
Answer
479.7k+ views
Hint: $'a'$ can be found out by using the formula for minimum value of a quadratic polynomial. We can use the L' Hopital rule to find $'b'$ . The required answer in the form of summation is a Geometric progression.
In the question, it is given $a=\min \left\{ {{x}^{2}}+2x+3,x\in R \right\}$
$\Rightarrow a=$ minimum value of ${{x}^{2}}+2x+3$
For a quadratic polynomial $a{{x}^{2}}+bx+c$, the minimum value is given by the formula,
$\dfrac{-\left( {{b}^{2}}-4ac \right)}{4a}.............\left( 1 \right)$
Since the polynomial given in the question is ${{x}^{2}}+2x+3$, substituting $a=1,b=2,c=3$ in equation $\left( 1 \right)$, we get,
$\begin{align}
& a=\dfrac{-\left( {{\left( 2 \right)}^{2}}-4\left( 1 \right)\left( 3 \right) \right)}{4\left( 1 \right)} \\
& \Rightarrow a=\dfrac{-\left( 4-12 \right)}{4} \\
& \Rightarrow a=\dfrac{-\left( -8 \right)}{4} \\
& \Rightarrow a=\dfrac{8}{4} \\
& \Rightarrow a=2...........\left( 2 \right) \\
\end{align}$
Also, it is given in the question $b=\underset{\theta \to 0}{\mathop{\lim }}\,\dfrac{1-\cos \theta }{{{\theta }^{2}}}$. If we substitute $\theta =0$ in the limit function, we can notice that this limit is of the form \[\dfrac{0}{0}\]. Since this limit is of the form \[\dfrac{0}{0}\], we can use L’ Hopital rule to solve this limit. In L’ Hopital rule, we individually differentiate the numerator and the denominator with respect to the limit variable i.e. $\theta $ in this case and then apply the limit again.
Applying L’ Hopital rule on $b$, we get,
$\begin{align}
& b=\underset{\theta \to 0}{\mathop{\lim }}\,\dfrac{\dfrac{d\left( 1-\cos \theta \right)}{d\theta }}{\dfrac{d{{\theta }^{2}}}{d\theta }} \\
& \Rightarrow b=\underset{\theta \to 0}{\mathop{\lim }}\,\dfrac{\sin \theta }{2\theta } \\
& \Rightarrow b=\dfrac{1}{2}\underset{\theta \to 0}{\mathop{\lim }}\,\dfrac{\sin \theta }{\theta }...........\left( 3 \right) \\
\end{align}$
There is a formula $\underset{\theta \to 0}{\mathop{\lim }}\,\dfrac{\sin \theta }{\theta }=1$. Substituting $\underset{\theta \to 0}{\mathop{\lim }}\,\dfrac{\sin \theta }{\theta }=1$ in equation $\left( 3 \right)$, we get,
$b=\dfrac{1}{2}...........\left( 4 \right)$
In the question, it is asked to find the value of $\sum\limits_{r=0}^{n}{{{a}^{r}}.{{b}^{n-r}}}$. Substituting equation $\left( 2 \right)$ and equation $\left( 4 \right)$ in $\sum\limits_{r=0}^{n}{{{a}^{r}}.{{b}^{n-r}}}$, we get,
\[\begin{align}
& \sum\limits_{r=0}^{n}{{{a}^{r}}.{{b}^{n-r}}}=\sum\limits_{r=0}^{n}{{{2}^{r}}.\dfrac{1}{{{2}^{n-r}}}} \\
& \Rightarrow \sum\limits_{r=0}^{n}{{{a}^{r}}.{{b}^{n-r}}}=\sum\limits_{r=0}^{n}{{{2}^{r-\left( n-r \right)}}} \\
& \Rightarrow \sum\limits_{r=0}^{n}{{{a}^{r}}.{{b}^{n-r}}}=\sum\limits_{r=0}^{n}{{{2}^{r-n+r}}} \\
& \Rightarrow \sum\limits_{r=0}^{n}{{{a}^{r}}.{{b}^{n-r}}}=\sum\limits_{r=0}^{n}{{{2}^{2r-n}}} \\
& \Rightarrow \sum\limits_{r=0}^{n}{{{a}^{r}}.{{b}^{n-r}}}=\sum\limits_{r=0}^{n}{\dfrac{{{2}^{2r}}}{{{2}^{n}}}} \\
& \Rightarrow \sum\limits_{r=0}^{n}{{{a}^{r}}.{{b}^{n-r}}}=\sum\limits_{r=0}^{n}{\dfrac{{{4}^{r}}}{{{2}^{n}}}} \\
\end{align}\]
Since the limits of this summation is with respect to $r$, we can take $\dfrac{1}{{{2}^{n}}}$ out of the summation.
