Answer
64.8k+ views
Hint: To solve this question, we need to find out the displacement current because of the changing potential difference between the plates of the capacitor. Then using the formula for the magnetic field due to an infinitely long current carrying wire, we can get the final answer.
Formula used: The formula used to solve this question is given by
$C = \dfrac{{{\varepsilon _0}A}}{d}$, here $C$ is the capacitance of a parallel plate capacitor having plates of area $A$ separated by a distance of $d$.
Complete step-by-step solution:
We know that the capacitance of a parallel plate capacitor is given by
$C = \dfrac{{{\varepsilon _0}A}}{d}$............(1)
We know that the area of a circular plate is given by
$A = \pi {r^2}$
Putting this in (1) we get
$C = \dfrac{{{\varepsilon _0}\pi {r^2}}}{d}$
$ \Rightarrow d = \dfrac{{{\varepsilon _0}\pi {r^2}}}{C}$ ………...(2)
Now, we know that
$E = \dfrac{V}{d}$
Differentiating both sides with respect to time t, we have
$\dfrac{{dE}}{{dt}} = \dfrac{1}{d} \times \dfrac{{dV}}{{dt}}$
Substituting (2) in the above equation we get
$\dfrac{{dE}}{{dt}} = \dfrac{C}{{{\varepsilon _0}\pi {r^2}}}\dfrac{{dV}}{{dt}}$...............(3)
Now, we know that the displacement current is given by
${i_d} = {\varepsilon _0}\dfrac{{d{\varphi _E}}}{{dt}}$...............(4)
The electric flux is given by
${\varphi _E} = EA$
$ \Rightarrow {\varphi _E} = \pi {r^2}E$
Putting this in (4) we have
${i_d} = {\varepsilon _0}\dfrac{{d\left( {\pi {r^2}E} \right)}}{{dt}}$
$ \Rightarrow {i_d} = {\varepsilon _0}\pi {r^2}\dfrac{{dE}}{{dt}}$........(5)
Now, the magnetic field at the edge of the plate can be given by
$B = \dfrac{{{\mu _0}{i_d}}}{{2\pi r}}$
Putting (5) in the above expression we get
$B = \dfrac{{{\mu _0}{\varepsilon _0}\pi {r^2}}}{{2\pi r}}\dfrac{{dE}}{{dt}}$
$ \Rightarrow B = \dfrac{{{\mu _0}{\varepsilon _0}r}}{2}\dfrac{{dE}}{{dt}}$
Putting (3) in the above expression we get
$B = \dfrac{{{\mu _0}{\varepsilon _0}r}}{2}\dfrac{C}{{{\varepsilon _0}\pi {r^2}}}\dfrac{{dV}}{{dt}}$
$ \Rightarrow B = \dfrac{{{\mu _0}C}}{{2r}}\dfrac{{dV}}{{dt}}$.............(6)
Now, according to the question, we have the radius of the plate equal to $10cm$. So we have
$r = 10cm$
$ \Rightarrow r = 0.1m$ …………..(7)
Also, the capacity of the capacitor is given to be equal to \[2\mu F\]. So we have
$C = 2\mu F$
$ \Rightarrow C = 2 \times {10^{ - 6}}F$..........(8)
Also the variation of voltage with time is given to be equal to $6 \times {10^2}{\text{V}}{{\text{s}}^{ - 1}}$. So we have
$\dfrac{{dV}}{{dt}} = 6 \times {10^2}{\text{V}}{{\text{s}}^{ - 1}}$............(9)
Substituting (7), (8), and (9) in (7), we get the final value of the magnetic field as
$B = 3.33 \times {10^{ - 9}}T$
Hence, the correct answer is option C.
Note: The magnetic field obtained from the displacement current is similar to that of the magnetic field produced by an infinitely long current carrying wire. The displacement current passes through the centre of the plates of the capacitor, and hence we obtained the magnetic field at the edge of the plate.
