Answer
64.8k+ views
Hint: In the position of the solar eclipse, the moon will attract the earth towards the sun. When the moon moves to the other side, it will attract the earth away from the sun. The acceleration of the earth towards the moon would be directly proportional to the mass of the moon and inversely proportional to the orbital radius.
Formula used: In this solution we will be using the following formulae;
\[a = G\dfrac{M}{{{r^2}}}\] where \[a\] is the acceleration of an object near a gravitational body, \[M\] is the mass of the gravitational body, and \[r\] is the distance of the object to the gravitational body, and \[G\] is the universal gravitational constant.
Complete Step-by-Step Solution:
Generally, it is common knowledge that the moon revolves around the earth due to the gravitational field of the earth attracting the moon. Nevertheless, the moon is also a gravitational body and the earth can act as an object to it.
The acceleration of the earth towards the moon can be given as
\[{a_e} = G\dfrac{{{M_m}}}{{{r^2}}}\] where \[M\] is the mass of the moon, and \[r\] is the distance of the moon to the earth (in this case the orbital radius), and \[G\] is the universal gravitational constant.
Now, when the moon is on the other side, the acceleration will be in the opposite direction, hence the change in acceleration would be
\[\Delta {a_e} = G\dfrac{{{M_m}}}{{{r^2}}} - \left( { - G\dfrac{{{M_m}}}{{{r^2}}}} \right) = 2\dfrac{{G{M_m}}}{{{r^2}}}\] (because acceleration is now in opposite direction relative to the sun)
Hence, by inserting all known values, we have
\[\Delta {a_e} = 2\dfrac{{\left( {6.67 \times {{10}^{ - 11}}} \right)\left( {7.36 \times {{10}^{22}}} \right)}}{{{{\left( {3.28 \times {{10}^8}} \right)}^2}}}\]
By computation, we have
\[\Delta {a_e} = 9.12 \times {10^{ - 5}}m/{s^2}\]
Hence, the correct option is D
Note: In the above, we assumed that the distance of the earth to the moon during the ellipse is the same as the distance on the other side. In actuality, this is not necessarily so, as the orbit of the moon is not a perfect circle. Also, although small, the earth actually shifts towards the moon as the moon is orbiting about it, allowing the earth itself to have an orbit about a point.
Formula used: In this solution we will be using the following formulae;
\[a = G\dfrac{M}{{{r^2}}}\] where \[a\] is the acceleration of an object near a gravitational body, \[M\] is the mass of the gravitational body, and \[r\] is the distance of the object to the gravitational body, and \[G\] is the universal gravitational constant.
Complete Step-by-Step Solution:
Generally, it is common knowledge that the moon revolves around the earth due to the gravitational field of the earth attracting the moon. Nevertheless, the moon is also a gravitational body and the earth can act as an object to it.
The acceleration of the earth towards the moon can be given as
\[{a_e} = G\dfrac{{{M_m}}}{{{r^2}}}\] where \[M\] is the mass of the moon, and \[r\] is the distance of the moon to the earth (in this case the orbital radius), and \[G\] is the universal gravitational constant.
Now, when the moon is on the other side, the acceleration will be in the opposite direction, hence the change in acceleration would be
\[\Delta {a_e} = G\dfrac{{{M_m}}}{{{r^2}}} - \left( { - G\dfrac{{{M_m}}}{{{r^2}}}} \right) = 2\dfrac{{G{M_m}}}{{{r^2}}}\] (because acceleration is now in opposite direction relative to the sun)
Hence, by inserting all known values, we have
\[\Delta {a_e} = 2\dfrac{{\left( {6.67 \times {{10}^{ - 11}}} \right)\left( {7.36 \times {{10}^{22}}} \right)}}{{{{\left( {3.28 \times {{10}^8}} \right)}^2}}}\]
By computation, we have
\[\Delta {a_e} = 9.12 \times {10^{ - 5}}m/{s^2}\]
Hence, the correct option is D
Note: In the above, we assumed that the distance of the earth to the moon during the ellipse is the same as the distance on the other side. In actuality, this is not necessarily so, as the orbit of the moon is not a perfect circle. Also, although small, the earth actually shifts towards the moon as the moon is orbiting about it, allowing the earth itself to have an orbit about a point.
