
Answer
377.7k+ views
Hint:Use the superposition principle, to find the electric potential at a point in a system with multiple charges. Use the known geometrical relationships to find the distances between the charges and the point.
Complete step by step answer:
Let us first label the points in the diagram, to refer to them easily while using geometrical relationships.
We are asked to find the electric potential at the point P due to the charges $ + q, - q{\text{ and }} + 3q$. We will first find out the potential at P due to a single charge and then use the superposition principle to find the collective electric potential at P. And for this we need to know the distances $AP,BP,CP$.
We are given that $\vartriangle ABC$ is a right-angled triangle and point P is the midpoint of $AC$. So, by using Pythagoras theorem we have, $AC = \sqrt 2 a$ and $AP = PC = \dfrac{a}{{\sqrt 2 }}$. We can also observe that $\vartriangle ABP \cong \vartriangle CBP$ [by SSS congruence criterion]. And so, $\angle ABP = \angle CBP = 45^\circ $. Also, since $\vartriangle ABC$ is isosceles we have $\angle A = \angle C = 45^\circ $. Now since, sides opposite to opposite angles are equal, in $\vartriangle ABP$, we have $AP = BP = \dfrac{a}{{\sqrt 2 }}$ [because $\angle ABP = \angle BAP = 45^\circ $].
So, we now know the distances between the charges and the point P;
$AP = BP = CP = \dfrac{a}{{\sqrt 2 }}$
So, the electric potential at P due to $ + q$ is,
${V_1} = \dfrac{q}{{4\pi {\varepsilon _0}\dfrac{a}{{\sqrt 2 }}}} = \dfrac{q}{{2\sqrt 2 \pi {\varepsilon _0}a}}$
Electric potential at P due to $ - q$ is,
${V_2} = \dfrac{{ - q}}{{4\pi {\varepsilon _0}\dfrac{a}{{\sqrt 2 }}}} = \dfrac{{ - q}}{{2\sqrt 2 \pi {\varepsilon _0}a}}$
And, electric potential at P due to $ + q$ is,
${V_3} = \dfrac{{3q}}{{4\pi {\varepsilon _0}\dfrac{a}{{\sqrt 2 }}}} \\
\Rightarrow {V_3}= \dfrac{{3q}}{{2\sqrt 2 \pi {\varepsilon _0}a}}$
We know from the superposition of electric potential that the electric potential of a system of charges is the sum of potentials of the individual charges.
So, the electric potential at P $V = {V_1} + {V_2} + {V_3}$.
$V = \dfrac{q}{{2\sqrt 2 \pi {\varepsilon _0}a}} + \dfrac{{ - q}}{{2\sqrt 2 \pi {\varepsilon _0}a}} + \dfrac{{3q}}{{2\sqrt 2 \pi {\varepsilon _0}a}}$
$\therefore V = \dfrac{{3q}}{{2\sqrt 2 \pi {\varepsilon _0}a}}$
Hence, the answer is option D.
Note:The electric potential at a point is the amount of work which is needed to move a charge from a reference point to that point. Usually, we consider the reference point at infinity. Whereas, potential difference between two points is the amount of work that needs to be done to bring a charge from one point to the other.
Complete step by step answer:
Let us first label the points in the diagram, to refer to them easily while using geometrical relationships.

We are asked to find the electric potential at the point P due to the charges $ + q, - q{\text{ and }} + 3q$. We will first find out the potential at P due to a single charge and then use the superposition principle to find the collective electric potential at P. And for this we need to know the distances $AP,BP,CP$.
We are given that $\vartriangle ABC$ is a right-angled triangle and point P is the midpoint of $AC$. So, by using Pythagoras theorem we have, $AC = \sqrt 2 a$ and $AP = PC = \dfrac{a}{{\sqrt 2 }}$. We can also observe that $\vartriangle ABP \cong \vartriangle CBP$ [by SSS congruence criterion]. And so, $\angle ABP = \angle CBP = 45^\circ $. Also, since $\vartriangle ABC$ is isosceles we have $\angle A = \angle C = 45^\circ $. Now since, sides opposite to opposite angles are equal, in $\vartriangle ABP$, we have $AP = BP = \dfrac{a}{{\sqrt 2 }}$ [because $\angle ABP = \angle BAP = 45^\circ $].
