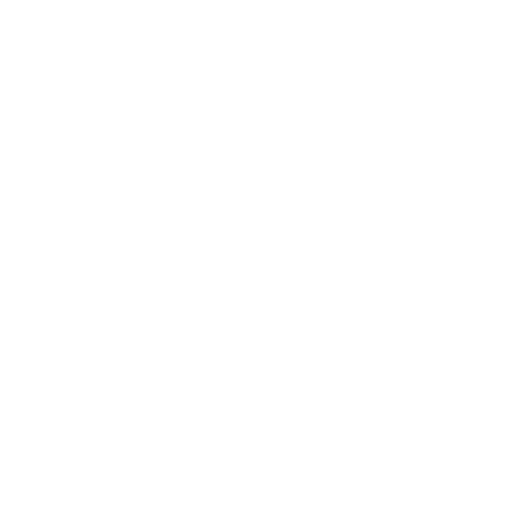

A Complete Guide to the Time Period of SHM: Definition, Formula, Derivation, and Its Importance
The Time Period of Simple Harmonic Motion (SHM) is a fundamental concept in physics and important for JEE Main, describing the regular back-and-forth motion of an object around an equilibrium point. Whether it's a pendulum swinging, a spring oscillating, or molecules vibrating, SHM is all around us. The time period, which represents the time taken for one complete oscillation, is crucial for understanding the behaviour of oscillatory systems. This concept not only helps in studying physical phenomena but also has applications in engineering, acoustics, and even timekeeping. Before Understanding deeply about Time period let us first understand about SHM.
What is Simple Harmonic Motion?
SHM is defined as a motion in which the magnitude of the restoring force is directly proportional to the body's displacement from its mean position. It is always directed towards the mean position. Furthermore, all simple harmonic motions are periodic, but not all periodic motions are simple harmonic motions. The acceleration of a particle in simple harmonic motion is given by
Terms Related to SHM
Mean Position
The point at which the particle's net force is zero.
Mean Position Conditions
The force acting on the particle is inversely proportional to the displacement. As a result, this point of equilibrium will be stable.
SHM Amplitude
It is the particle's maximum deviation from its mean position.
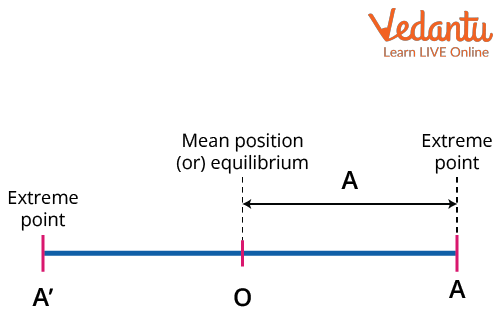
SHM diagram
Time Period of SHM
The time period of a particle executing SHM is defined as the shortest time taken to complete one oscillation or the minimum time after which the particle continues to repeat its motion. The period can be calculated by timing the duration of one complete oscillation where T gives the Time Period of SHM Formula. The two most common simple harmonic motion experiments are:
1. Pendulum: An oscillation with a period of a mass m attached to the end of a pendulum of length l. (T).
2. Mass on a Spring: A mass m attached to a spring with a spring constant k will oscillate with a period (T).
It is worth noting that the period of a pendulum is independent of its mass, whereas the period of a mass on a spring is independent of the length of the spring. A simple harmonic oscillator's period is also independent of its amplitude.
3. Frequency of SHM: The frequency is defined as the number of oscillations per second. Angular frequency
This topic defines the amplitude and time period of simple harmonic motion.
Time Period of SHM Derivation
Consider a spring with one end fixed. When no force is applied to the spring, it remains in its equilibrium position. When we pull the spring outward, it exerts a force that directs it towards the equilibrium position.
When we push the spring inward, a force is generated on it that is directed towards the equilibrium position. In both cases, the spring's force is directed towards the equilibrium position. This is also called the restoring force. Let's call the spring's force 'F' and the spring's displacement from its equilibrium position 'x'.