Formula used: The formula used to solve this question is given by
$C = \dfrac{{{\varepsilon _0}A}}{d}$, here $C$ is the capacitance of a parallel plate capacitor having plates of area $A$ separated by a distance of $d$.
Complete step-by-step solution:
We know that the capacitance of a parallel plate capacitor is given by
$C = \dfrac{{{\varepsilon _0}A}}{d}$............(1)
We know that the area of a circular plate is given by
$A = \pi {r^2}$
Putting this in (1) we get
$C = \dfrac{{{\varepsilon _0}\pi {r^2}}}{d}$
$ \Rightarrow d = \dfrac{{{\varepsilon _0}\pi {r^2}}}{C}$ ………...(2)
Now, we know that
$E = \dfrac{V}{d}$
Differentiating both sides with respect to time t, we have
$\dfrac{{dE}}{{dt}} = \dfrac{1}{d} \times \dfrac{{dV}}{{dt}}$
Substituting (2) in the above equation we get
$\dfrac{{dE}}{{dt}} = \dfrac{C}{{{\varepsilon _0}\pi {r^2}}}\dfrac{{dV}}{{dt}}$...............(3)
Now, we know that the displacement current is given by
${i_d} = {\varepsilon _0}\dfrac{{d{\varphi _E}}}{{dt}}$...............(4)
The electric flux is given by
${\varphi _E} = EA$
$ \Rightarrow {\varphi _E} = \pi {r^2}E$
Putting this in (4) we have
${i_d} = {\varepsilon _0}\dfrac{{d\left( {\pi {r^2}E} \right)}}{{dt}}$
$ \Rightarrow {i_d} = {\varepsilon _0}\pi {r^2}\dfrac{{dE}}{{dt}}$........(5)
Now, the magnetic field at the edge of the plate can be given by
$B = \dfrac{{{\mu _0}{i_d}}}{{2\pi r}}$
Putting (5) in the above expression we get
$B = \dfrac{{{\mu _0}{\varepsilon _0}\pi {r^2}}}{{2\pi r}}\dfrac{{dE}}{{dt}}$
$ \Rightarrow B = \dfrac{{{\mu _0}{\varepsilon _0}r}}{2}\dfrac{{dE}}{{dt}}$
Putting (3) in the above expression we get
$B = \dfrac{{{\mu _0}{\varepsilon _0}r}}{2}\dfrac{C}{{{\varepsilon _0}\pi {r^2}}}\dfrac{{dV}}{{dt}}$
$ \Rightarrow B = \dfrac{{{\mu _0}C}}{{2r}}\dfrac{{dV}}{{dt}}$.............(6)
Now, according to the question, we have the radius of the plate equal to $10cm$. So we have
$r = 10cm$
$ \Rightarrow r = 0.1m$ …………..(7)
Also, the capacity of the capacitor is given to be equal to \[2\mu F\]. So we have
$C = 2\mu F$
$ \Rightarrow C = 2 \times {10^{ - 6}}F$..........(8)
Also the variation of voltage with time is given to be equal to $6 \times {10^2}{\text{V}}{{\text{s}}^{ - 1}}$. So we have
$\dfrac{{dV}}{{dt}} = 6 \times {10^2}{\text{V}}{{\text{s}}^{ - 1}}$............(9)
Substituting (7), (8), and (9) in (7), we get the final value of the magnetic field as
$B = 3.33 \times {10^{ - 9}}T$
Hence, the correct answer is option C.
Note: The magnetic field obtained from the displacement current is similar to that of the magnetic field produced by an infinitely long current carrying wire. The displacement current passes through the centre of the plates of the capacitor, and hence we obtained the magnetic field at the edge of the plate.