Recently Updated Pages
Write a composition in approximately 450 500 words class 10 english JEE_Main
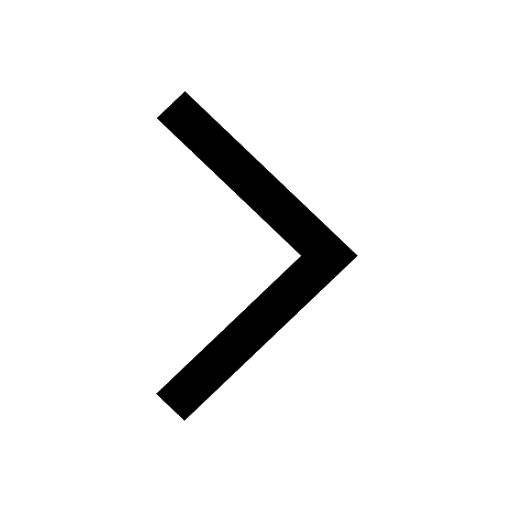
Arrange the sentences P Q R between S1 and S5 such class 10 english JEE_Main
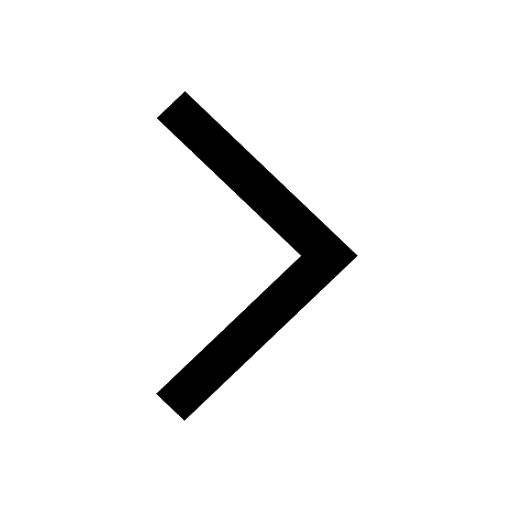
What is the common property of the oxides CONO and class 10 chemistry JEE_Main
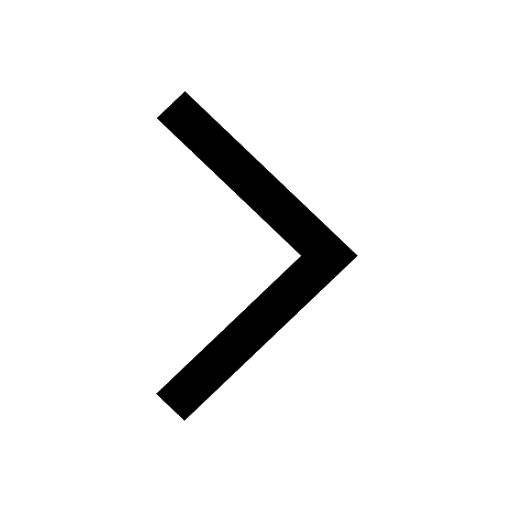
What happens when dilute hydrochloric acid is added class 10 chemistry JEE_Main
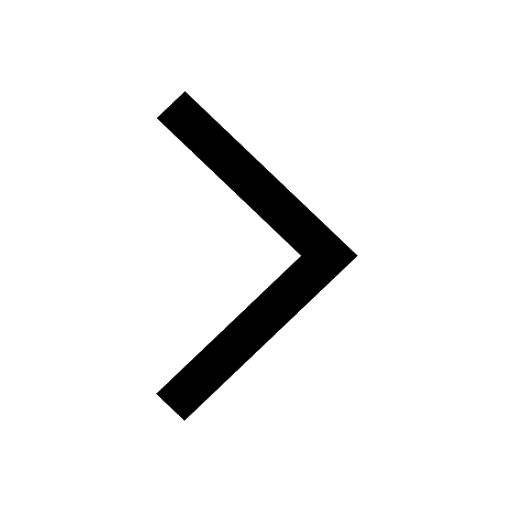