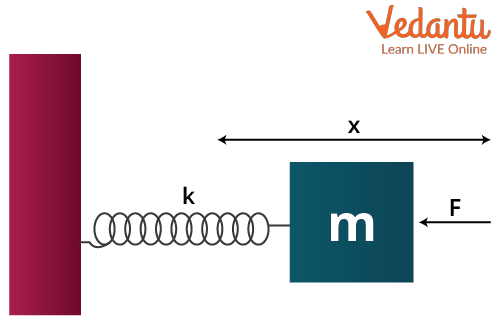
SHM spring system diagram
The restoring force will be given by
F= – kx.
The negative sign denotes that the restoring force acts in the opposite direction.
Here, k is the force constant.
Consider a particle of mass (m) that is executing Simple Harmonic Motion along a path X’OX; and the mean position is at point O. Let the speed of the particle be v0 when it is at position p (at a distance no from O)
At t = 0, the particle at P (moving towards the right)
At t = t, the particle is at Q (at a distance x from O)
With a velocity (v)
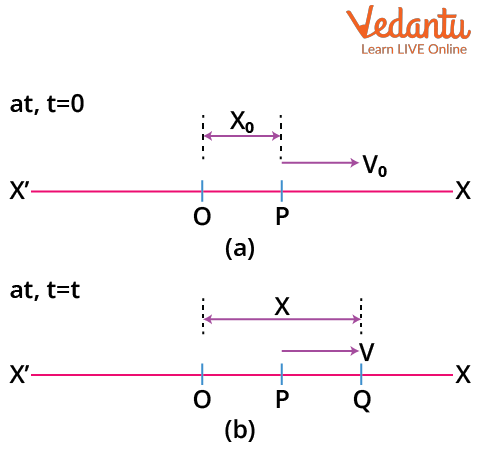
A particle executing SHM
The restoring force at Q is given by
Here, K is the positive constant
Also we have
Let a is the acceleration at Q
This gives
Put,
So,
As
So,
This is the differential equation for linear simple harmonic motion
The time period of SHM is given as
We have already written for a spring system that
This means that the Time Period of SHM Formula
Simple Harmonic Motion Examples
Simple harmonic motion is executed by any object that repeats its motion to and fro about a mean position over time.
Examples:
A basic pendulum.
System of mass springs
A steel ball rolling through a curved dish.
The swinging motion.
Angular Simple Harmonic Motion
An angular oscillation can be produced by a body that is free to rotate about an axis. For example, a photo frame or a calendar hung from a nail on the wall. It produces angular oscillations when slightly pushed from its mean position and then released.
What is Time Period of Angular SHM?
The Time Period of Angular Simple Harmonic Motion (SHM) refers to the time taken for a system undergoing oscillatory rotational motion to complete one full cycle around its equilibrium position. Unlike linear SHM, where the motion is back-and-forth along a straight line, angular SHM involves oscillation around a fixed axis or point. This type of motion is commonly observed in physical pendulums, torsional pendulums, and other systems where angular displacement plays a role. Understanding the time period in angular SHM is essential for studying rotational dynamics and its applications in clocks, machinery, and scientific experiments.
Time Period of Angular SHM Formula
The formula for the time period of angular Simple Harmonic motion is given by
Where:
T = Time period of angular SHM (in seconds)
I = Moment of inertia of the oscillating body about the axis of rotation (in
)k = Restoring torque constant (in
)
This formula shows that the time period depends on the moment of inertia and the restoring torque constant, making it specific to the geometry and physical properties of the system.
Time Period of Angular SHM Derivation
The body must feel a net torque that has been restored in nature. If the oscillation angle is small, the restoring torque will be proportional to the angular displacement. The expression of time period of angular SHM is as follows:
So,
This is the differential equation of an angular simple harmonic motion.
Now, we have that
We know that the time period T is given as
So, the time period for the angular SHM will be
Time Period of a Body Under SHM
The time period of a body under SHM depends on the system’s parameters, such as mass and restoring force. For a spring-mass system or pendulum, these properties determine the duration of one complete oscillation.
Time Period of a Particle Executing SHM
For a particle executing SHM, the time period reflects its periodic motion. Whether in linear or angular oscillation, the time period remains constant, depending on the restoring force and system parameters.
Key Features of Time Period in SHM
Independence from Amplitude: The time period remains constant, irrespective of the amplitude of oscillation, as long as the motion follows SHM.
