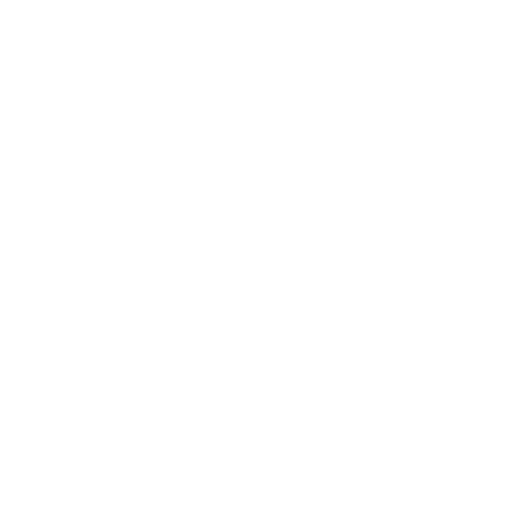
What is Magnetohydrodynamics?
Magnetohydrodynamics is one of the interesting sub-branch of physics, where we analyse fluid dynamics with the help of magnetic effects. The word magnetohydrodynamics is derived from the words magneto (implies magnetic field), hydro (meaning water) and dynamics refer to movement. So, also known as magneto-fluid dynamics or hydromagnetics is the study of magnetic properties and behaviour of electrically conducting fluids. Examples of such magneto fluids are plasma, liquid metal (such as mercury), saltwater and electrolytes.
Magnetohydrodynamics is abbreviated as MHD. The basic principle behind MHD is that magnetic fields can induce a current in a moving conducting field which in turn polarizes the fluid and as a result changes the magnetic field. In this article, we will have an introduction to magnetohydrodynamics along with a discussion of some principles of magnetohydrodynamics.
[Image will be Uploaded Soon]
Ideal MHD
Magnetohydrodynamics or hydromagnetics couples Maxwell's equations with hydrodynamics to describe the macroscopic behaviour of highly conducting fluids such as plasmas or liquid metals. In an ideal MHD, the plasma fluid is considered as an electrically conducting fluid and the governing equations of ideal MHD are equations of fluid dynamics and Maxwell’s equations. Any movement of conducting material in an external field generates an electric current(I), which in turn induces the magnetic field. A self-consistent set of ideal MHD equations co-relates the plasma mass density ρ, the plasma velocity V, the thermodynamic (also called gaseous or kinetic) pressure P and the magnetic field B.
The plasma equilibrium can be easily perturbed and as a result of perturbation small amplitude and fluctuations can be excited. For convenience, while considering the small waves one should always start with ideal plasmas. The damping waves require some kind of dissipation which will not be considered here. The ideal MHD equations derived with ideal ohms law and space charges, in the standard form, is given by:
⇒ \[\frac{\partial n}{\partial t}\] + Δ • (nv) = 0
⇒ \[\frac{\partial (mnv)}{\partial t}\] + Δ • (nmvv) = - Δ • (P + \[\frac{B^{2}}{2\mu_{0}}\]I) + \[\frac{1}{\mu_{0}}\] Δ • (BB)
⇒ \[\frac{\partial B}{\partial t}\] = Δ x (v x B)
⇒ Δ • B = 0
This set of equations are known as the ideal MHD equations and these are derived from Maxwell's equations.
MHD Physics:
Magnetohydrodynamics plays an important role in several branches of physics, such as solar physics where we will study the magnetohydrodynamics of the sun, astrophysics, plasma physics, etc. The MHD physics is mainly concerned with the effects of the magnetic field on the dynamic conducting fluid. The fundamental principle of magnetohydrodynamics is that magnetic fields can induce the electric current in a dynamic conducting field which in turn polarizes the fluid and as a result of the polarization in turn it will also change the magnetic field. Hence, the MHD is also relativistic in nature and sometimes it is also referred to as relativistic magnetohydrodynamics or general relativistic magnetohydrodynamics.