\[\Rightarrow \sum\limits_{r=0}^{n}{{{a}^{r}}.{{b}^{n-r}}}=\dfrac{1}{{{2}^{n}}}\sum\limits_{r=0}^{n}{{{4}^{r}}}\]
Evaluating the summation, we get,
\[\begin{align}
& \Rightarrow \sum\limits_{r=0}^{n}{{{a}^{r}}.{{b}^{n-r}}}=\dfrac{1}{{{2}^{n}}}\left( {{4}^{0}}+{{4}^{1}}+{{4}^{2}}+{{4}^{3}}+............+{{4}^{n}} \right) \\
& \Rightarrow \sum\limits_{r=0}^{n}{{{a}^{r}}.{{b}^{n-r}}}=\dfrac{1}{{{2}^{n}}}\left( 1+{{4}^{1}}+{{4}^{2}}+{{4}^{3}}+............+{{4}^{n}} \right).........\left( 5 \right) \\
& \\
\end{align}\]
The above series is a geometric progression of which we have to calculate the sum.
The sum of the G.P. $a,ar,a{{r}^{2}},a{{r}^{3}},............,a{{r}^{x}}$ is given by the formula,
$S=\dfrac{a\left( {{r}^{x}}-1 \right)}{r-1}........\left( 6 \right)$
From equation $\left( 5 \right)$, substituting $a=1,r=4,x=n+1$ in equation $\left( 6 \right)$, we get,
\[\begin{align}
& \sum\limits_{r=0}^{n}{{{a}^{r}}.{{b}^{n-r}}}=\dfrac{1}{{{2}^{n}}}\left( \dfrac{1\left( {{4}^{n+1}}-1 \right)}{4-1} \right) \\
& \Rightarrow \sum\limits_{r=0}^{n}{{{a}^{r}}.{{b}^{n-r}}}=\dfrac{1}{{{2}^{n}}}\left( \dfrac{{{4}^{n+1}}-1}{3} \right) \\
& \Rightarrow \sum\limits_{r=0}^{n}{{{a}^{r}}.{{b}^{n-r}}}=\dfrac{{{4}^{n+1}}-1}{{{2}^{n}}.3} \\
\end{align}\]
So the answer is option (c)
Note: There is a possibility of error while finding the value of $b$, since it involves derivative of $\cos x$ which is equal to $-\sin x$. But sometimes, we may get confused while applying the negative sign and may write the derivative of $\cos x$ as $\sin x$ which will lead to an incorrect answer.
In the question, it is given $a=\min \left\{ {{x}^{2}}+2x+3,x\in R \right\}$
$\Rightarrow a=$ minimum value of ${{x}^{2}}+2x+3$
For a quadratic polynomial $a{{x}^{2}}+bx+c$, the minimum value is given by the formula,
$\dfrac{-\left( {{b}^{2}}-4ac \right)}{4a}.............\left( 1 \right)$
Since the polynomial given in the question is ${{x}^{2}}+2x+3$, substituting $a=1,b=2,c=3$ in equation $\left( 1 \right)$, we get,
$\begin{align}
& a=\dfrac{-\left( {{\left( 2 \right)}^{2}}-4\left( 1 \right)\left( 3 \right) \right)}{4\left( 1 \right)} \\
& \Rightarrow a=\dfrac{-\left( 4-12 \right)}{4} \\
& \Rightarrow a=\dfrac{-\left( -8 \right)}{4} \\
& \Rightarrow a=\dfrac{8}{4} \\
& \Rightarrow a=2...........\left( 2 \right) \\
\end{align}$
Also, it is given in the question $b=\underset{\theta \to 0}{\mathop{\lim }}\,\dfrac{1-\cos \theta }{{{\theta }^{2}}}$. If we substitute $\theta =0$ in the limit function, we can notice that this limit is of the form \[\dfrac{0}{0}\]. Since this limit is of the form \[\dfrac{0}{0}\], we can use L’ Hopital rule to solve this limit. In L’ Hopital rule, we individually differentiate the numerator and the denominator with respect to the limit variable i.e. $\theta $ in this case and then apply the limit again.