So, we now know the distances between the charges and the point P;
$AP = BP = CP = \dfrac{a}{{\sqrt 2 }}$
So, the electric potential at P due to $ + q$ is,
${V_1} = \dfrac{q}{{4\pi {\varepsilon _0}\dfrac{a}{{\sqrt 2 }}}} = \dfrac{q}{{2\sqrt 2 \pi {\varepsilon _0}a}}$
Electric potential at P due to $ - q$ is,
${V_2} = \dfrac{{ - q}}{{4\pi {\varepsilon _0}\dfrac{a}{{\sqrt 2 }}}} = \dfrac{{ - q}}{{2\sqrt 2 \pi {\varepsilon _0}a}}$
And, electric potential at P due to $ + q$ is,
${V_3} = \dfrac{{3q}}{{4\pi {\varepsilon _0}\dfrac{a}{{\sqrt 2 }}}} \\
\Rightarrow {V_3}= \dfrac{{3q}}{{2\sqrt 2 \pi {\varepsilon _0}a}}$
We know from the superposition of electric potential that the electric potential of a system of charges is the sum of potentials of the individual charges.
So, the electric potential at P $V = {V_1} + {V_2} + {V_3}$.
$V = \dfrac{q}{{2\sqrt 2 \pi {\varepsilon _0}a}} + \dfrac{{ - q}}{{2\sqrt 2 \pi {\varepsilon _0}a}} + \dfrac{{3q}}{{2\sqrt 2 \pi {\varepsilon _0}a}}$
$\therefore V = \dfrac{{3q}}{{2\sqrt 2 \pi {\varepsilon _0}a}}$
Hence, the answer is option D.