The MHD physics incorporates certain approximations which are also known as the MHD approximations or the ideal MHD approximations.
MHD Approximations:
The most important ideal MHD approximations are as follows:
MHD is a low-frequency, long-wavelength approximation.
The magnetohydrodynamics is valid for length scales more than the Debye length and electron/ion gyroradii. I.e., L >> λ\[_{D}\], ρ\[_{e}\], ρ\[_{i}\]
The magnetohydrodynamics is valid for a long time scales more than the inverses of the plasma frequency and the electron/ion cyclotron frequencies: τ >> ω\[_{P}^{-1}\], Ω\[_{e}^{-1}\], Ω\[_{i}^{-1}\]
In ideal MHD and MHD physics, we assume quasineutrality (since L >> λ\[_{D}\])
In ideal MHD, we assume that collisions are frequent enough (they occur at regular interval) for the particle distribution function to be Maxwellian and T\[_{i}\] = T\[_{e}\].
In magnetohydrodynamics, we assume an adiabatic equation of state (no additional heating) and no dissipation.
Ignore the most significant physics advances since 1860,
Relativity (V\[^{2}\] << c\[^{2}\]), which is generally referred to as general relativistic magnetohydrodynamics.
Quantum mechanics
Displacement current in Ampere’s law (again, since V\[^{2}\] << c\[^{2}\])
Applications of Magnetohydrodynamics:
There are several applications of MHD physics because of its relativistic study. Let us have a look at a few important applications of Magnetohydrodynamics.
MHD traditionally describes macroscopic force balance, equilibria, and dynamics. Ideal MHD describes dynamics reasonably well on large scales
Ideal MHD is a good predictor of plasma stability. One of the interesting facts is that most catastrophic instabilities are unstable in ideal MHD. MHD physics is found to be important in laboratory plasmas, solar atmosphere, etc.
Systems that are described reasonably well by MHD include, Solar wind, heliosphere, and Earth's magnetosphere, Inertial range of plasma turbulence, Neutron star magnetospheres.
MHD is a reasonably good approximation in most astrophysical plasmas. However, extensions are often needed.
Just like every advancement in technology has pros and cons, even magnetohydrodynamics is also having its own drawbacks. Magnetohydrodynamics can not be useful all the time, there are certain limitations to the applications of MHD.
Drawbacks of MHD:
Magnetohydrodynamics has limited applicability when:
Non-fluid or kinetic effects are important:
Dissipation in the turbulent solar wind
Magnetic reconnection
Small-scale dynamics in Earth’s magnetosphere
The particle distribution functions are not Maxwellian, like Cosmic rays.
The plasma is weakly ionized:
Solar photosphere /chromosphere, molecular clouds, protoplanetary disks, Earth’s ionosphere, some laboratory plasmas.
MHD is mediocre at describing the dynamics of laboratory plasmas but remains a good predictor of stability.
These are a few drawbacks of MHD, in other words, the limitations of MHD.
Did You Know?
The term magnetohydrodynamics was first introduced by Alfven. He was the one who described the astrophysical phenomenon as an independent scientific discipline. But the official origin or the birth of non-compressible magnetohydrodynamic fluid is in the year 1936-37. Hartmann and Lazarus conducted a theoretical and experimental study of MHD fluid flow in ducts.