Applying L’ Hopital rule on $b$, we get,
$\begin{align}
& b=\underset{\theta \to 0}{\mathop{\lim }}\,\dfrac{\dfrac{d\left( 1-\cos \theta \right)}{d\theta }}{\dfrac{d{{\theta }^{2}}}{d\theta }} \\
& \Rightarrow b=\underset{\theta \to 0}{\mathop{\lim }}\,\dfrac{\sin \theta }{2\theta } \\
& \Rightarrow b=\dfrac{1}{2}\underset{\theta \to 0}{\mathop{\lim }}\,\dfrac{\sin \theta }{\theta }...........\left( 3 \right) \\
\end{align}$
There is a formula $\underset{\theta \to 0}{\mathop{\lim }}\,\dfrac{\sin \theta }{\theta }=1$. Substituting $\underset{\theta \to 0}{\mathop{\lim }}\,\dfrac{\sin \theta }{\theta }=1$ in equation $\left( 3 \right)$, we get,
$b=\dfrac{1}{2}...........\left( 4 \right)$
In the question, it is asked to find the value of $\sum\limits_{r=0}^{n}{{{a}^{r}}.{{b}^{n-r}}}$. Substituting equation $\left( 2 \right)$ and equation $\left( 4 \right)$ in $\sum\limits_{r=0}^{n}{{{a}^{r}}.{{b}^{n-r}}}$, we get,
\[\begin{align}
& \sum\limits_{r=0}^{n}{{{a}^{r}}.{{b}^{n-r}}}=\sum\limits_{r=0}^{n}{{{2}^{r}}.\dfrac{1}{{{2}^{n-r}}}} \\
& \Rightarrow \sum\limits_{r=0}^{n}{{{a}^{r}}.{{b}^{n-r}}}=\sum\limits_{r=0}^{n}{{{2}^{r-\left( n-r \right)}}} \\
& \Rightarrow \sum\limits_{r=0}^{n}{{{a}^{r}}.{{b}^{n-r}}}=\sum\limits_{r=0}^{n}{{{2}^{r-n+r}}} \\
& \Rightarrow \sum\limits_{r=0}^{n}{{{a}^{r}}.{{b}^{n-r}}}=\sum\limits_{r=0}^{n}{{{2}^{2r-n}}} \\
& \Rightarrow \sum\limits_{r=0}^{n}{{{a}^{r}}.{{b}^{n-r}}}=\sum\limits_{r=0}^{n}{\dfrac{{{2}^{2r}}}{{{2}^{n}}}} \\
& \Rightarrow \sum\limits_{r=0}^{n}{{{a}^{r}}.{{b}^{n-r}}}=\sum\limits_{r=0}^{n}{\dfrac{{{4}^{r}}}{{{2}^{n}}}} \\
\end{align}\]
Since the limits of this summation is with respect to $r$, we can take $\dfrac{1}{{{2}^{n}}}$ out of the summation.
\[\Rightarrow \sum\limits_{r=0}^{n}{{{a}^{r}}.{{b}^{n-r}}}=\dfrac{1}{{{2}^{n}}}\sum\limits_{r=0}^{n}{{{4}^{r}}}\]
Evaluating the summation, we get,
\[\begin{align}
& \Rightarrow \sum\limits_{r=0}^{n}{{{a}^{r}}.{{b}^{n-r}}}=\dfrac{1}{{{2}^{n}}}\left( {{4}^{0}}+{{4}^{1}}+{{4}^{2}}+{{4}^{3}}+............+{{4}^{n}} \right) \\
& \Rightarrow \sum\limits_{r=0}^{n}{{{a}^{r}}.{{b}^{n-r}}}=\dfrac{1}{{{2}^{n}}}\left( 1+{{4}^{1}}+{{4}^{2}}+{{4}^{3}}+............+{{4}^{n}} \right).........\left( 5 \right) \\
& \\
\end{align}\]
The above series is a geometric progression of which we have to calculate the sum.