Recently Updated Pages
Write a composition in approximately 450 500 words class 10 english JEE_Main
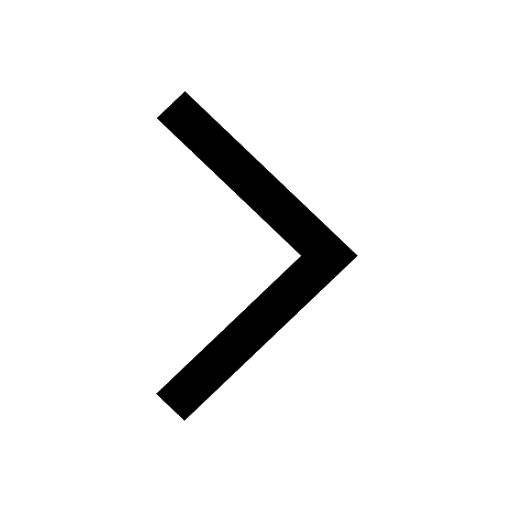
Arrange the sentences P Q R between S1 and S5 such class 10 english JEE_Main
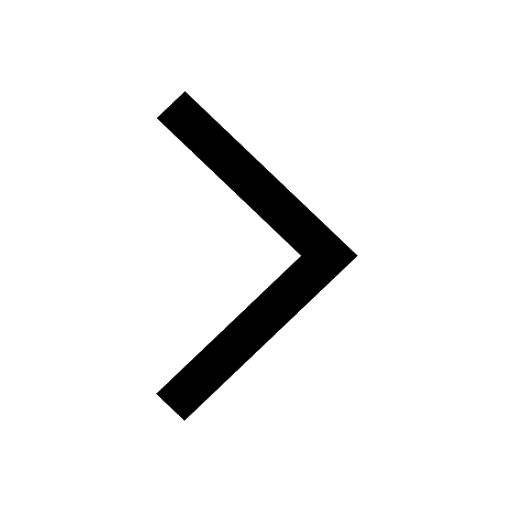
What is the common property of the oxides CONO and class 10 chemistry JEE_Main
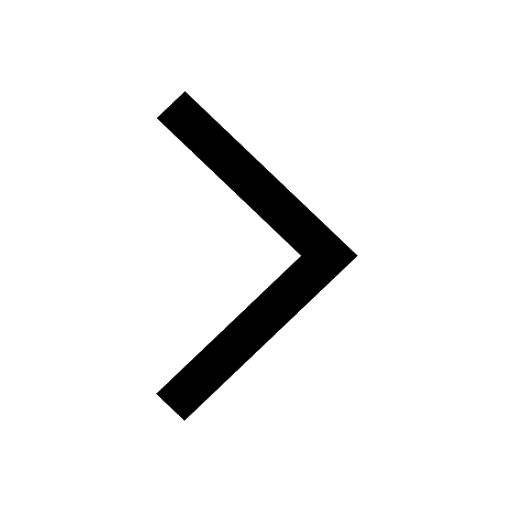
What happens when dilute hydrochloric acid is added class 10 chemistry JEE_Main
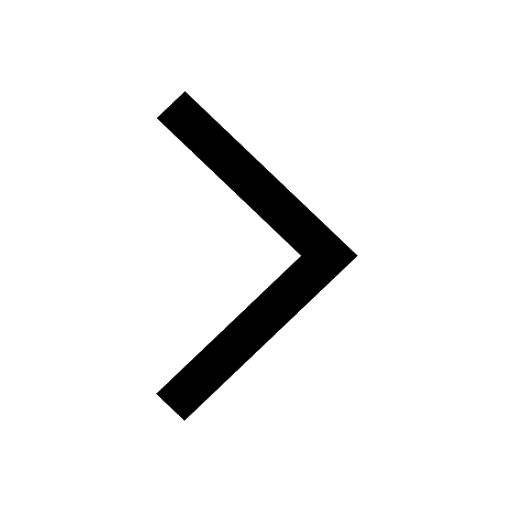