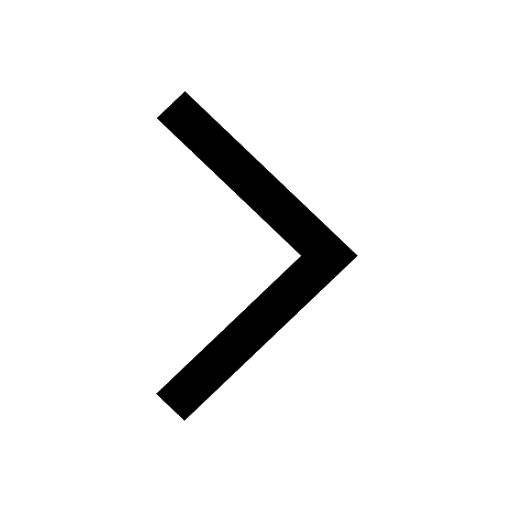
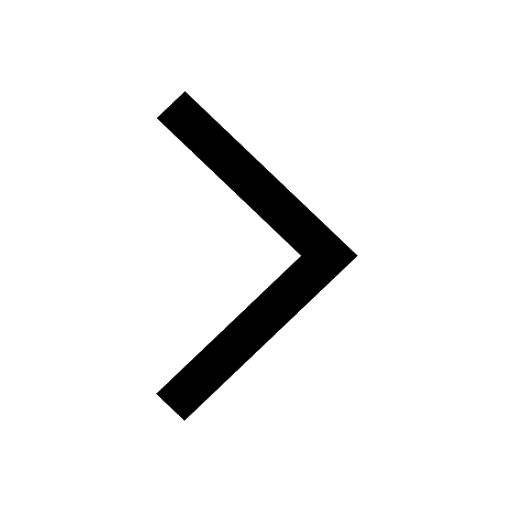
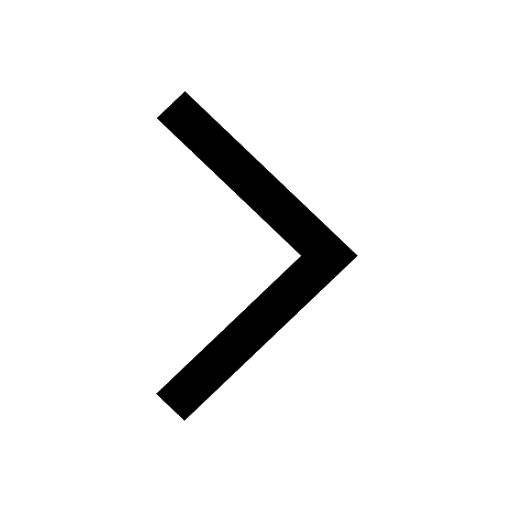
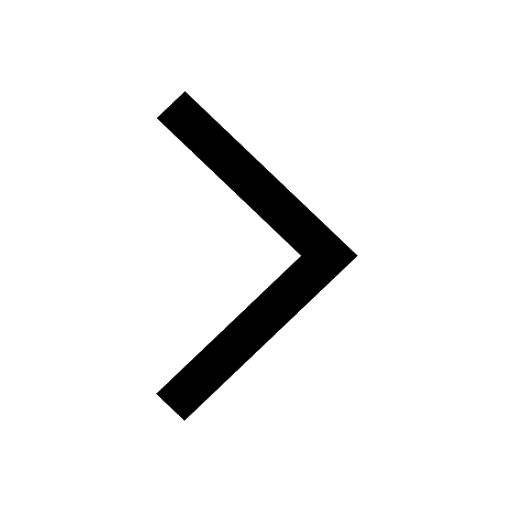
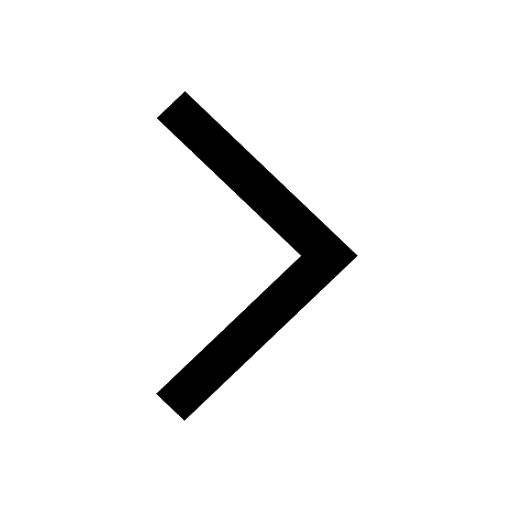
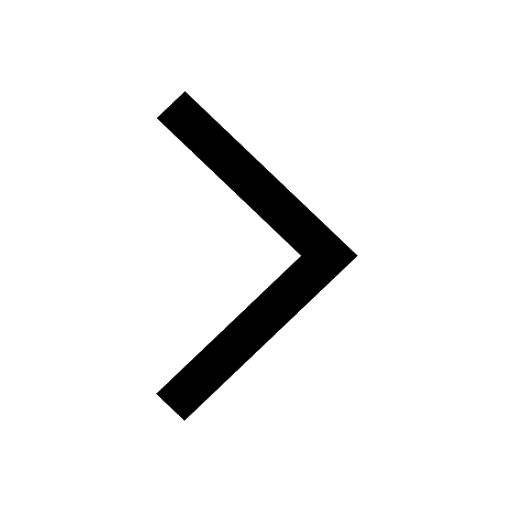
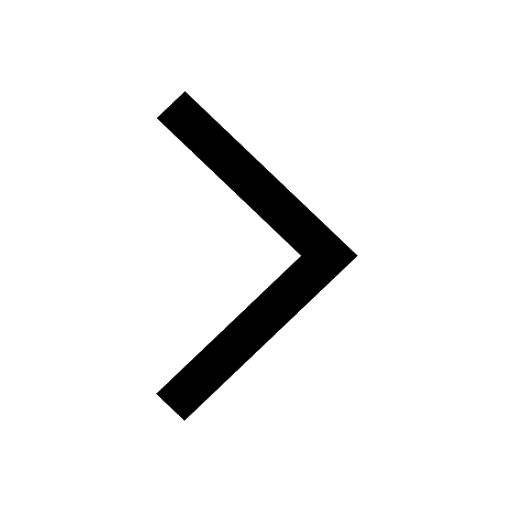
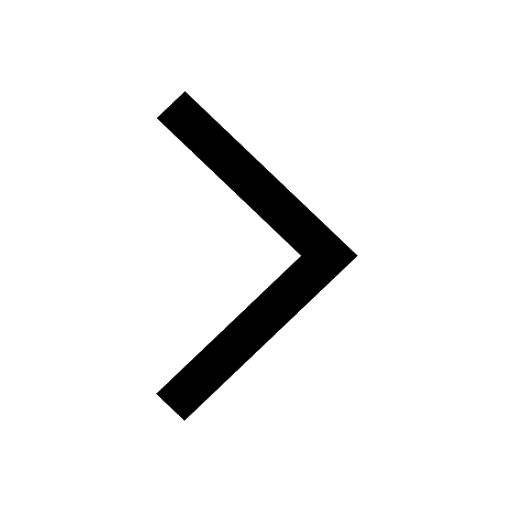