Dependence on System Properties: It is determined by factors like mass, length, restoring force constant, and gravitational acceleration.
Frequency Relation: The time period is inversely proportional to the frequency of oscillation:
Expression for Time Period of SHM
The expression for the time period of SHM can be summarised as:
Linear SHM (spring-mass system):
Simple Pendulum:
Angular SHM:
Solved Examples on Time Period of SHM
Example 1: The mass and the diameter of a planet are thrice the value of the Earth. The time period of a simple pendulum on the Earth is 10s. What will be its time period of oscillation of the same pendulum on the planet ?
Solution:
Time period of oscillation means the time in which a simple pendulum will complete its one oscillation. Acceleration of the gravity is given by the formula :
Let us suppose that gp and ge are the acceleration of gravity due to the planet and the Earth. Taking the ratio of the both, we have:
as given
and
So,
As T is inversely proportional to the square root of the acceleration due to gravity, so we will have:
Hence, the time period of oscillation of the same pendulum on the planet is
Example 2 : A simple pendulum oscillating in the air has time period T. The bob of the simple pendulum is now completely immersed in the non viscous liquid; given the density of the liquid is (1/8)th of the material of the bob. If the bob is inside the liquid all the time then what is the time period of oscillation of a simple pendulum of the bob in this liquid?
Solution:
The time period of oscillation for a simple pendulum is given according to the relation:
When we will immerse in the non viscous liquid, the acceleration due to gravity will become:
Now, the ratio of the time period of the simple pendulum in the liquid to the time period in the air will be:
So,
Here, g represents gravitational acceleration, T’ represents the time period of the simple pendulum in the liquid and T represents the time period of the simple pendulum in the air.
Applications of Time Period in SHM
Understanding the time period of oscillation helps in designing clocks, studying vibrations in machinery, and analysing natural phenomena like seismic waves.
This concept is fundamental in physics and engineering for predicting and managing oscillatory behaviour.
Conclusion
In short, the period of the simple harmonic motion can be determined by the time duration of one complete oscillation. We've learnt that simple harmonic motion (SHM) is a motion in which the magnitude of the restoring force is directly proportional to the body's displacement from its mean position. This restoring force is always directed towards the mean position. We also discussed the period of the particle's oscillation, its formula, examples and the various factors that help us understand the topic clearly.
JEE Main 2025 Subject-Wise Important Chapters
The JEE Main 2025 subject-wise important chapters provide a focused strategy for Chemistry, Physics, and Maths. These chapters help students prioritise their preparation, ensuring they cover high-weightage topics for better performance in the exam.
Check Other Important Links for JEE Main 2025
Prepare effectively for JEE with our comprehensive study material links, including notes, question papers, sample papers, and mock test series for both JEE Main and Advanced. Access essential resources like formulas, important questions, and preparation tips to boost your performance.
FAQs on Time Period of SHM for JEE Main Physics 2025
1. What is the use of simple harmonic motion?
The following are some real-world applications of SHM:
Clock
Instruments of music
Shock absorbers for automobiles
Jumping from a bungee
Scuba diving board
Hearing procedures
Metronome
Clock
A clock's time is kept by a pendulum or a vibrating quartz crystal. To ensure accurate time, the motion of the pendulum or quartz must be periodic.
Shock Absorbers for Automobiles
Springs attached to car wheels are required for a smooth ride for passengers. The wheels rise as the car passes over a bump in the road. If there are no shock absorbers in the car, the entire vehicle will be thrown up and down, making the passengers uncomfortable.
2. What are the linear SHM conditions?
The linear simple harmonic motion occurs when a particle moves back and forth along a straight line around a fixed point (called the equilibrium position). As an example, consider the spring-mass system. The restoring force or acceleration acting on the particle should always be proportional to the particle's displacement and directed towards the equilibrium position.
The particles’ displacement from their equilibrium position
The particle-acting restoring force
Acceleration of the particle
3. Define Time Period Of SHM.
The time period of SHM is the time taken by an oscillating object to complete one full cycle of its motion and return to its initial position. It is represented by the symbol T and measured in seconds.
