Answer
64.8k+ views
Hint: Here, we have two cases of motion of the satellite. Find the relative velocity of the satellite with respect to earth. The obtain the kinetic energy of the satellite for both cases and take their ratio. Express the terms in terms of the acceleration due to gravity and then we can find our answer.
Complete step by step answer:
Let, the orbital velocity of the satellite in the equatorial plane of earth is ${{v}_{0}}$
Let us consider the case where the satellite is moving in the earth’s rotation direction.
Let, the relative velocity of the satellite in the rest frame of earth is, ${{v}_{1}}$
So, we can write,
${{v}_{1}}={{v}_{0}}-\omega R$
Where $\omega $ is the angular velocity of rotation of earth around its own axis and R is the radius of orbit of the satellite around earth.
Now,
$\omega $ can be written as,
$\omega =\dfrac{2\pi }{t}$
So,
${{v}_{1}}={{v}_{0}}-\dfrac{2\pi R}{T}$
So, the kinetic energy of the satellite will be,
${{T}_{1}}=\dfrac{1}{2}m{{\left( {{v}_{0}}-\dfrac{2\pi R}{T} \right)}^{2}}$
Where, m is the mass of the satellite.
Now, considering the second case where the satellite moves in the opposite direction to the earth’s rotational motion, let, the relative velocity of the satellite is ${{v}_{2}}$
So,
${{v}_{2}}={{v}_{0}}+\dfrac{2\pi R}{T}$
So, the kinetic energy of the satellite will be,
${{T}_{2}}=\dfrac{1}{2}m{{\left( {{v}_{0}}+\dfrac{2\pi R}{T} \right)}^{2}}$
Taking the ratio of the two kinetic energies, we get that,
$\begin{align}
& \dfrac{{{T}_{2}}}{{{T}_{1}}}=\dfrac{\dfrac{1}{2}m{{\left( {{v}_{0}}+\dfrac{2\pi R}{T} \right)}^{2}}}{\dfrac{1}{2}m{{\left( {{v}_{0}}-\dfrac{2\pi R}{T} \right)}^{2}}} \\
& \dfrac{{{T}_{2}}}{{{T}_{1}}}=\dfrac{{{\left( {{v}_{0}}+\dfrac{2\pi R}{T} \right)}^{2}}}{{{\left( {{v}_{0}}-\dfrac{2\pi R}{T} \right)}^{2}}} \\
\end{align}$
Now, the satellite moves around the earth due to gravitational pull of earth and the required centripetal force on the satellite is equal to this gravitational pull.
So, we can write that,
$\dfrac{GMm}{{{R}^{2}}}=\dfrac{m{{v}_{0}}^{2}}{R}$
Where, M is the Earth's mass and G is the gravitational constant.
${{v}_{0}}=\sqrt{\dfrac{GM}{{{R}^{2}}}R}$
Again, acceleration due to gravity can be given by,
$g=\dfrac{GM}{{{R}^{2}}}$
So,
${{v}_{0}}=\sqrt{gR}$
Putting this value on the kinetic energy ratio we get,
$\dfrac{{{T}_{2}}}{{{T}_{1}}}=\dfrac{{{\left( \sqrt{gR}+\dfrac{2\pi R}{T} \right)}^{2}}}{{{\left( \sqrt{gR}-\dfrac{2\pi R}{T} \right)}^{2}}}$
Now, the satellite is moving near the surface of earth. So, R will be almost equal to the radius of earth.
$R=6.4\times {{10}^{6}}m$
$g=10m{{s}^{-2}}$
$T=24h=24\times 60\times 60s$
Putting these values on the above equation, we get that,
$\begin{align}
& \dfrac{{{T}_{2}}}{{{T}_{1}}}=\dfrac{{{\left( \sqrt{10\times 6.4\times {{10}^{6}}}+\dfrac{2\pi \times 6.4\times {{10}^{6}}}{24\times 60\times 60} \right)}^{2}}}{{{\left( \sqrt{10\times 6.4\times {{10}^{6}}}-\dfrac{2\pi \times 6.4\times {{10}^{6}}}{24\times 60\times 60} \right)}^{2}}} \\
& \dfrac{{{T}_{2}}}{{{T}_{1}}}=1.27 \\
& {{T}_{2}}=1.27{{T}_{1}} \\
\end{align}$
The kinetic energy of the satellite in the second case exceeds the first case by 1.27 times.