Note:The electric potential at a point is the amount of work which is needed to move a charge from a reference point to that point. Usually, we consider the reference point at infinity. Whereas, potential difference between two points is the amount of work that needs to be done to bring a charge from one point to the other.
Recently Updated Pages
How many sigma and pi bonds are present in HCequiv class 11 chemistry CBSE
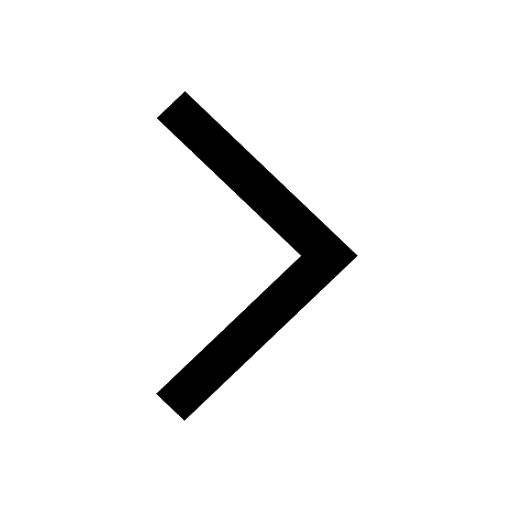
Mark and label the given geoinformation on the outline class 11 social science CBSE
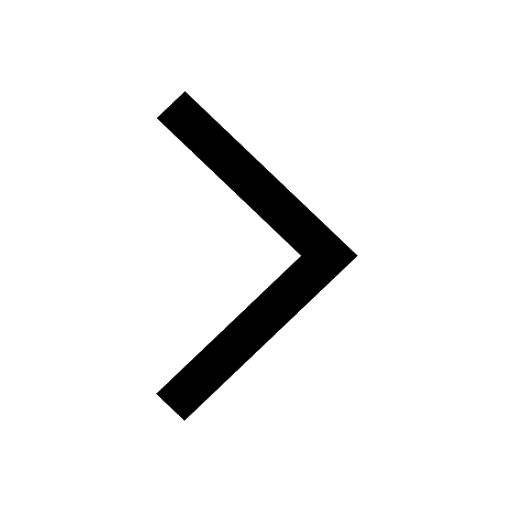
When people say No pun intended what does that mea class 8 english CBSE
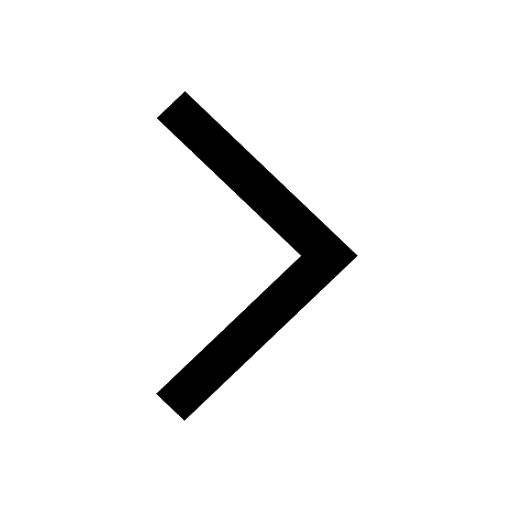
Name the states which share their boundary with Indias class 9 social science CBSE
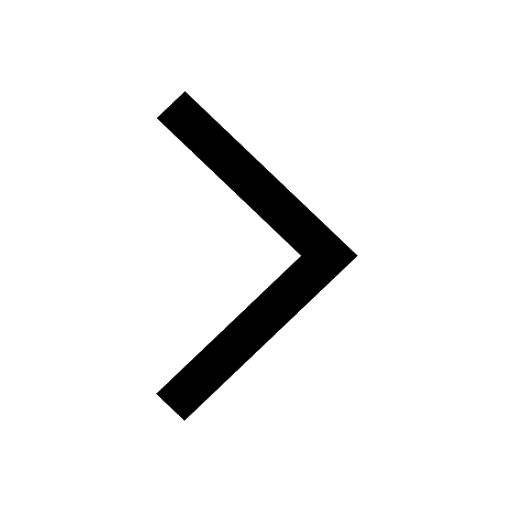
Give an account of the Northern Plains of India class 9 social science CBSE
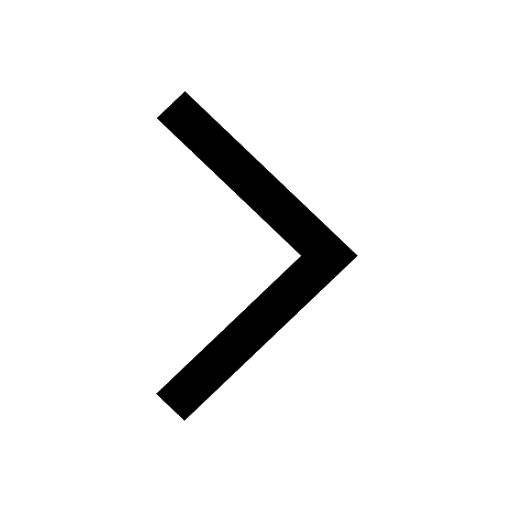
Change the following sentences into negative and interrogative class 10 english CBSE
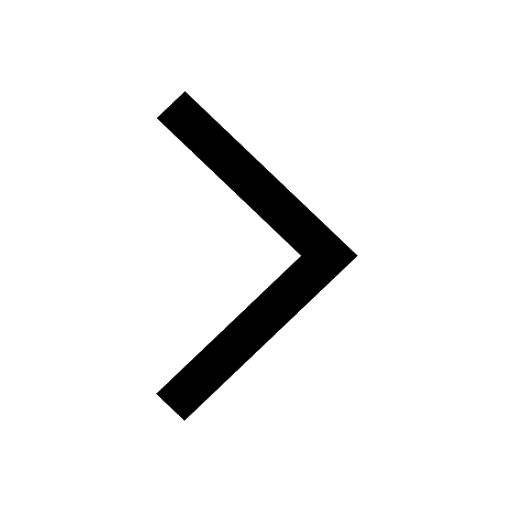
Trending doubts
Fill the blanks with the suitable prepositions 1 The class 9 english CBSE
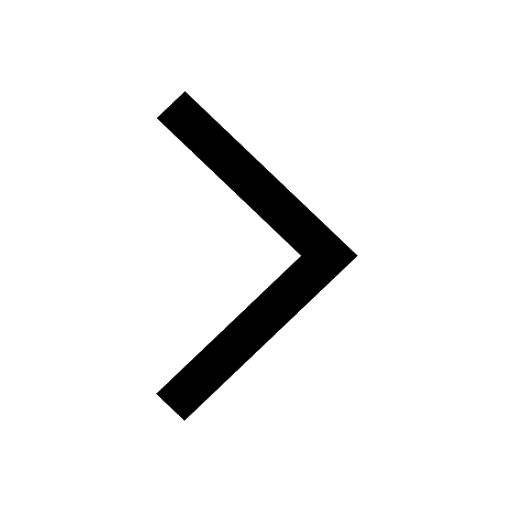
Which are the Top 10 Largest Countries of the World?
