}{\omega }} \right)} \right)\]
\[ \Rightarrow M = \left( {25 + 50} \right) = 75{{\text{A}}^2}\]
Now, we take the square root of this mean value to get the final RMS value of the resulting current as
$RMS = \sqrt M $
\[ \Rightarrow RMS = \sqrt {75} {\text{A}} = 5\sqrt 3 {\text{A}}\]
Thus the effective value of the resulting current is equal to \[5\sqrt 3 {\text{A}}\].
Hence, the correct answer is option B.
Note: We can integrate the square of the current between any time interval which makes a complete time period. But it will be convenient to set the lower limit of the integral equal to zero. So we chose the period from $0$ to $2\pi $.
Recently Updated Pages
How many sigma and pi bonds are present in HCequiv class 11 chemistry CBSE
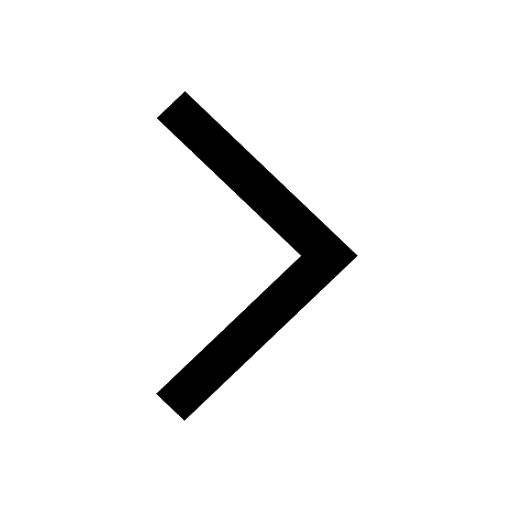
Why Are Noble Gases NonReactive class 11 chemistry CBSE