The correct option is (A).
Note: A satellite moves around the earth due to its gravitational attraction. The gravitational attraction provides the required centripetal force to move the satellite in a circular orbit. Depending on the direction of motion and the height of the satellite the velocity of the satellite will be different and kinetic energy will also be different.
Complete step by step answer:
Let, the orbital velocity of the satellite in the equatorial plane of earth is ${{v}_{0}}$
Let us consider the case where the satellite is moving in the earth’s rotation direction.
Let, the relative velocity of the satellite in the rest frame of earth is, ${{v}_{1}}$
So, we can write,
${{v}_{1}}={{v}_{0}}-\omega R$
Where $\omega $ is the angular velocity of rotation of earth around its own axis and R is the radius of orbit of the satellite around earth.
Now,
$\omega $ can be written as,
$\omega =\dfrac{2\pi }{t}$
So,
${{v}_{1}}={{v}_{0}}-\dfrac{2\pi R}{T}$
So, the kinetic energy of the satellite will be,
${{T}_{1}}=\dfrac{1}{2}m{{\left( {{v}_{0}}-\dfrac{2\pi R}{T} \right)}^{2}}$
Where, m is the mass of the satellite.
Now, considering the second case where the satellite moves in the opposite direction to the earth’s rotational motion, let, the relative velocity of the satellite is ${{v}_{2}}$
So,
${{v}_{2}}={{v}_{0}}+\dfrac{2\pi R}{T}$
So, the kinetic energy of the satellite will be,
${{T}_{2}}=\dfrac{1}{2}m{{\left( {{v}_{0}}+\dfrac{2\pi R}{T} \right)}^{2}}$
Taking the ratio of the two kinetic energies, we get that,
$\begin{align}
& \dfrac{{{T}_{2}}}{{{T}_{1}}}=\dfrac{\dfrac{1}{2}m{{\left( {{v}_{0}}+\dfrac{2\pi R}{T} \right)}^{2}}}{\dfrac{1}{2}m{{\left( {{v}_{0}}-\dfrac{2\pi R}{T} \right)}^{2}}} \\
& \dfrac{{{T}_{2}}}{{{T}_{1}}}=\dfrac{{{\left( {{v}_{0}}+\dfrac{2\pi R}{T} \right)}^{2}}}{{{\left( {{v}_{0}}-\dfrac{2\pi R}{T} \right)}^{2}}} \\
\end{align}$
Now, the satellite moves around the earth due to gravitational pull of earth and the required centripetal force on the satellite is equal to this gravitational pull.
So, we can write that,
$\dfrac{GMm}{{{R}^{2}}}=\dfrac{m{{v}_{0}}^{2}}{R}$
Where, M is the Earth's mass and G is the gravitational constant.
${{v}_{0}}=\sqrt{\dfrac{GM}{{{R}^{2}}}R}$
Again, acceleration due to gravity can be given by,
$g=\dfrac{GM}{{{R}^{2}}}$
So,
${{v}_{0}}=\sqrt{gR}$
Putting this value on the kinetic energy ratio we get,
$\dfrac{{{T}_{2}}}{{{T}_{1}}}=\dfrac{{{\left( \sqrt{gR}+\dfrac{2\pi R}{T} \right)}^{2}}}{{{\left( \sqrt{gR}-\dfrac{2\pi R}{T} \right)}^{2}}}$
Now, the satellite is moving near the surface of earth. So, R will be almost equal to the radius of earth.
$R=6.4\times {{10}^{6}}m$
$g=10m{{s}^{-2}}$
$T=24h=24\times 60\times 60s$
Putting these values on the above equation, we get that,
$\begin{align}
& \dfrac{{{T}_{2}}}{{{T}_{1}}}=\dfrac{{{\left( \sqrt{10\times 6.4\times {{10}^{6}}}+\dfrac{2\pi \times 6.4\times {{10}^{6}}}{24\times 60\times 60} \right)}^{2}}}{{{\left( \sqrt{10\times 6.4\times {{10}^{6}}}-\dfrac{2\pi \times 6.4\times {{10}^{6}}}{24\times 60\times 60} \right)}^{2}}} \\
& \dfrac{{{T}_{2}}}{{{T}_{1}}}=1.27 \\
& {{T}_{2}}=1.27{{T}_{1}} \\
\end{align}$
The kinetic energy of the satellite in the second case exceeds the first case by 1.27 times.
