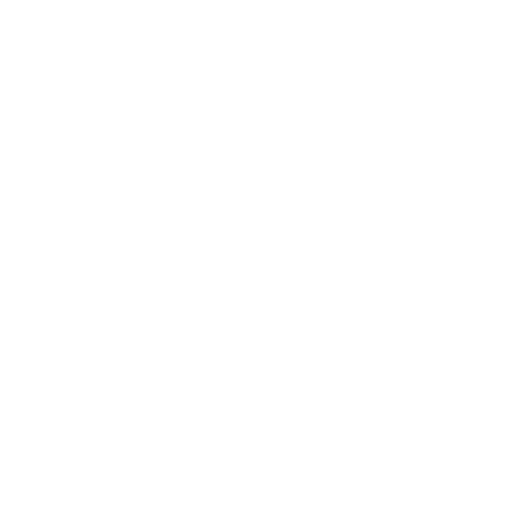

Photochemical Equivalence Law Explanation on Vedantu
The photochemical equivalence law is a basic concept relating to light-induced chemical reactions, which states that for every unit of radiation absorbed, a molecule of the substance reacts. A quantum is defined as a unit of electromagnetic radiation with an energy equal to the product of a constant (or Planck's constant - h) and the frequency of the radiation, which is symbolized by the Greek letter nu (ν).
Discovery and Expressing the Photochemical Equivalence Law
In Chemistry, quantitative measures of the substances can be expressed in terms of gram moles, one single gram mole consisting of 6.022140857 × 1023 (which is an Avogadro's number) molecules. As a result, the photochemical equivalence law can be restated as follows: 6.022140857 × 1023 quanta of light are absorbed for every mole of the material that reacts.
The photochemical equivalence law will be applied to the part of a light-induced reaction, which is known to be the primary process; it means the initial chemical change, which results directly from the light absorption. In most photochemical reactions, usually, the primary process can be followed by so-called secondary processes, which are normal interactions between the reactants that do not require the absorption of light.
Such reactions resultantly do not appear to obey the one quantum - one single molecule reactant relationship. This law is further restricted to the conventional photochemical processes using the light sources with moderate intensities; high-intensity light sources like the ones used in laser experiments and flash photolysis are referred to cause the so-called bi-photonic processes; it means the absorption by a molecule of a substance of the light two photons.
Also, the photochemical equivalence law was at times referred to as the Stark–Einstein law after the German-born Physicists Albert Einstein and Johannes Stark, who independently formulated the law between the years 1908 and 1913.
Photochemical Reactions
A photochemical reaction is defined as a chemical reaction, which results when the reacting substance has been exposed to radiation. The activation of the reacting molecule can be achieved by the absorption of photons of the appropriate energy. Often, a radiation photon, which is known as quantum, is given as the primary unit of radiation of energy hv, where v is the frequency of radiation.
These particular reactions are affected by the absorption of radiation of visible and ultraviolet regions of wavelength. As per Einstein's law of Photochemical equivalence, "Each quantum of the radiation absorbed activates one molecule in the major step of a photochemical process."
The reason behind rate photochemical reactions are independent of the concentration of product and reactant is given by this law. This law also states that every molecule takes one quantum of radiation for its activation, including the subsequent chemical reaction. So, the rate of Photochemical reaction increases with increasing intensity of light and can be independent of the concentration of the product and reactant.
Einstein Law of Photochemical Equivalence
Einstein's Law of Photochemical Equivalence is a fundamental law of photochemistry, which establishes that every photon absorbed causes a single elementary reaction. The reaction can consist of the chemical transformation of the substance's molecules or in their physical excitation and the emission of the energy that is absorbed (or in the thermal energy transformation). The number N of the reacted molecules can be related to the energy E, which is absorbed by the system by the equation as:
E = Nhv = Nh \[\frac{c}{λ}\]
where 'v' is given as the frequency of radiation, 'c' is given as the velocity of light, 'λ' is given as the wavelength of the light, and 'h' is given as Planck's constant. The criterion for the applicability of Einstein's law of photochemical equivalence is typically the quantity " (the photochemical reaction's quantum yield), which is equal to the ratio of the number of reacted molecules of a given material to many light quanta absorbed. As per the law, 'γ' should be equal to unity.
From the Einstein law of photochemical equivalence, the deviations that are frequently observed in nature are explained by secondary processes usually. This law was discovered by Albert Einstein in 1912. Einstein's photochemical equivalence law is applicable to that segment of light-induced reaction which is known as the primary process; that is, the preliminary chemical amends that lead to the outcome directly from the incorporation of light. In most photochemical reactions, the above-mentioned primary process is more often than not followed by the allegedly known secondary processes that are standard interactions amid reactants that do not need to absorb light.
As a result, any of such reactions appear to not be in abeyance of the commonly known one quantum–one molecule reactant relationship. The law additionally has limitations involving the conventional photochemical process which involves the usage of light sources using reasonable intensities; high-intensity light sources on the other hand such as those that are being used in flash photolysis along with laser experiments are said to be causing the so-called biphotonic processes; i.e., the process of absorption happening throughout a molecule of a substance for two photons of light.