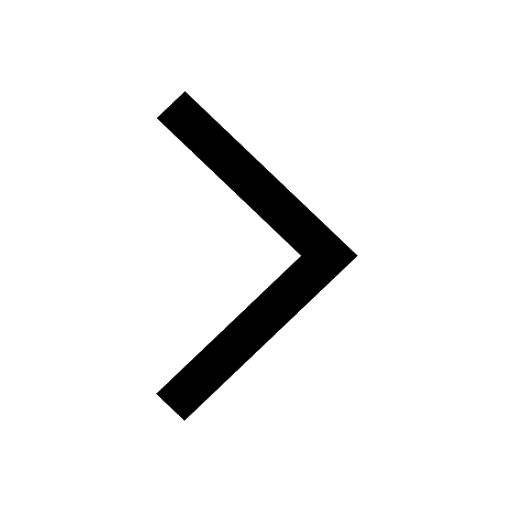
Let X and Y be the sets of all positive divisors of class 11 maths CBSE
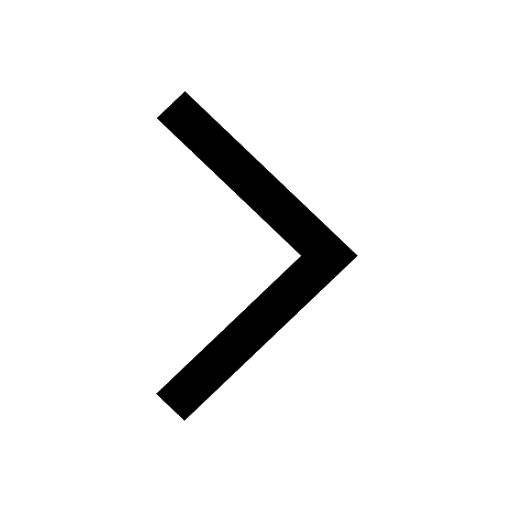
Let x and y be 2 real numbers which satisfy the equations class 11 maths CBSE
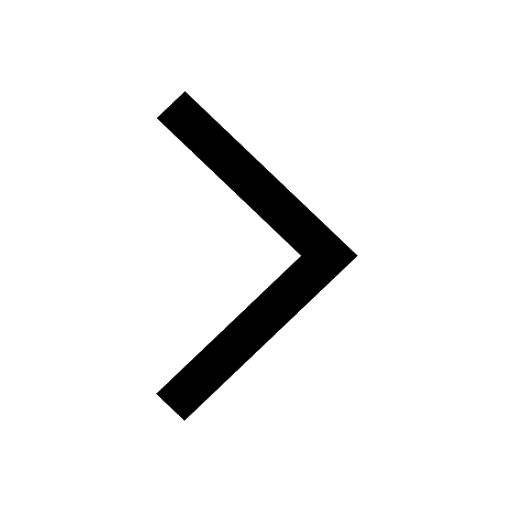
Let x 4log 2sqrt 9k 1 + 7 and y dfrac132log 2sqrt5 class 11 maths CBSE
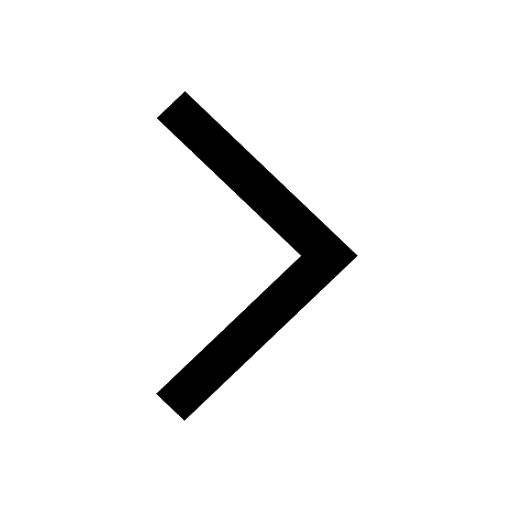
Let x22ax+b20 and x22bx+a20 be two equations Then the class 11 maths CBSE
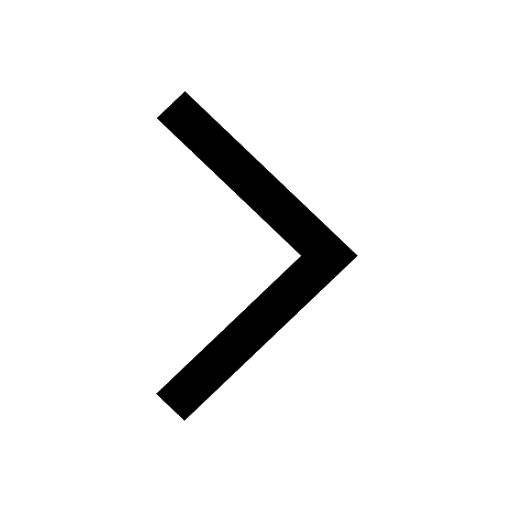
Trending doubts
Fill the blanks with the suitable prepositions 1 The class 9 english CBSE
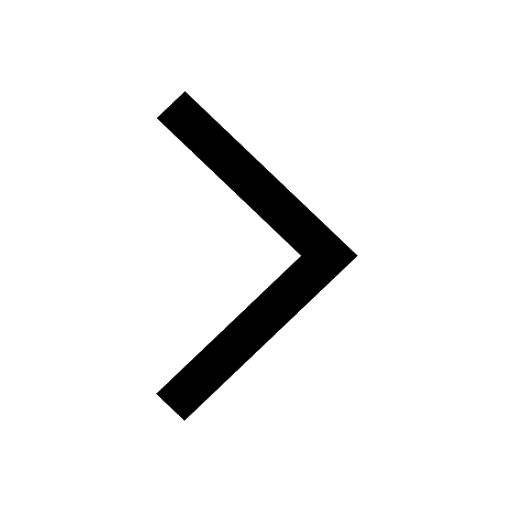
At which age domestication of animals started A Neolithic class 11 social science CBSE
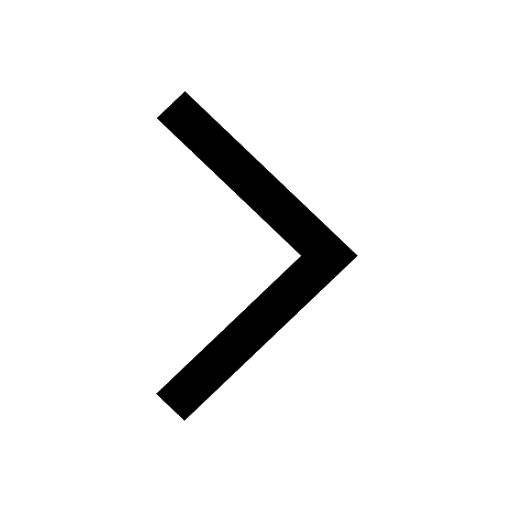
Which are the Top 10 Largest Countries of the World?