If four points A63B 35C4 2 and Dx3x are given in such class 10 maths JEE_Main
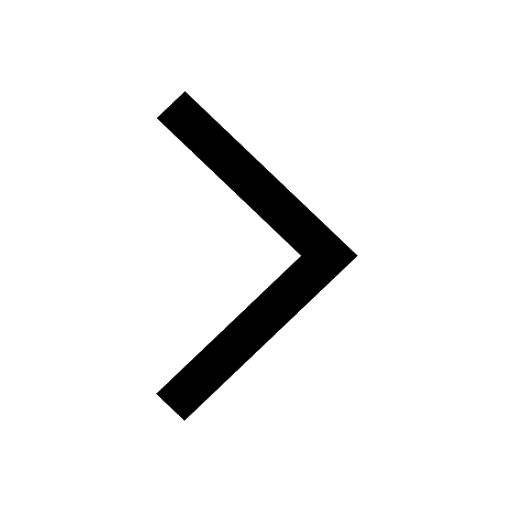
The area of square inscribed in a circle of diameter class 10 maths JEE_Main
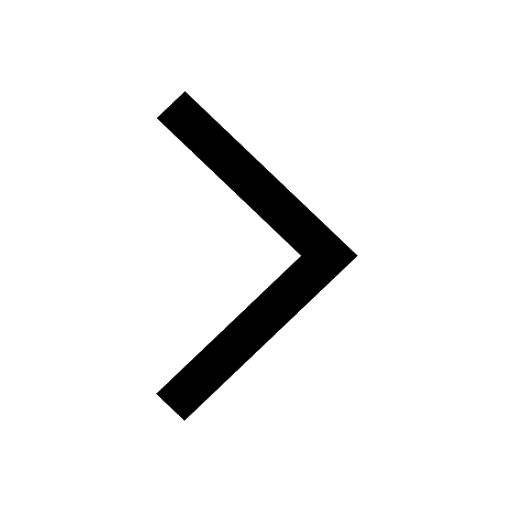
Other Pages
A boat takes 2 hours to go 8 km and come back to a class 11 physics JEE_Main
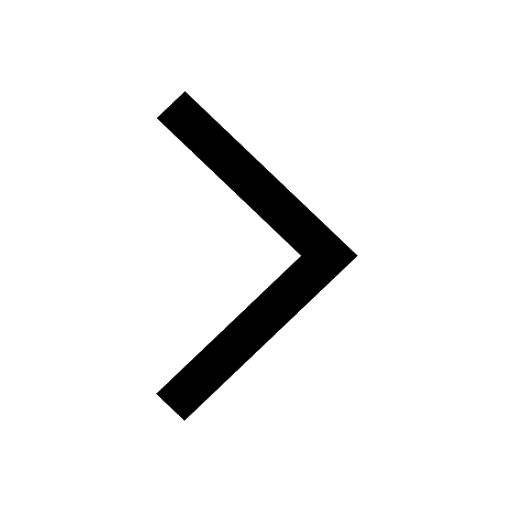
Electric field due to uniformly charged sphere class 12 physics JEE_Main
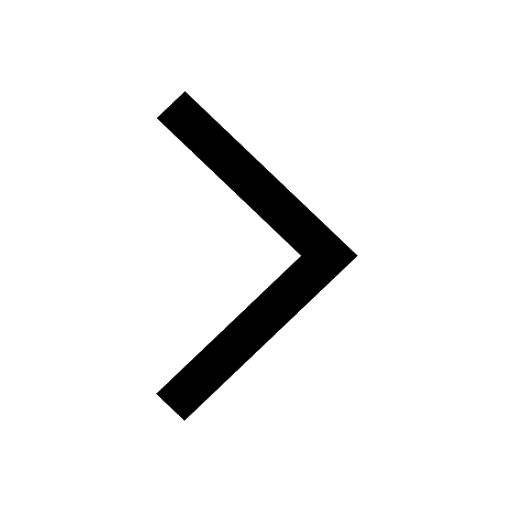