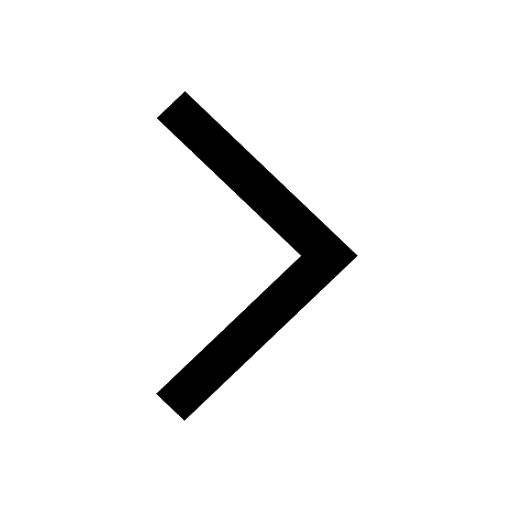
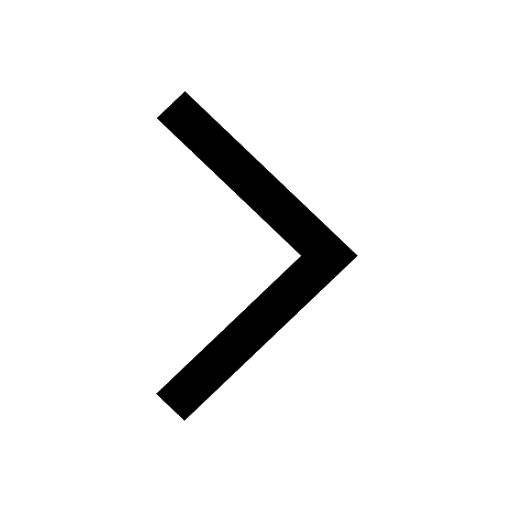
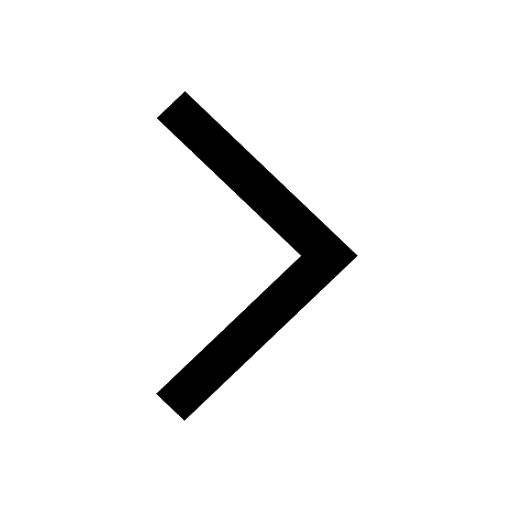
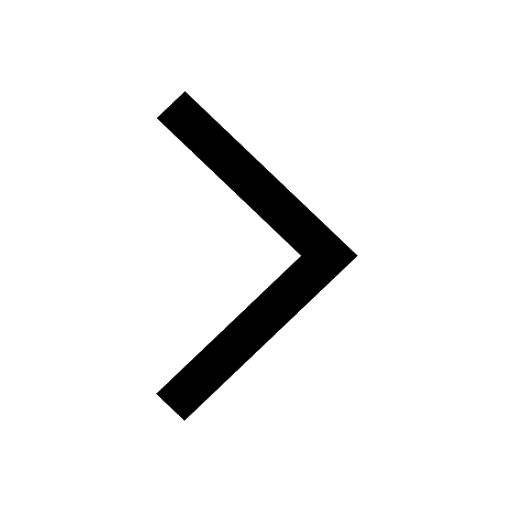
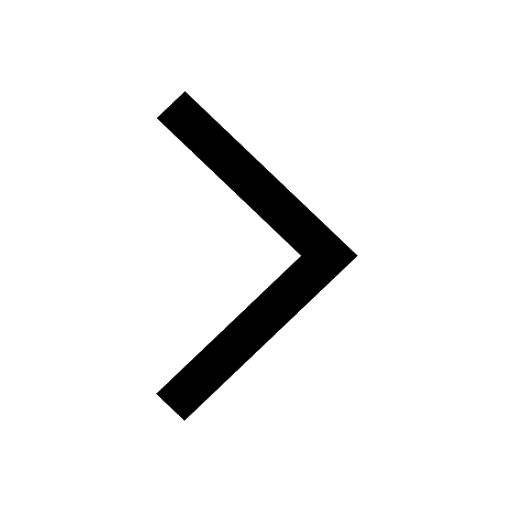
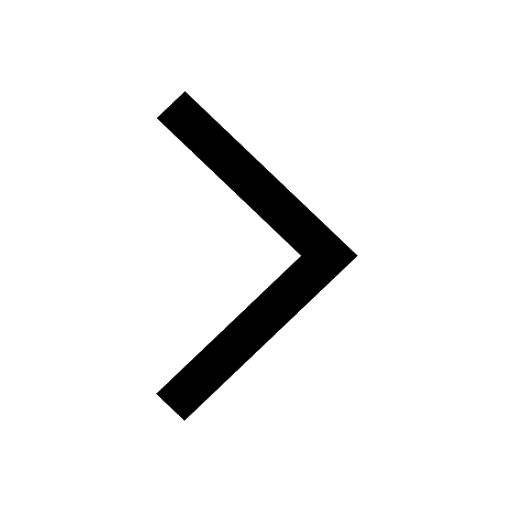
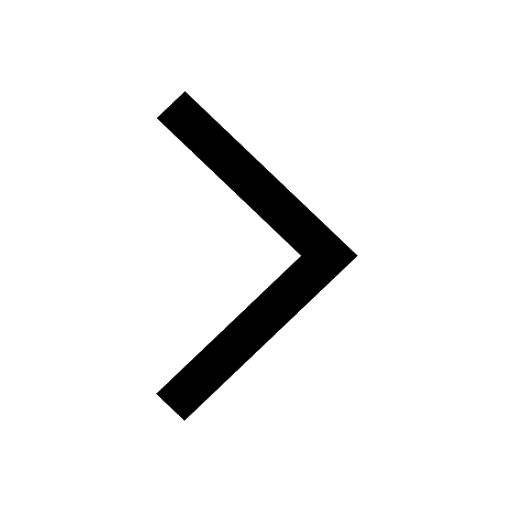
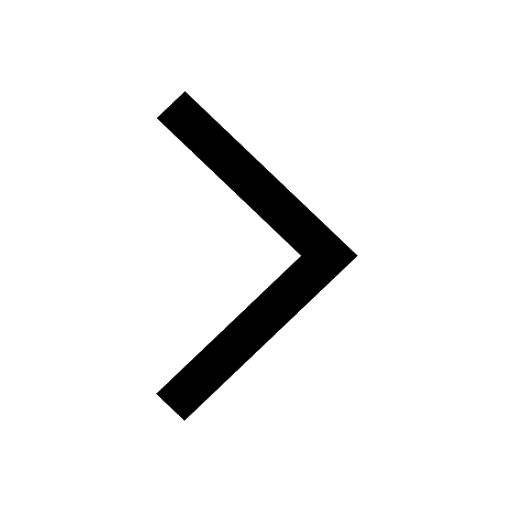
