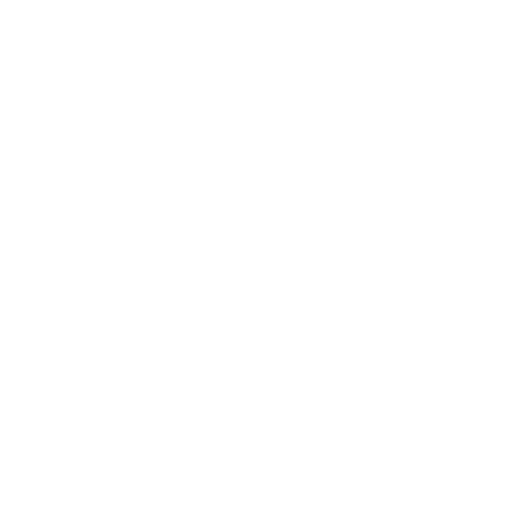

What is the Law of Mass Action?
The law of Mass action asserts that at a constant temperature, the product of the number of electrons in the conduction band and the number of holes in the valence band remains constant, regardless of the quantity of donor and acceptor impurities added.
It is expressed Mathematically as
np = ni2 = constant
Where ni is the intrinsic carrier concentration
n is number of electrons in the conduction band
p is number of holes in the valence band
The rate of each chemical reaction is proportional to the product of the Masses of the reacting substances, each Mass raised to a power equal to the coefficient that occurs in the chemical equation, according to the law of Mass action. The Norwegian scientists Cato M. Guldberg and Peter Waage created this law between 1864 and 1879, although it is now primarily of historical relevance. Although this law was beneficial for determining the correct Equilibrium equation for a reaction, the rate expressions it provided are now known to only apply to elementary reactions.
The Law of Mass Action in Practice
As this law also applies to semiconductors, it has a number of major consequences in the domains of electronics and semiconductor physics. When the semiconductor system is in thermal Equilibrium, the law of Mass action gives a link between the concentrations of electron holes and free electrons.
The law of Mass action is also useful in the following areas:
Ecological Mathematics
Socio physics is a branch of physics that deals with (social physics)
Mathematical Expression of the Law of Mass Action
Consider a hypothetical reaction:
A + B → Products
As per the Law of Mass Action definition, the rate of reaction ‘R’ is given as:
R ∝ AB
For a general reaction denoted by aA + bB → Products, rate of the reaction according to the Law of Mass Action is indicated as:
R ∝ Aa Bb
As a result, Rf = Kf Aa Bb, where Kf is the rate constant of the forward reaction.
In the same way, the rate of backward reaction will be:
Rb ∝ Cc Dd
Now, in order for a reaction to being chemically balanced,
Rf = Rb
In the Law of Mass Action Equation, what is the Equilibrium Constant?
The Equilibrium constant, Kc, is defined as the ratio of the product of the Equilibrium concentrations of the products to the product of the Equilibrium concentration of the reactants in a balanced chemical equation, with each concentration term raised to the individual stoichiometric coefficients of the reactants and products.
The Law of Mass Action in a Gaseous System explained
The concentration terms are replaced with partial pressures in gaseous systems. We assume that the partial pressure of a gas is precisely proportional to its concentration at a given temperature to explain the Law of Mass Action for such systems.
The Equilibrium constant Kp in terms of partial pressures for the hypothetical reversible equation aA(g) + bB(g) cC(g) + dD(g) will be:
\[K_p = \frac{\begin{bmatrix} P_C \end{bmatrix}^c \begin{bmatrix}P_D\end{bmatrix}^d }{\begin{bmatrix}P_A\end{bmatrix}^a \begin{bmatrix}P_B \end{bmatrix}^b }\]
Ostwald's Dilution Law is used to determine the dissociation Equilibrium of weak electrolytes using the Law of Mass Action.
Semiconductor physics is a branch of physics that deals with semiconductors.
For the purpose of explaining condensed matter diffusion
In Mathematical epidemiology, to solve the disease transmission model.