The correct option is (A).
Note: A satellite moves around the earth due to its gravitational attraction. The gravitational attraction provides the required centripetal force to move the satellite in a circular orbit. Depending on the direction of motion and the height of the satellite the velocity of the satellite will be different and kinetic energy will also be different.
Recently Updated Pages
Write a composition in approximately 450 500 words class 10 english JEE_Main
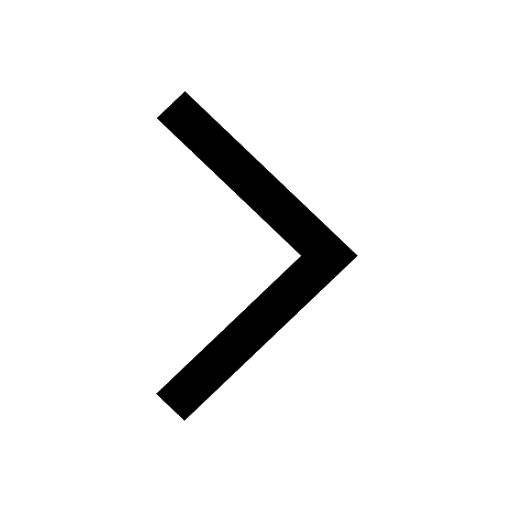
Arrange the sentences P Q R between S1 and S5 such class 10 english JEE_Main