The sum of the G.P. $a,ar,a{{r}^{2}},a{{r}^{3}},............,a{{r}^{x}}$ is given by the formula,
$S=\dfrac{a\left( {{r}^{x}}-1 \right)}{r-1}........\left( 6 \right)$
From equation $\left( 5 \right)$, substituting $a=1,r=4,x=n+1$ in equation $\left( 6 \right)$, we get,
\[\begin{align}
& \sum\limits_{r=0}^{n}{{{a}^{r}}.{{b}^{n-r}}}=\dfrac{1}{{{2}^{n}}}\left( \dfrac{1\left( {{4}^{n+1}}-1 \right)}{4-1} \right) \\
& \Rightarrow \sum\limits_{r=0}^{n}{{{a}^{r}}.{{b}^{n-r}}}=\dfrac{1}{{{2}^{n}}}\left( \dfrac{{{4}^{n+1}}-1}{3} \right) \\
& \Rightarrow \sum\limits_{r=0}^{n}{{{a}^{r}}.{{b}^{n-r}}}=\dfrac{{{4}^{n+1}}-1}{{{2}^{n}}.3} \\
\end{align}\]
So the answer is option (c)
Note: There is a possibility of error while finding the value of $b$, since it involves derivative of $\cos x$ which is equal to $-\sin x$. But sometimes, we may get confused while applying the negative sign and may write the derivative of $\cos x$ as $\sin x$ which will lead to an incorrect answer.
Recently Updated Pages
How many sigma and pi bonds are present in HCequiv class 11 chemistry CBSE
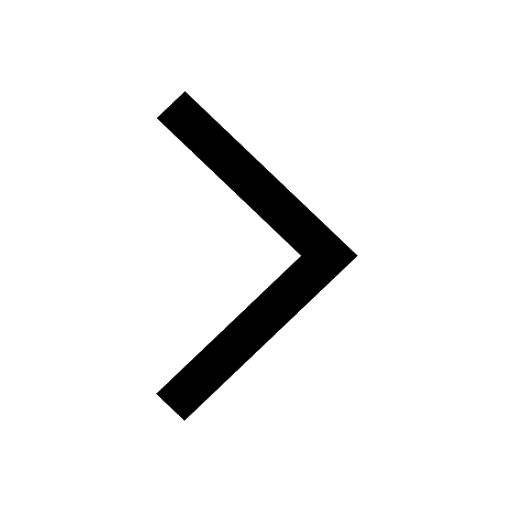
Mark and label the given geoinformation on the outline class 11 social science CBSE
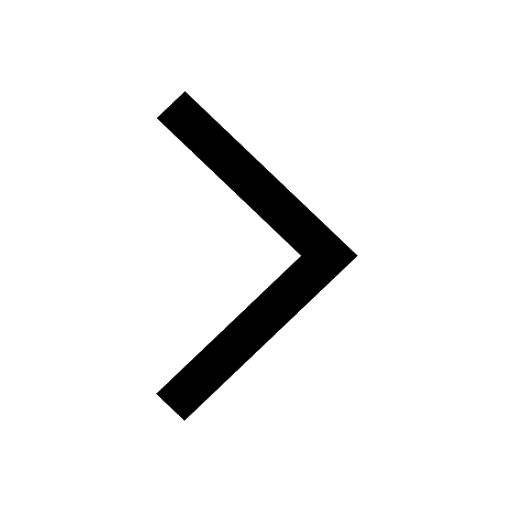
When people say No pun intended what does that mea class 8 english CBSE
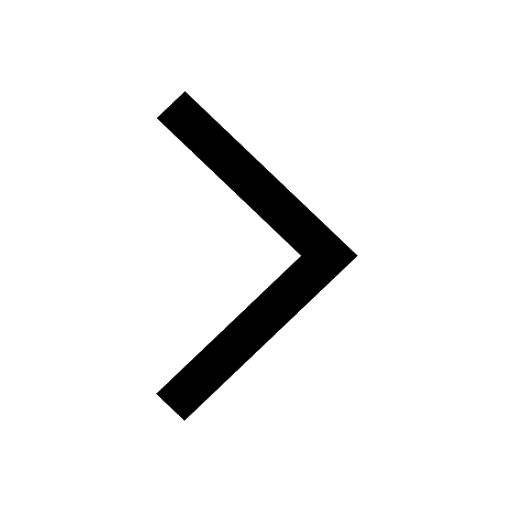