If four points A63B 35C4 2 and Dx3x are given in such class 10 maths JEE_Main
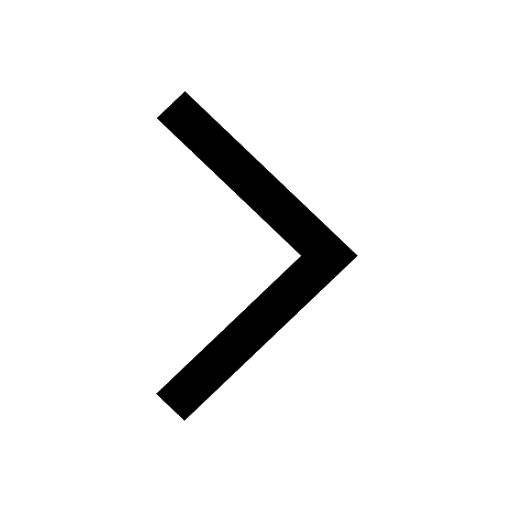
The area of square inscribed in a circle of diameter class 10 maths JEE_Main
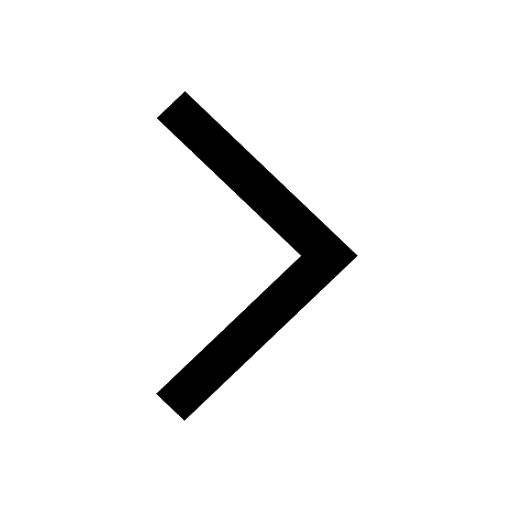
Other Pages
Excluding stoppages the speed of a bus is 54 kmph and class 11 maths JEE_Main
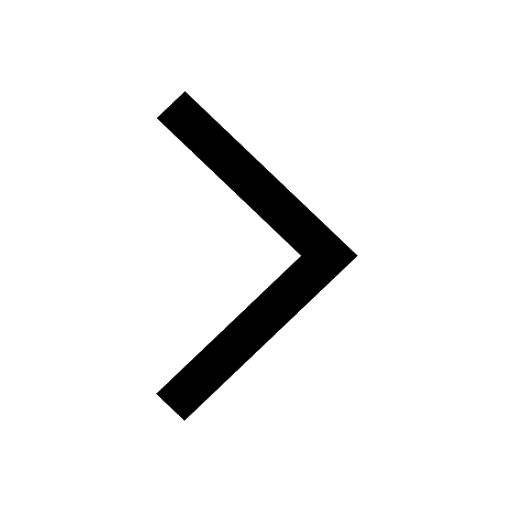
In the ground state an element has 13 electrons in class 11 chemistry JEE_Main
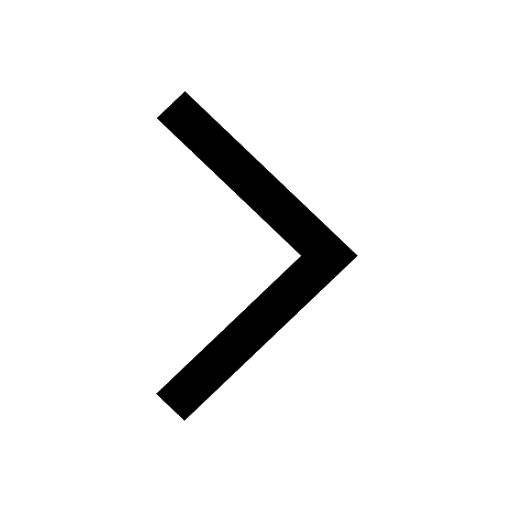