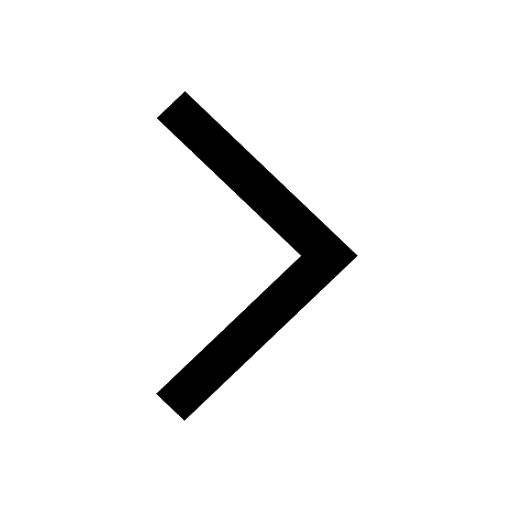
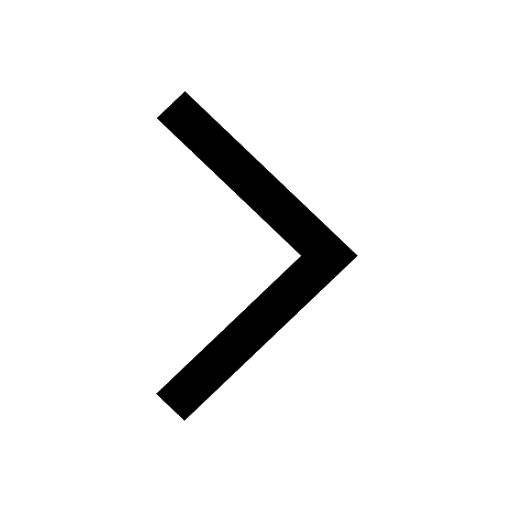
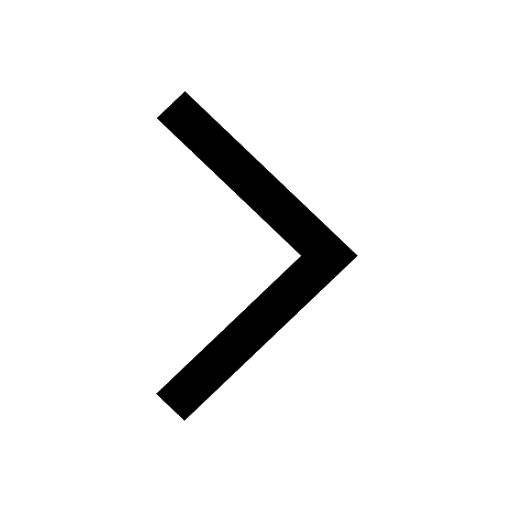
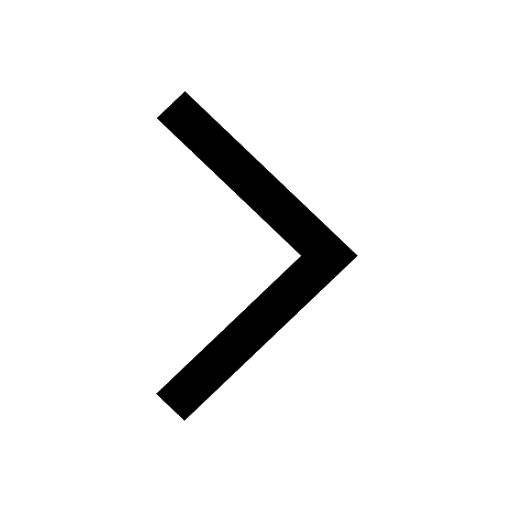
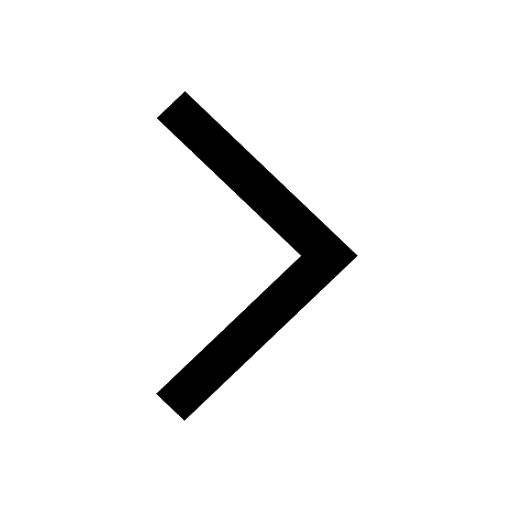
FAQs on Magnetohydrodynamics
1. What is the Meaning of Magnetohydrodynamics?
Ans: Magnetohydrodynamics (or MHD) the word is basically the combination of two terms or words magneto (implies magnetic field), hydro (meaning water) and dynamics refer to movement. So, magnetohydrodynamics (or MHD) which is also known as magneto-fluid dynamics or hydromagnetics in general, is the study of magnetic properties and behaviour of electrically conducting fluids. There are many examples of magneto fluids, few familiar ones are the plasma fluid, mercury (liquid metal), brine and electrolytes.
2. What is the Magnetohydrodynamic Effect or MHD Effect?
Ans: The Magnetohydrodynamic Effect or the MHD effect is a physical phenomenon describing the motion of a conducting fluid flowing under the influence of an external magnetic field.