Example-
Consider the response. N2O4 2NO2
The Equilibrium constant for this reaction can be calculated using the Law of Mass Action formula:
\[\frac{\begin{bmatrix}N_2O_4\end{bmatrix}}{\begin{bmatrix}NO_2\end{bmatrix}_2} = Kc \]
Using [N2O4] = 0.0417 mol L-1 and [NO2] = 0.0165 mol L-1 as starting points,
\[153 = Kc = \frac{0.0417}{0.01652}\]
The Law of Mass Action explains the relationship between the velocity of a chemical reaction and the molar concentration of the reactants at a particular temperature. Put forward by Norwegian scientists, Peter Wage and Cato Gulberg in 1864, the Law of Mass Action in Chemistry underpins many different types of physiological, biochemical, and pharmacological phenomena.
Now, let’s define the Law of Mass Action:
The law states that the rate of a chemical reaction at a given temperature and instant is directly proportional to the product of the reactants’ active Masses. Here, active Mass means the molar concentration of a substance per unit volume of it. The unit of active Mass is mol dm-3, and its value is expressed within square brackets.
Mathematical Expression of the Law of Mass Action
Consider a hypothetical reaction:
A + B → Products
As per the Law of Mass Action definition, the rate of reaction ‘R’ is given as:
R ∝ A B
For any general reaction denoted by aA + bB → Products, the rate of the reaction according to the Law of Mass Action is indicated as:
R ∝ Aa Bb
How to Derive the Law of Mass Action?
Consider the following reversible reaction hypothetically:
aA + bB ⇌ cC + dD
According to the Law of Mass Action, the rate of forward reaction will be:
Ri ∝ Aa Bb
Therefore, Ri = Kf Aa Bb , where Kf is the forward reaction’s rate constant
Similarly, the backward reaction rate will be:
Rb ∝ Cc Dd
Or, Rb = Kb Cc Dd , where Kb is the backward reaction’s rate constant
Now, for a reaction to be in chemical Equilibrium,
Rf = Rb
Or Kf Aa Bb = Kb Cc Dd
Therefore, \[\frac{K_f}{K_b} = \frac{C^c D^d}{A^a B^b} \]
Or \[K_c = \frac{C^c D^d}{A^a B^b} \]
The above equation is known as the Mass Law equation, and Kc is termed as the Equilibrium constant.
What is the Equilibrium Constant in the Law of Mass Action Equation?
Kc or the Equilibrium constant is expressed as the ratio of the product of the Equilibrium concentrations of the products to the product of the Equilibrium concentration of the reactants, with each concentration term raised to the individual stoichiometric coefficients of the reactants and the products in the balanced chemical equation.
Explanation of the Law of Mass Action for a Gaseous System
For gaseous systems, the concentration terms are replaced by partial pressures. To explain the Law of Mass Action for such systems, we consider that the partial pressure of a gas is directly proportional to its concentration at a given temperature.
For the hypothetical reversible equation aA(g) + bB(g) ⇌ cC(g) + dD(g), the Equilibrium constant Kp in terms of partial pressures will be:
\[K_p = \frac{\begin{bmatrix} P_C \end{bmatrix}^c \begin{bmatrix}P_D\end{bmatrix}^d }{\begin{bmatrix}P_A\end{bmatrix}^a \begin{bmatrix}P_B \end{bmatrix}^b }\]
Concentration Quotient versus Equilibrium Constant
The Concentration Quotient (Qc)of a chemical reaction at a given temperature is defined as the ratio of the product of the concentrations of the products to that of the reactants. However, as the system reacts the value of Qc will fluctuate, but the Equilibrium concentrations will determine the Equilibrium constant Kc.