Photoisomerization of Azobenzene
Photoisomerization is a molecular behavior in chemistry, where photoexcitation causes the structural transition between the isomers. Both the reversible and irreversible photoisomerization reactions do exist. However, usually, the term "photoisomerization" indicates a reversible process. For rewritable DVDs, CDs, and 3D optical data storage, photoisomerizable molecules are already used in pigments. In addition, photoisomerizable molecules have recently sparked interest in molecular devices such as molecular motors, switches, and electronics.
The below figure shows the photoisomerization of azobenzene:
(Image will be uploaded soon)
Photoisomerization behavior may be roughly categorized into many classes. Two major classes of it are trans-cis (or 'E-'Z) conversion and the open-closed ring transition. Examples of the former include azobenzene and stilbene. This type of compound contains a double bond, and inversion or rotation around the double bond affords isomerization between the two states. Examples of the latter are given as diarylethene and fulgide. This compound type undergoes bond cleavage and bond creation upon irradiation with specific wavelengths of light. Still, the other class is given as the Di-pi-methane rearrangement.
Discuss the Structure of Azobenzene in Brief
The structure of the Azobenzene molecule comprises two phenyl rings that are linked to one another through an N=N double bond. This kind of bond is also known as the parent or the main compound as known in the azobenzene class of compounds.
Manipulation of Azobenzene Photoisomerization by way of Strong Light– also known as Molecule Coupling
The process of formation of hybrid light–molecule states that are known as polaritons provides a fresh plan and strategy to control the photochemistry inside the molecules. To exploit its potential to the complete limits, one has to put together a toolbox of polaritonic phenomenologies that add on the ones of standard photochemistry.
By using a method of a state-of-the-art computational photochemistry strategy accompanied by a strong molecule coupling system, here we also make known a variety of mechanisms not relating to polaritonic chemistry: coherent population oscillations amid polaritons, the process of quenching by capturing the dead-end polaritonic states, and the adjustment of the photochemical response pathway alongside quantum yields.
We will currently have our focus on azobenzene photoisomerization, which holds in itself the necessary characteristics of complex photochemical reactions like we can say the occurrence of conical intersections and reaction coordinates involving multiple internal modes. A chemically comprehensive simulation offers a structure to rationalize how the process of strong molecule coupling has its effects on the photochemistry of real molecules.
Why is the Process of Photoisomerization Considered Important?
The process of Photoisomerization helps in modifying the polarizability inside a molecule that shows the end results as changes in the linear optical properties (i.e., indices of refraction alongside absorption can be seen easily), and also to alterations occurring in the nonlinear optical properties in the second and third-order given that the isomer stops any such characteristics.
The Main Purpose of Irradiating the Azobenzene
The trans-azobenzene with no trouble isomerizes to the cis isomer by the process of irradiation of the trans isomer that has a wavelength in the range of 320–350 nm. The reaction then produced is reversible and apart from that the trans isomer can be brought back only when the cis isomer gets irradiated with light having wavelengths of 400–450 nm, or if it is heated up.
FAQs on Photochemical Equivalence Law
1. What is Photoionization?
Photoionization is given as the physical process, where an incident photon ejects either one or more electrons from an atom, molecule, or ion. This is important, a similar process that takes place with the photoelectric effect with metals. In the case of a single atom or gas, the term photoionization is very common.
2. State Einstein Law of Photochemical Equivalence?
Consider a system, which is in free fall; only the gravitational field is said to be acting. Inside it, locally, we can neutralize all the gravitation effects. It is used as an inertial system, where all the laws of the special theory of relativity contain.
The laws of gravitation may only be neutralized locally due to the uniformly accelerating frame not equivalent to the frame in a non-homogeneous gravitational field. Therefore, locally, we can gain an inertial system in a region where the variation of the gravitational field is very small. In such an inertial system, masses remain at either rest or move in straight lines with uniform velocity.
3. What is the Equivalence Principle?
The Equivalence Principle was the formalization, in which Inertial Mass and Gravitational Mass were equal by Einstein. It is not quite sure why anyone considers them to be different, but actually, still, the Establishment view is that Gravity and Inertia are separate forces. But this produces a Paradox, which none seems to want to discuss.
4. Give the Example of Equivalence Relation.
A relation that is Symmetric, Reflexive, and Transitive is called an Equivalence relation. For example, suppose a relation R - "x is parallel to y." Here R is given as an Equivalence relation. Whereas, for reflexive, each line is parallel to itself, thus Reflexive.
5. How is the verification done of the photochemical equivalence law of Einstein?
The decisive factor needed for the Einstein's law of photochemical equivalence to be applicable is usually the number " (the photochemical reaction's quantum yield), which is one and the same to the relative amount of the amount of reacted molecules of the material being affected to countless light quanta absorbed.