Name the states which share their boundary with Indias class 9 social science CBSE
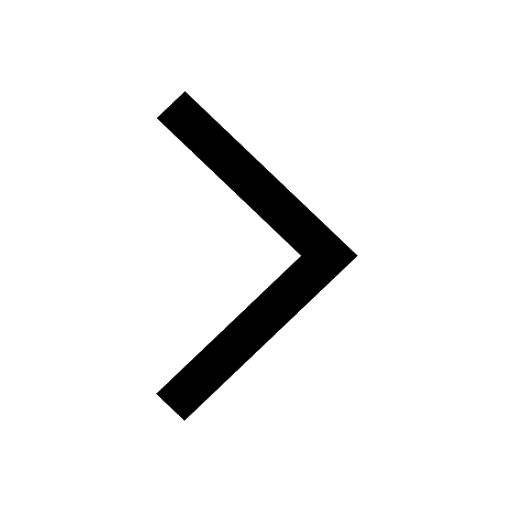
Give an account of the Northern Plains of India class 9 social science CBSE
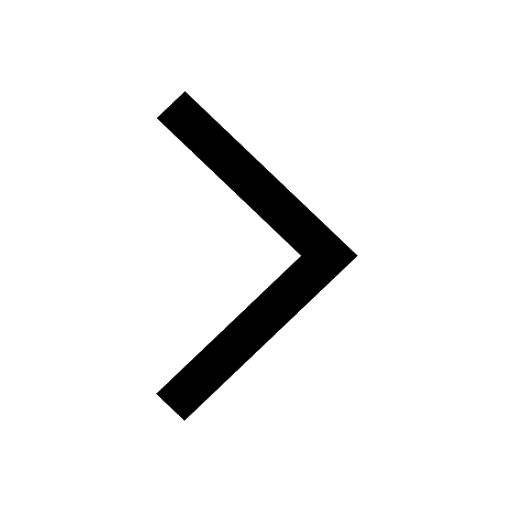
Change the following sentences into negative and interrogative class 10 english CBSE
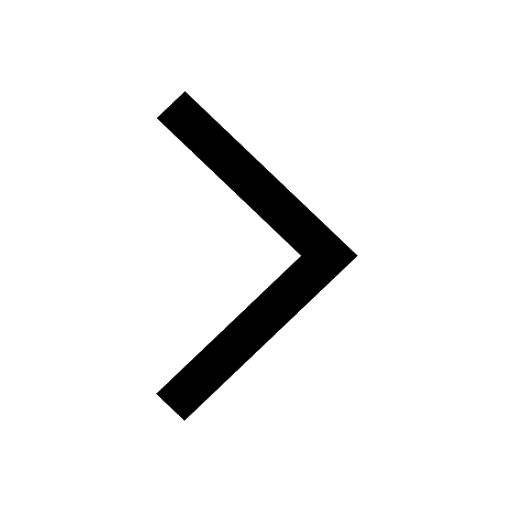
Trending doubts
Fill the blanks with the suitable prepositions 1 The class 9 english CBSE
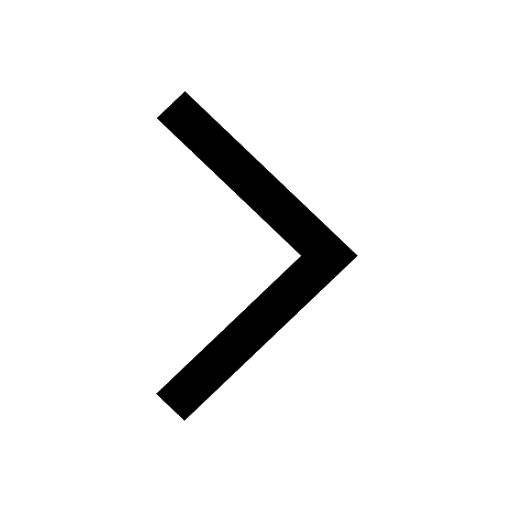
Give 10 examples for herbs , shrubs , climbers , creepers
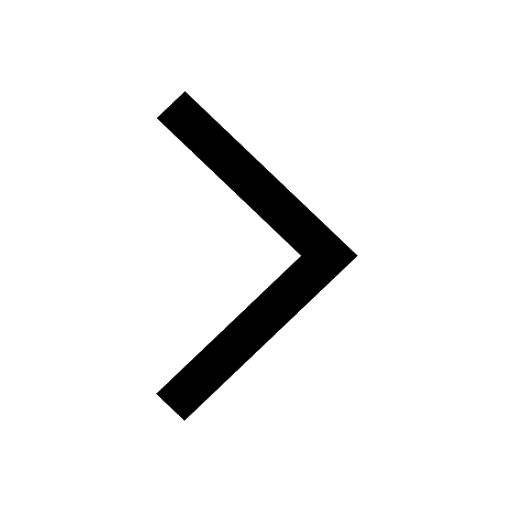
Change the following sentences into negative and interrogative class 10 english CBSE
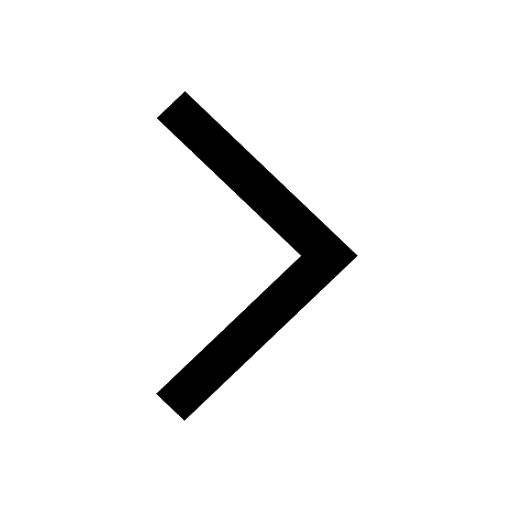