4. What is the formula for the Time Period of SHM?
The general formula for the time period of SHM is:
Where m is the mass of the object and k is the spring constant. For a pendulum, it is given by
5. How do you define the Time Period of SHM?
The time period of SHM is defined as the duration required for an oscillating object to complete one full oscillation, including both forward and backward motion.
6. What is the Time Period of Angular SHM?
For angular SHM, the time period is:
Where I is the moment of inertia and k is the restoring torque constant.
7. How is the Time Period of SHM derived?
The time period of SHM is derived from the equation of motion:
F=−kx
Using F=ma and solving the differential equation, the angular frequency is
8. What determines the Time Period of a Body Under SHM?
The time period of a body in SHM is determined by factors like its mass, the restoring force acting on it, and the system’s physical properties such as spring constant or pendulum length.
9. What is the Time Period of a Particle Executing SHM?
The time period of a particle executing SHM depends on its oscillation system. For example, in a spring-mass system:
For a pendulum:
10. What is the Expression for the Time Period of SHM?
The expression for the time period depends on the system:
For a spring-mass system:
For a pendulum:
For angular SHM:
11. What factors affect the Time Period of Oscillation in SHM?
Factors include the mass of the object, the stiffness of the spring (spring constant), the length of the pendulum, and gravitational acceleration. Amplitude does not affect the time period in SHM.