If Qc > Kc, the reaction will be occurring in the backward direction
If Qc < Kc, the reaction will be occurring in the forward direction
If Qc = Kc, the reaction shall remain in Equilibrium
Example of the Law of Mass Action
Consider the reaction 2NO2 ⇌ N2O4
Applying the Law of Mass Action formula, the expression for the Equilibrium constant for this reaction is:
\[K_c = \frac{\begin{bmatrix}N_2O_4\end{bmatrix}} {\begin{bmatrix} NO_2 \end{bmatrix}_2 } \]
Taking the values of [N2O4] = 0.0417 mol L-1 and [NO2] = 0.0165 mol L1,
\[K_c = \frac{0.0417}{0.01652} = 153\]
Applications of the Law of Mass Action
Ostwald’s Dilution Law for determining the dissociation Equilibrium of weak electrolytes.
Semiconductor physics.
For explaining diffusion in condensed matter.
For solving the model of disease dissemination in Mathematical Epidemiology.
FAQs on Law of Mass Action
1. What are the Factors Affecting the Rate of Chemical Reactions?
Concentration: The reaction rate increases with an increase in the concentration of reactants.
Pressure: Pressure influences gaseous reactions. These can be categorised as three types of gaseous reactions:
Reactions With an Increase in Volume: For reactions that are accompanied by an increase in volume, a reduction in pressure increases the rate of reaction.
Example: PCl5(g) ⇌ PCl3(g) + Cl2(g)
Reactions With a decrease in Volume: For reactions that are accompanied by a reduction in volume, an increase in pressure increases the rate of reaction.
Example: N2(g) + 3H2 ⇌ 2NH3 (g)
Reactions with No Change in Volume: Reactions, where volume remains unchanged are independent of changes in pressure.