In the ground state an element has 13 electrons in class 11 chemistry JEE_Main
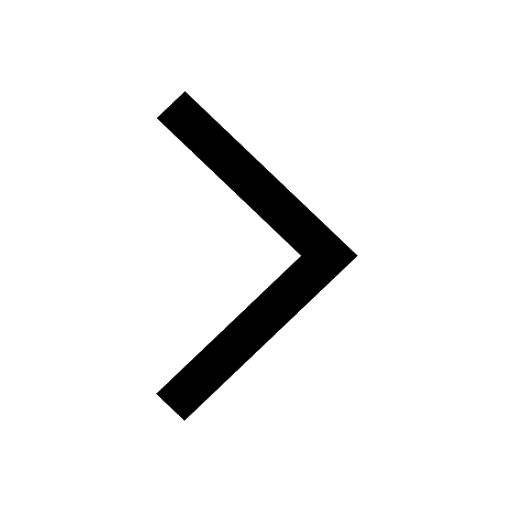
According to classical free electron theory A There class 11 physics JEE_Main
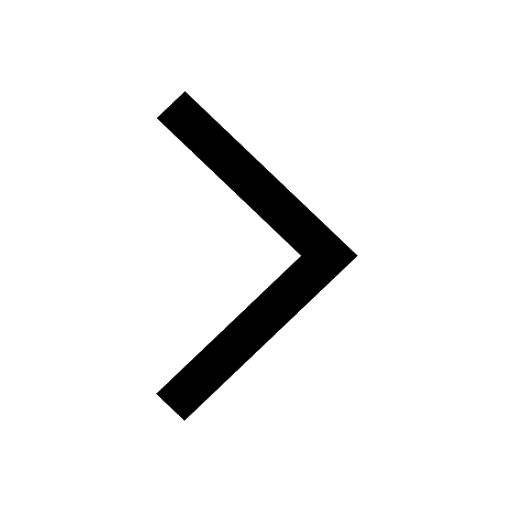
Differentiate between homogeneous and heterogeneous class 12 chemistry JEE_Main
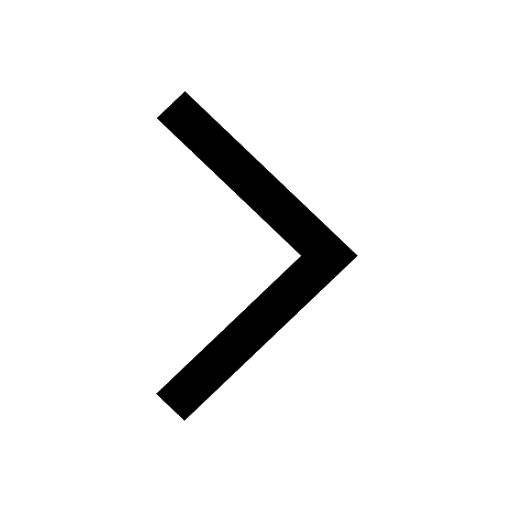
Excluding stoppages the speed of a bus is 54 kmph and class 11 maths JEE_Main
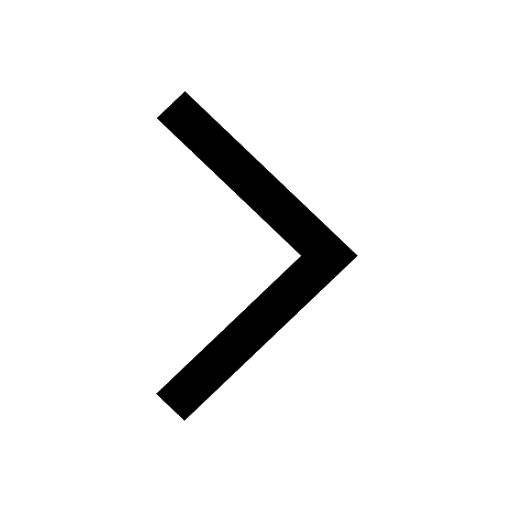