Electric field due to uniformly charged sphere class 12 physics JEE_Main
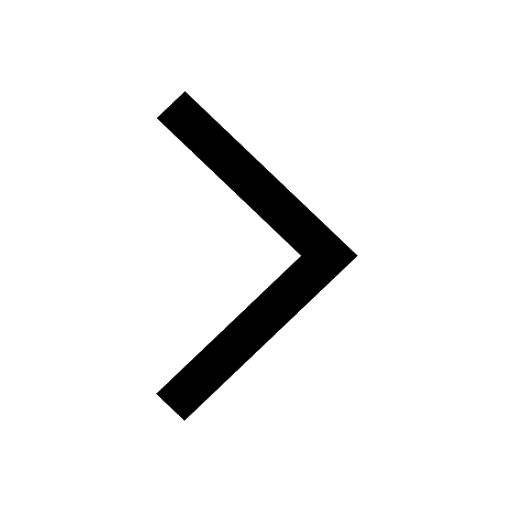
A boat takes 2 hours to go 8 km and come back to a class 11 physics JEE_Main
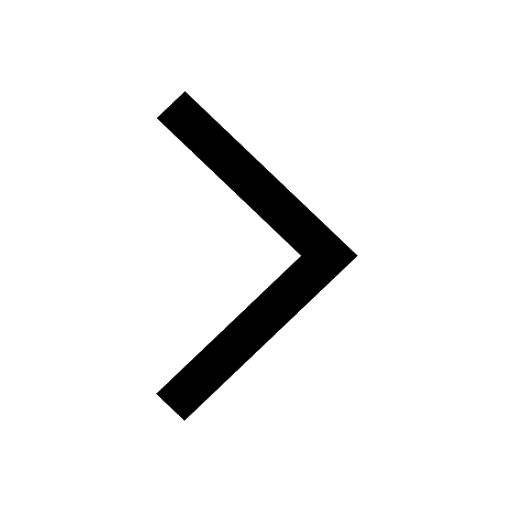
According to classical free electron theory A There class 11 physics JEE_Main
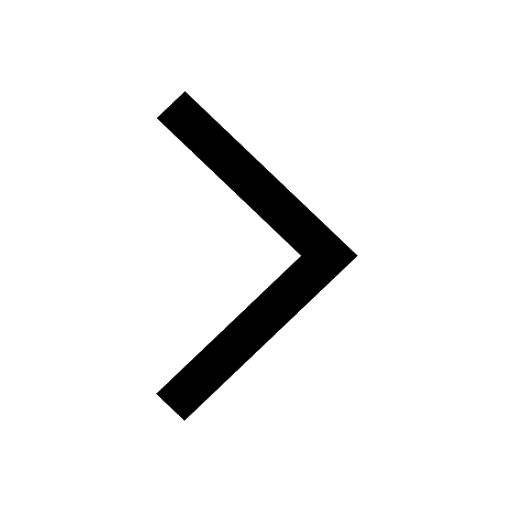
Differentiate between homogeneous and heterogeneous class 12 chemistry JEE_Main
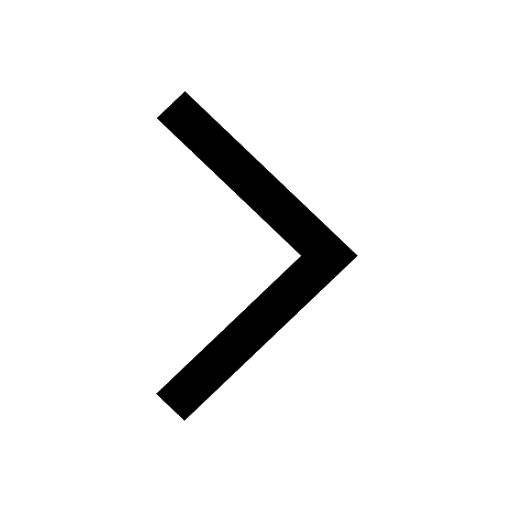