Example: H2(g) + Cl2 ⇌ 2HCl (g)
Temperature: In general, reaction rates increase with an increase in temperature.
Catalysts: Catalysts increase the reaction rate.
Particle size: Solid reactants in powdered form provide a greater surface area to increase the reaction rate.
2. What is Le Chatelier’s Principle of Chemical Equilibrium?
Le Chatelier’s principle states that for reactions that are in dynamic equilibria, changes in concentration, pressure, or temperature shifts the position of Equilibrium to counteract the change and to establish a new Equilibrium state.
Concentration: Increasing the reactant concentration of a system will shift the Equilibrium towards the right, forming more products. Conversely, reducing the concentration of the product(s) will also shift the Equilibrium towards the right.
Pressure: If the volume of a system decreases, or pressure increases, the Equilibrium will shift in the direction that involves a lesser number of moles of gas. Similarly, if the pressure decreases and the volume of the system increases, the Equilibrium will favour the side that produces more number of moles of gas.
Temperature: For endothermic reactions, an increase in temperature will shift the Equilibrium to the right. Conversely, for exothermic reactions, increasing the temperature will shift the Equilibrium to the left.
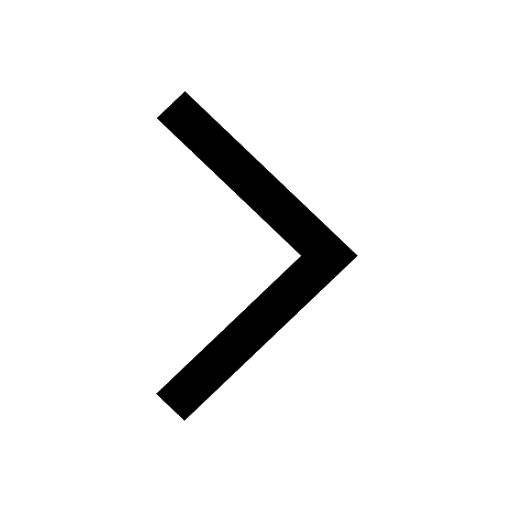
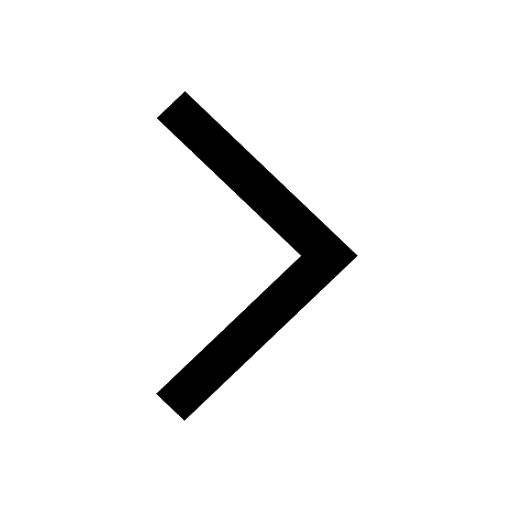
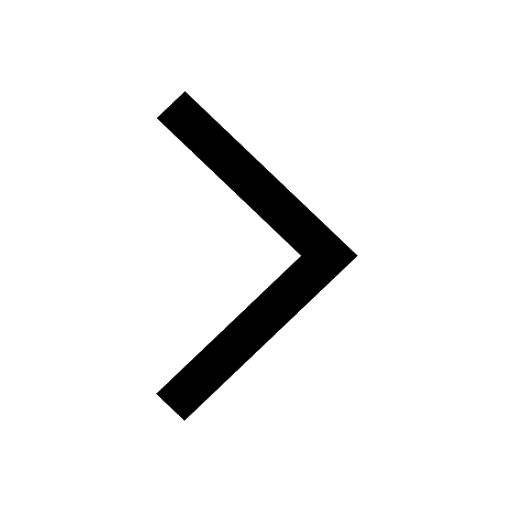
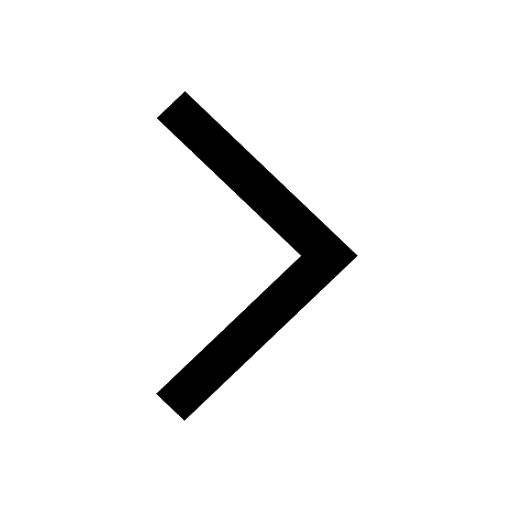
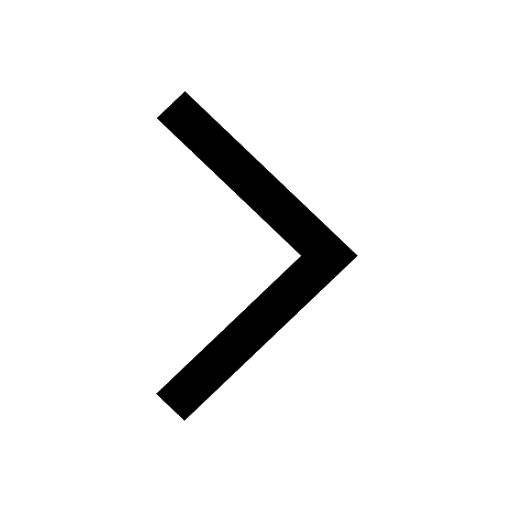
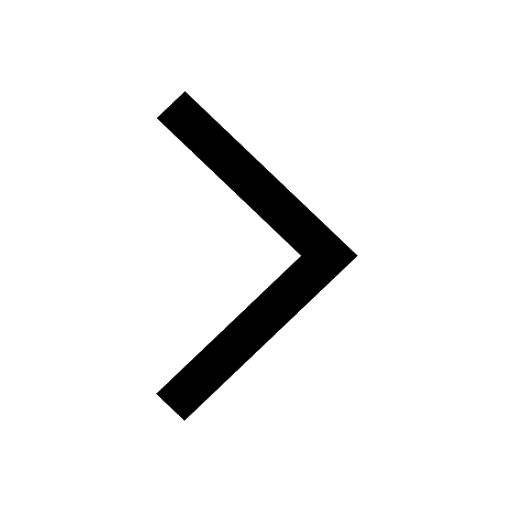