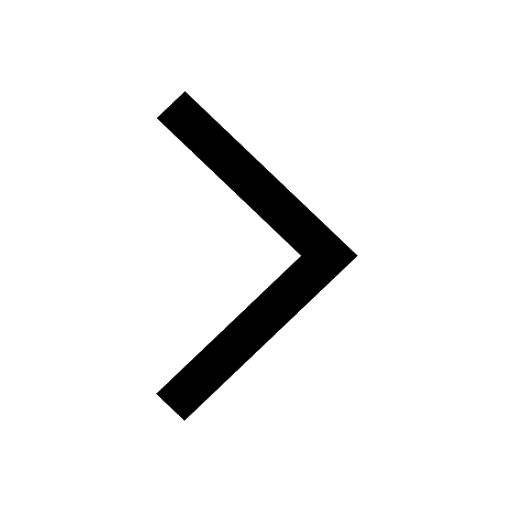
What is the common property of the oxides CONO and class 10 chemistry JEE_Main
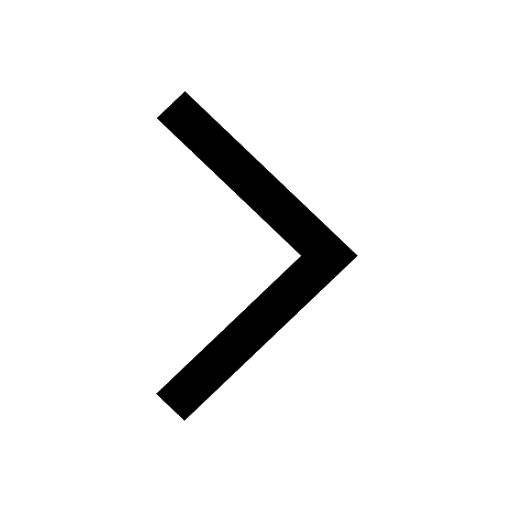
What happens when dilute hydrochloric acid is added class 10 chemistry JEE_Main
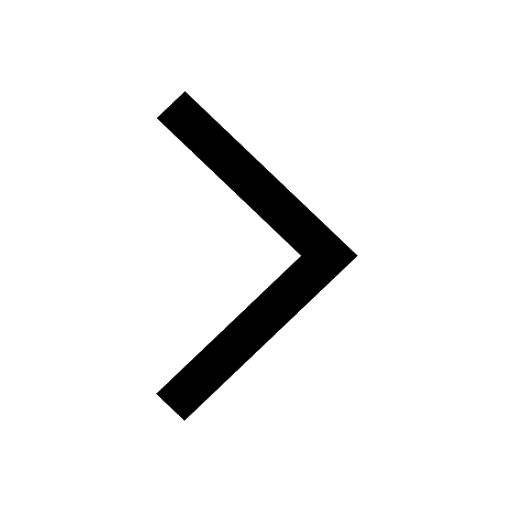
If four points A63B 35C4 2 and Dx3x are given in such class 10 maths JEE_Main
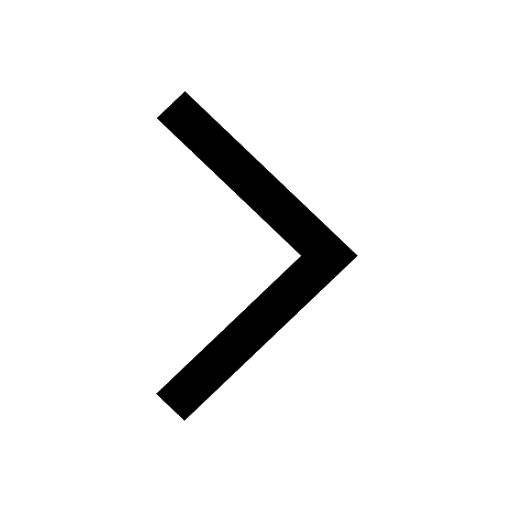
The area of square inscribed in a circle of diameter class 10 maths JEE_Main
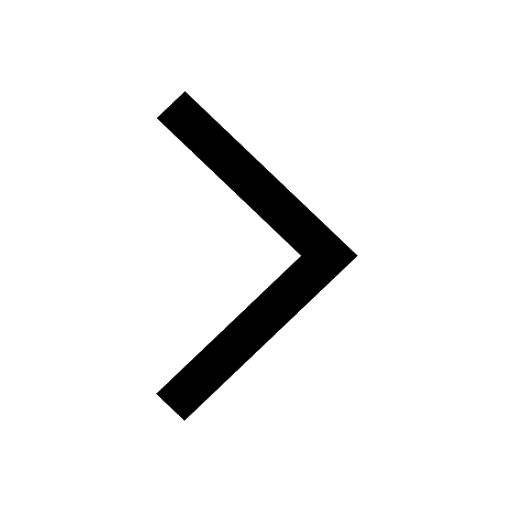
Other Pages
Excluding stoppages the speed of a bus is 54 kmph and class 11 maths JEE_Main