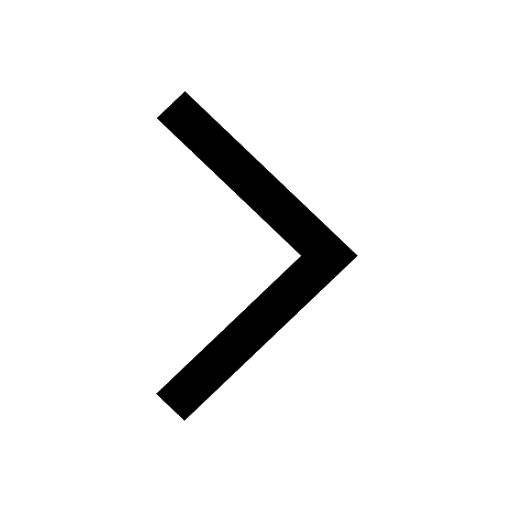
Give 10 examples for herbs , shrubs , climbers , creepers
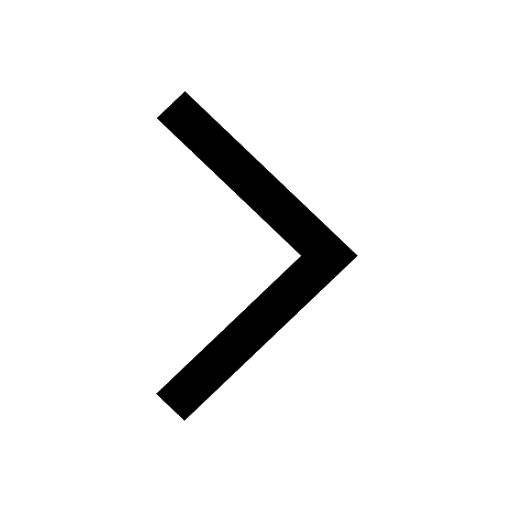
Difference Between Plant Cell and Animal Cell
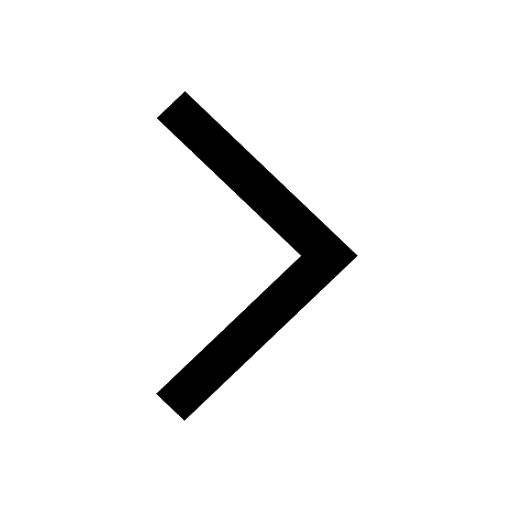
Difference between Prokaryotic cell and Eukaryotic class 11 biology CBSE
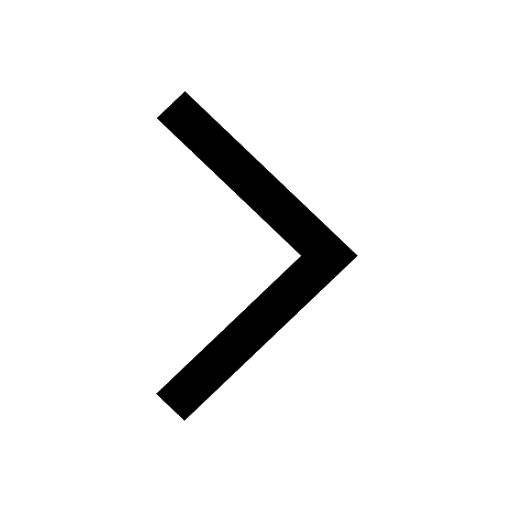
The Equation xxx + 2 is Satisfied when x is Equal to Class 10 Maths