12. Why is the Time Period of SHM constant?
The time period in SHM is constant because it depends only on intrinsic system properties like mass, length, and restoring force constant. This makes the motion independent of amplitude for small oscillations.
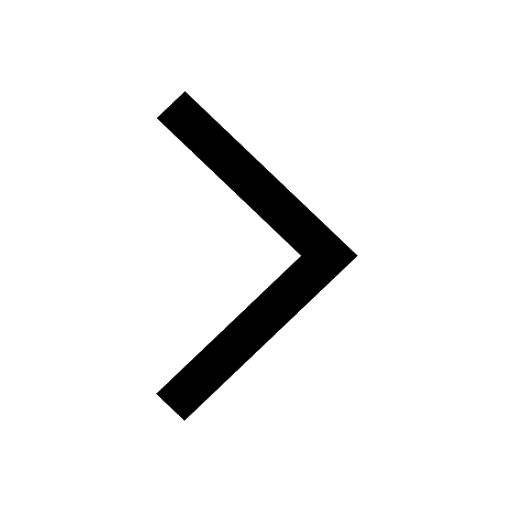
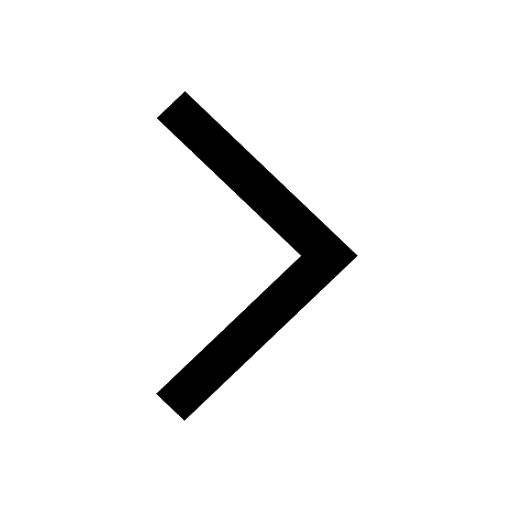
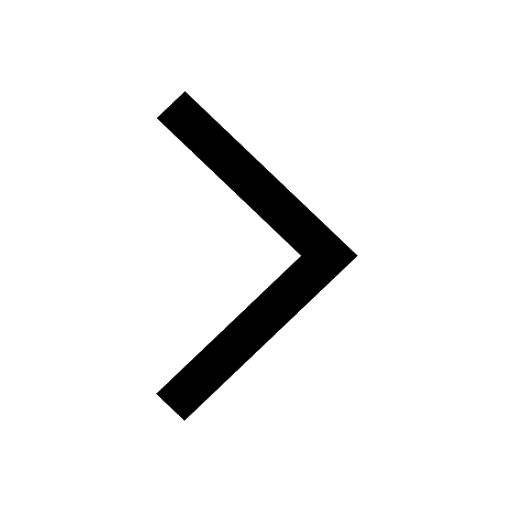
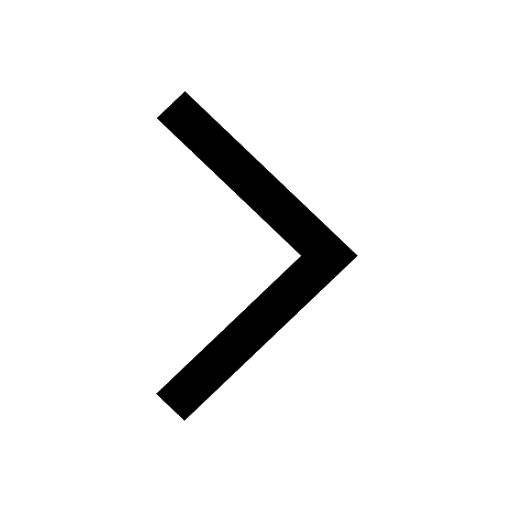
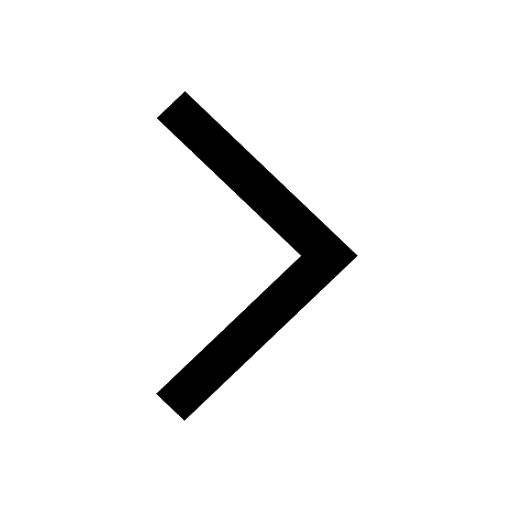
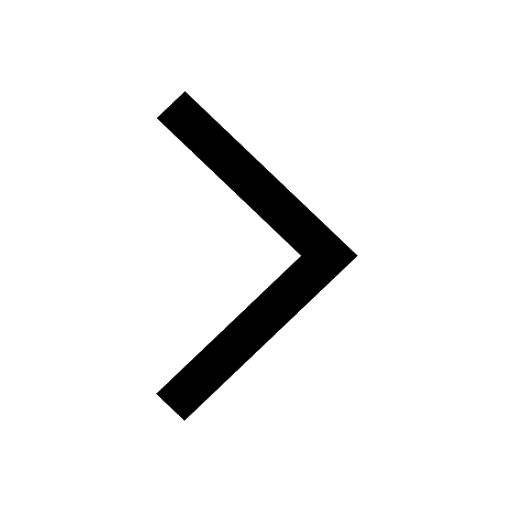
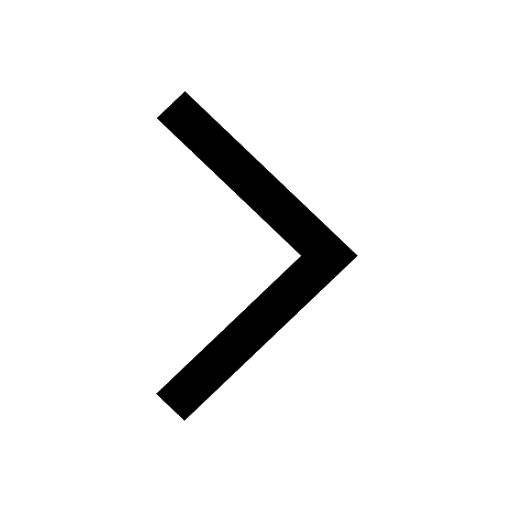
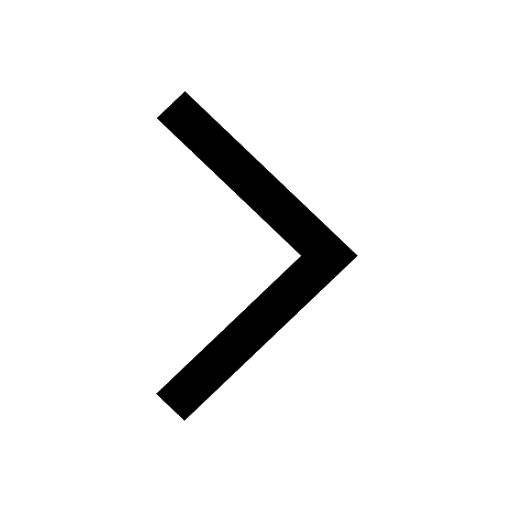
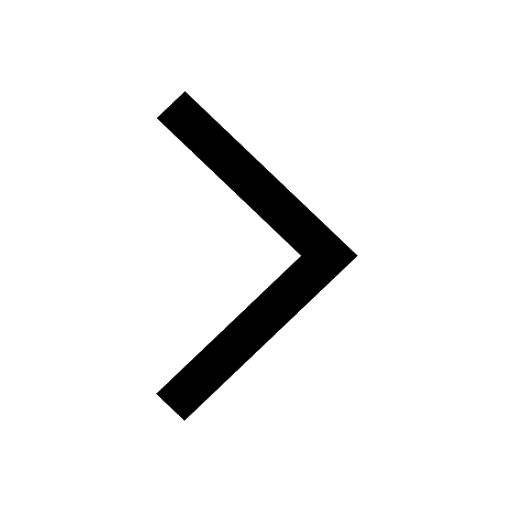
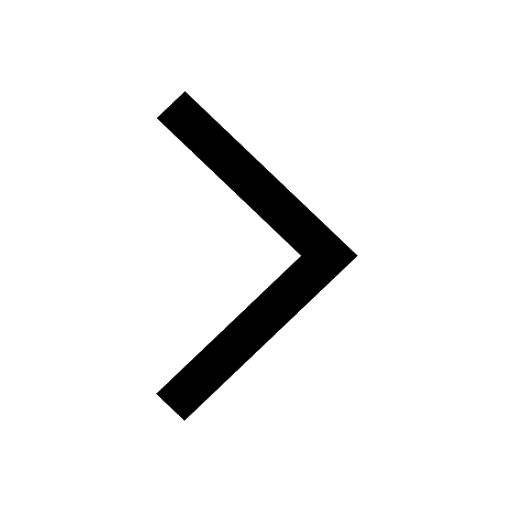
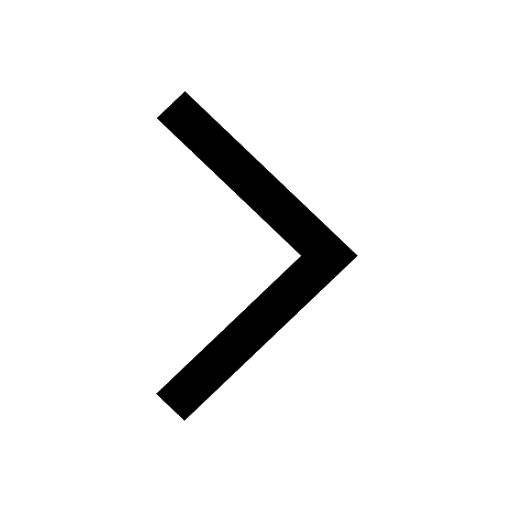
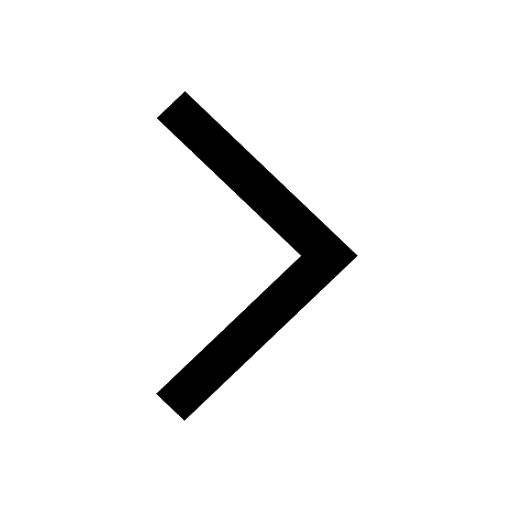