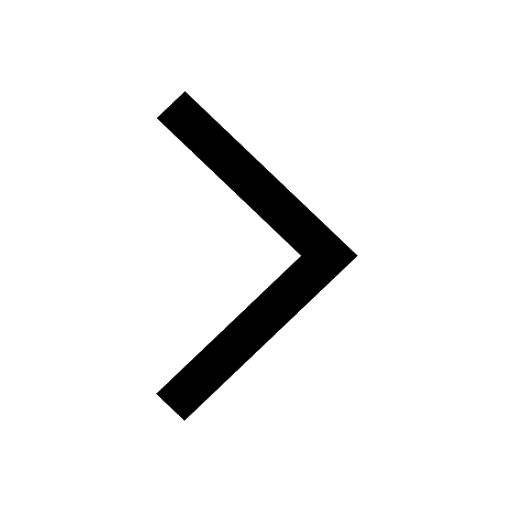
In the ground state an element has 13 electrons in class 11 chemistry JEE_Main
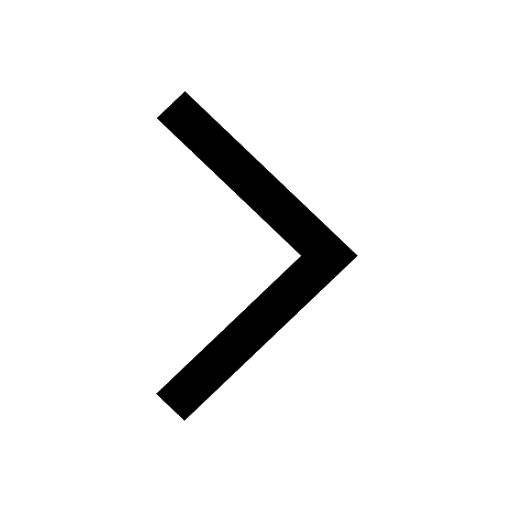
Electric field due to uniformly charged sphere class 12 physics JEE_Main
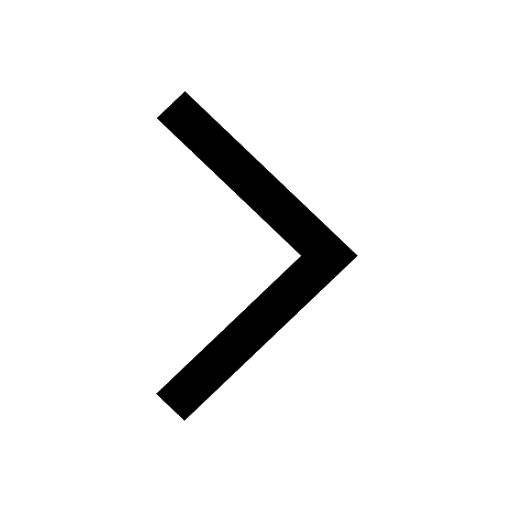
A boat takes 2 hours to go 8 km and come back to a class 11 physics JEE_Main
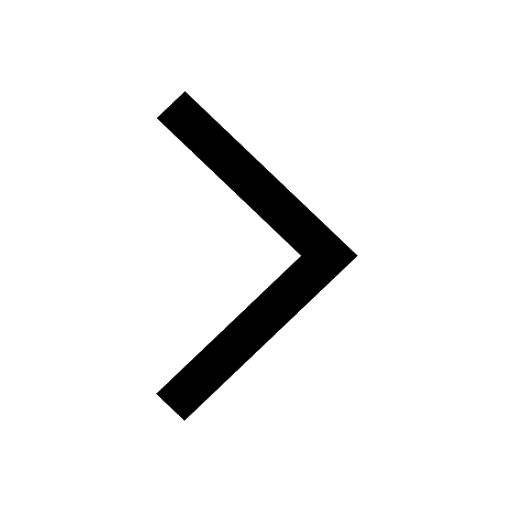
According to classical free electron theory A There class 11 physics JEE_Main
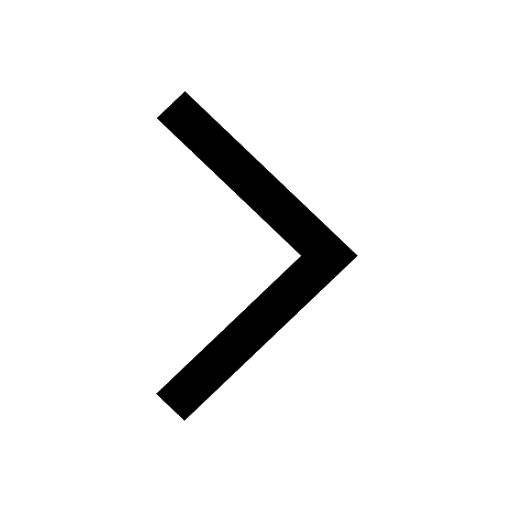
Differentiate between homogeneous and heterogeneous class 12 chemistry JEE_Main
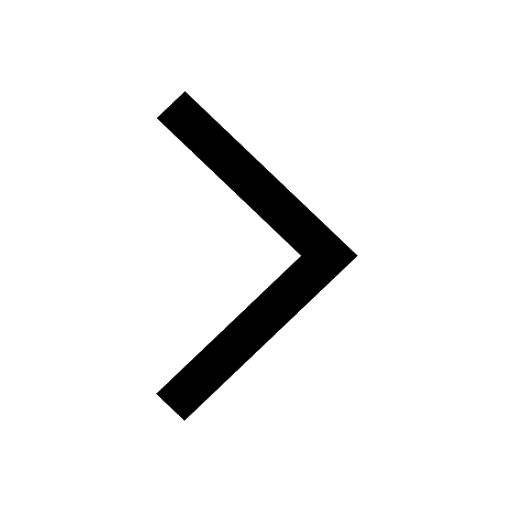