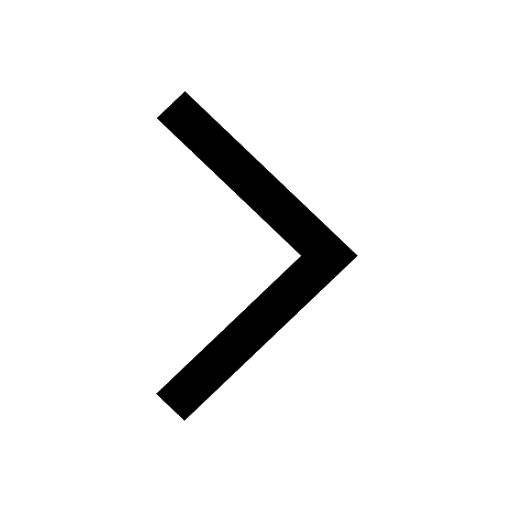
Give 10 examples for herbs , shrubs , climbers , creepers
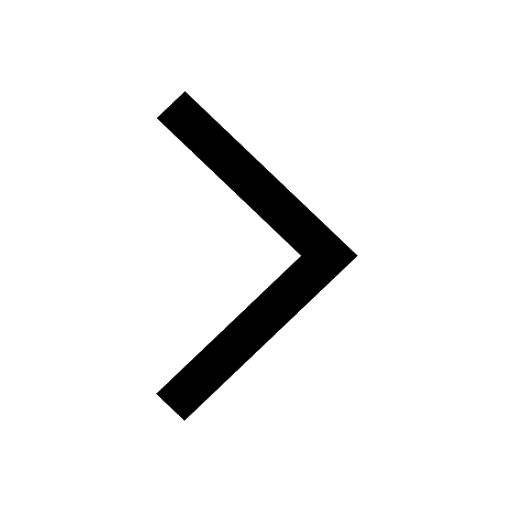
Difference between Prokaryotic cell and Eukaryotic class 11 biology CBSE
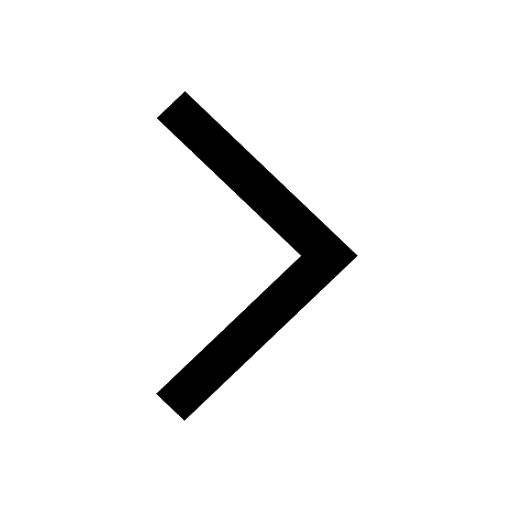
Difference Between Plant Cell and Animal Cell
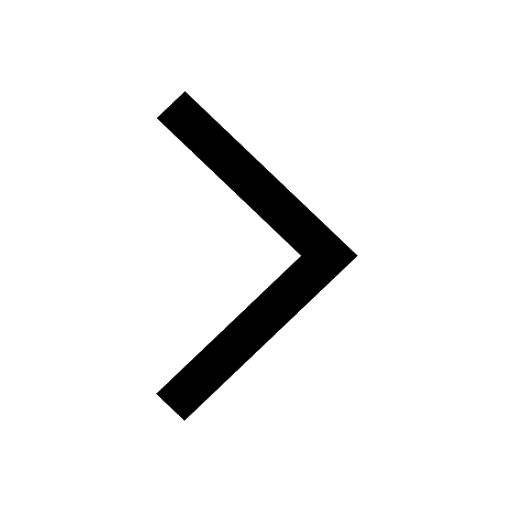