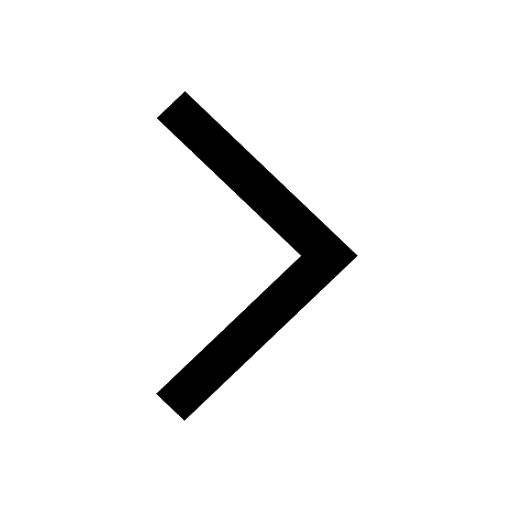
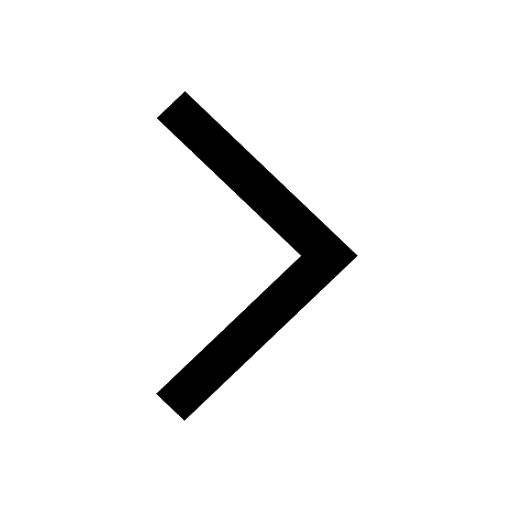
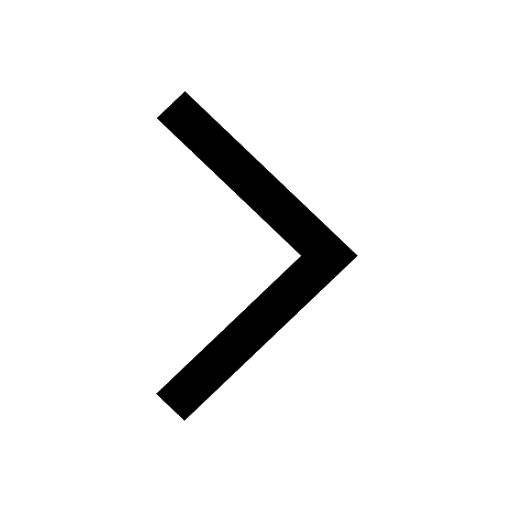
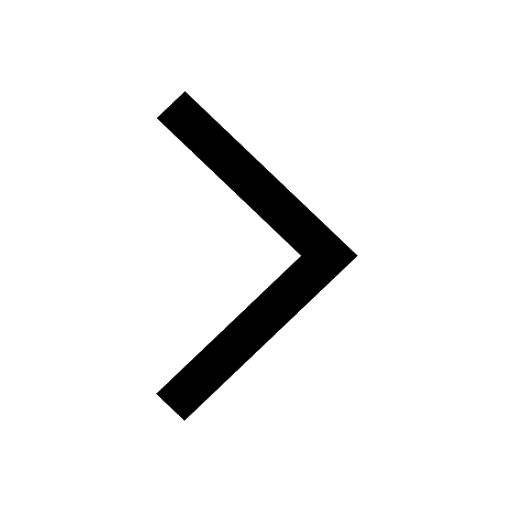
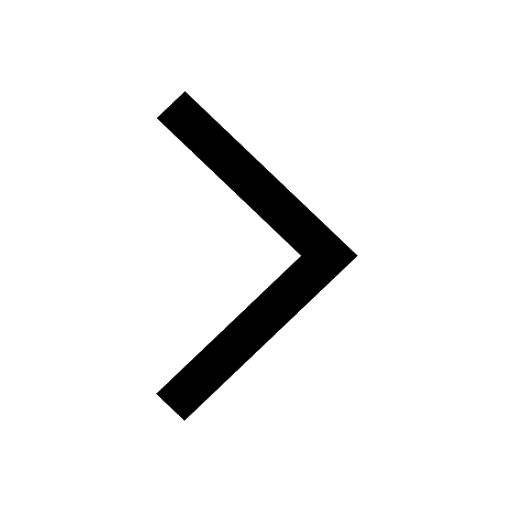
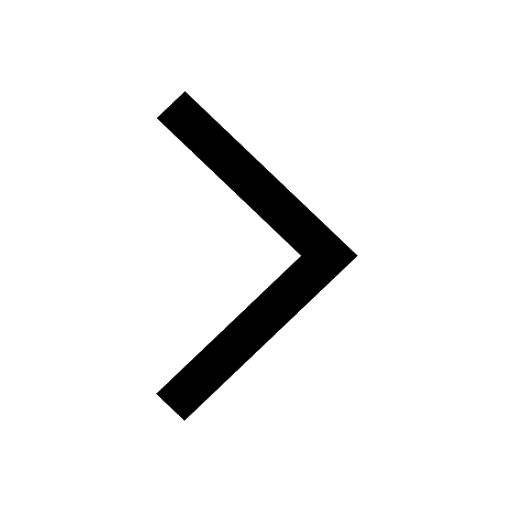