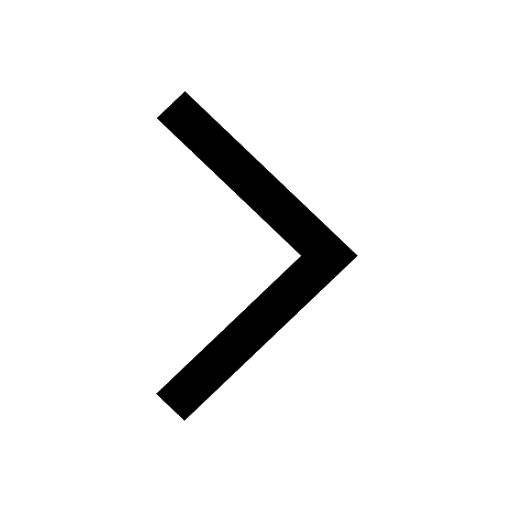
Change the following sentences into negative and interrogative class 10 english CBSE
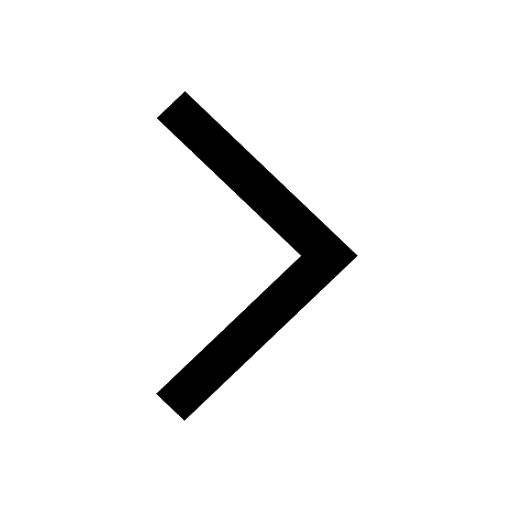
How do you graph the function fx 4x class 9 maths CBSE
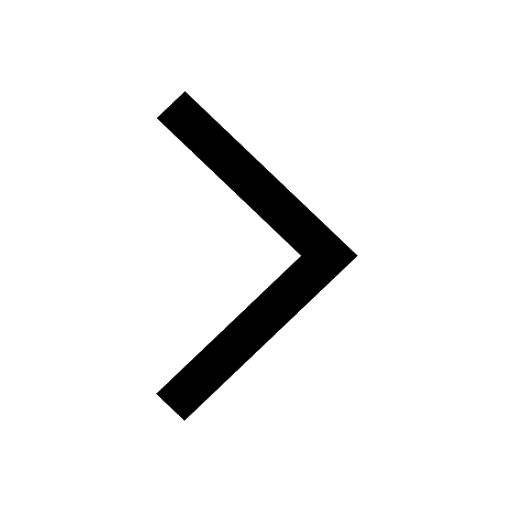
Write a letter to the principal requesting him to grant class 10 english CBSE
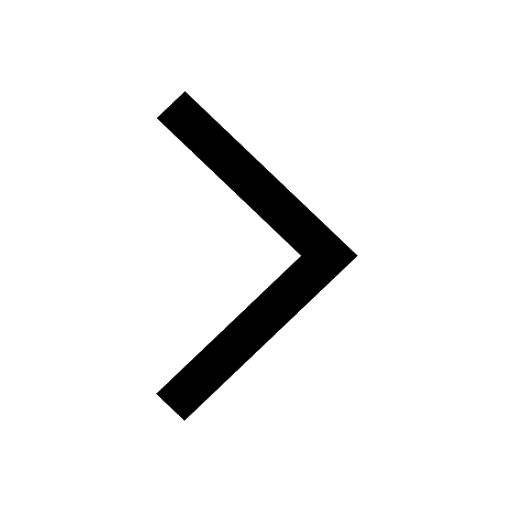