Write a letter to the principal requesting him to grant class 10 english CBSE
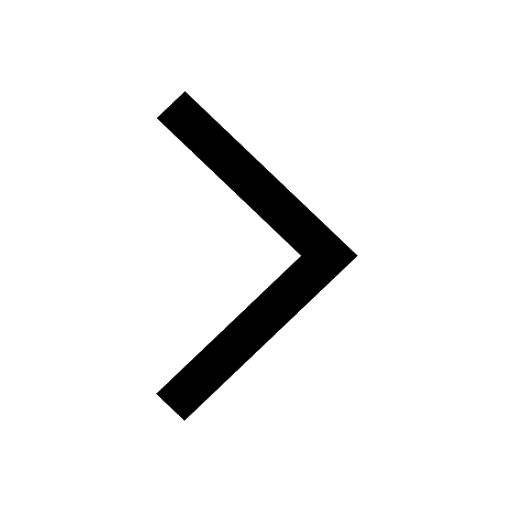
Change the following sentences into negative and interrogative class 10 english CBSE
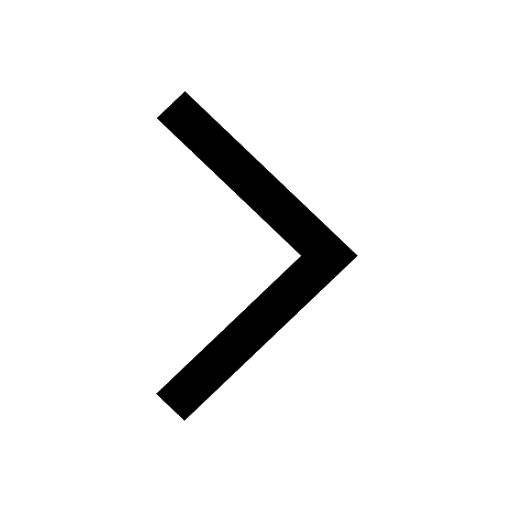
Fill in the blanks A 1 lakh ten thousand B 1 million class 9 maths CBSE
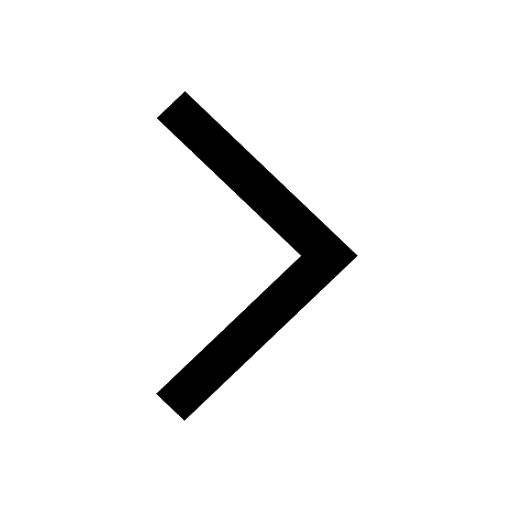