Difference between Prokaryotic cell and Eukaryotic class 11 biology CBSE
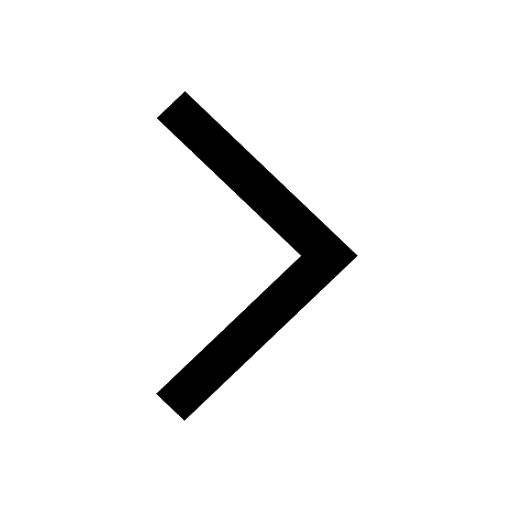
The Equation xxx + 2 is Satisfied when x is Equal to Class 10 Maths
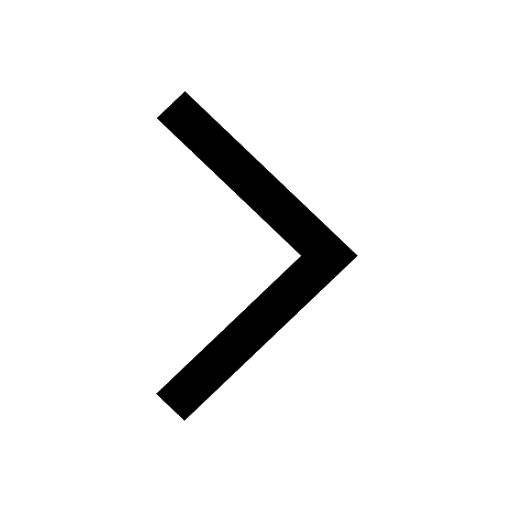
How do you graph the function fx 4x class 9 maths CBSE
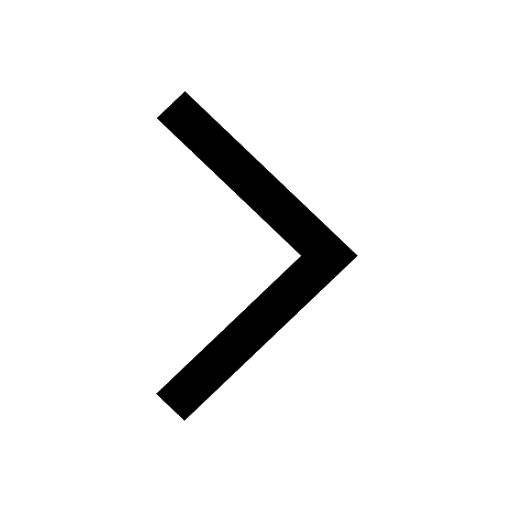
Differentiate between homogeneous and heterogeneous class 12 chemistry CBSE
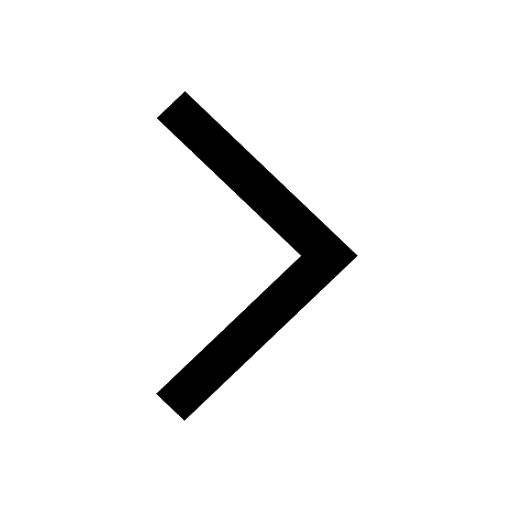
Application to your principal for the character ce class 8 english CBSE
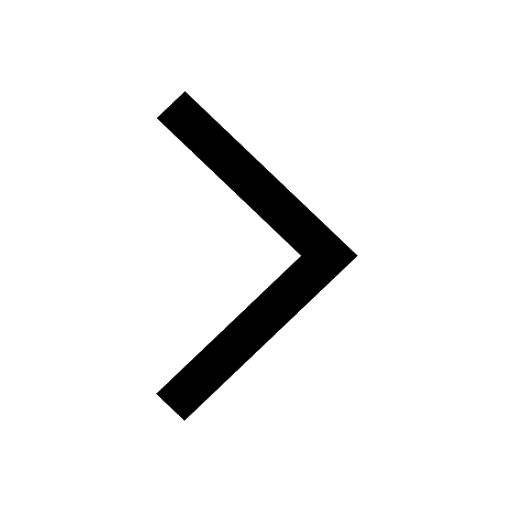
Write a letter to the principal requesting him to grant class 10 english CBSE
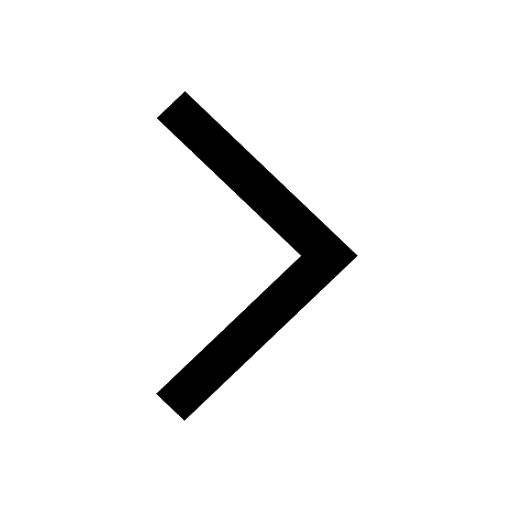