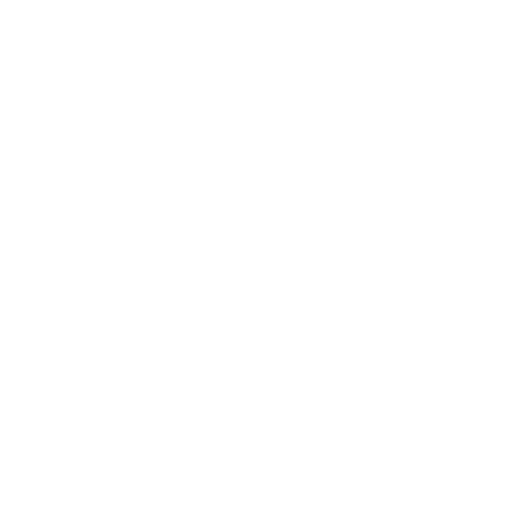

Nuclear Chemistry - Types, Nuclear Fission and Fusion and Applications
The discovery of radioactivity opened up the way for the creation and development of nuclear chemistry in the early twentieth century. In the mid-twentieth century, novel findings and the Second World War ushered in the Nuclear Age. From nuclear power generation to war damage, nuclear chemistry has shown tremendous potential. The wide proliferation of the area has brought about a wide variety of nuclear chemistry topics - what is nuclear chemistry, nuclear radiations, artificially simulated nuclear reactions (fission and fusion), and the uses of nuclear chemistry.
Nuclear Chemistry
Nuclear chemistry is the study of the chemical and physical properties of elements that deal with nuclear reactions or reactions that happen inside the structure of the nucleus. Modern nuclear chemistry sometimes referred to as radiochemistry, has become highly interdisciplinary in its applications, from the study of element formation in space to the design of radiopharmaceuticals for diagnostic medicine. In fact, the chemical technology developed by nuclear chemists has become so important that biologists, geologists, and physicists use nuclear chemistry as a common tool in their disciplines.
Nuclear chemists can be found in a variety of research areas, including nuclear imaging and nuclear technology. They often work to improve the efficiency and safety of nuclear energy sources and the way radioactive materials are stored and disposed of.
Nuclear chemists carry out basic research, applied research, or theoretical research. You often work in the laboratory and may be responsible for the operation, maintenance, and repair of state-of-the-art equipment. You are also responsible for the maintenance of sample preparation materials and equipment, and for the safe use and disposal of samples and other materials used in the laboratory.
Marie Curie, who was the founder of nuclear chemistry, was intrigued by Antoine Henri Bekrel's discovery that photographic film can emit light that can be exposed even when uranium minerals are wrapped in black paper. Using an electrometer that could measure the electrical conductivity of air (the predecessor of the Geiger counter) invented by her husband Pierre and his younger brother Jack, she was able to prove that thorium also produces these rays. This process is what she called radioactivity.
What Is Nuclear Chemistry?
Nuclear chemistry is a sub-discipline of chemistry dealing with the study of changes in the nucleus of atoms of elements. These nuclear changes are a source of nuclear power and radioactivity, and the energy released from the nuclear reactions has far-reaching applications. Nuclear chemistry is also termed radiochemistry, which involves the study of the elements composing the universe, design, and development of radioactive drugs for medicinal uses, and several other scientific applications.
Nuclear Radiations
Nuclear radiation refers to the photons and particles that are emitted during nuclear reactions. The particles emitted in nuclear reactions possess an energy that is tremendous enough to knock electrons from atoms and molecules, thereby ionizing them. For this reason, nuclear radiation is also known as ionizing radiation.
Nuclear radiations include alpha rays, beta rays, and gamma rays. Nuclear reactions release ionizing subatomic particles, including alpha particles, neutrons, beta particles, mesons, muons, positrons, and cosmic rays. For example: during Uranium-235 fission, the nuclear radiation that is emitted contains gamma-ray photons and neutrons.
Types of Radiations
Alpha Radiation: Alpha radiation is the emission of alpha particles when an atom goes through radioactive decay. An alpha particle consists of two protons and two neutrons and is similar to a Helium-4 atom. Thus, the resulting element has an atomic number less by two units and an atomic mass less by four units than that of the originating element. Example: Uranium-238 undergoes alpha decay in the following manner:
23892U → 23490Th + 42He
Beta Radiation: Consists of a stream of high-speed electrons. Beta-decay is of two types –beta plus and beta minus. In beta plus decay, the nucleus emits a positively charged electron (positron) and a proton that is converted into a neutron (neutrino). In beta minus decay, the nucleus emits a neutron that is transformed into a proton (antineutrino) and an electron.
Beta minus decay: 1n → 1p+ + 0-1β- + v̅
Beta plus decay: 11p+ → 10n + 01β + v
127N ⟶ 612C + 01β+
146C ⟶ 147N + 0-1β
Gamma Radiation: Gamma radiation (γ) does not consist of any particles. Instead, it involves photons of energy being emitted from an unstable radioactive nucleus. Gamma rays are electromagnetic radiations of short wavelengths and have no charge or mass. These rays represent the loss in energy when the remaining nucleons undergo stable rearrangements, and thus, gamma rays accompany other radioactive emissions. Example:
23892U → 23490Th + 42He + 200γ
Nuclear Fission
Nuclear Fission is an artificially simulated nuclear reaction where a heavy nucleus splits into two lighter nuclei. Fission was discovered by bombarding a sample of Uranium-235 with neutrons, which resulted in the production of lighter elements like Barium. In a typical nuclear chain reaction, each dividing nucleus releases more than one neutron, which, in turn, collides with neighboring nuclei and induces a succession of self-sustaining nuclear fission reactions. The fission rate increases geometrically with each generation of events.
(image will be uploaded soon)
Nuclear Fusion
Nuclear Fusion is also an artificially simulated nuclear reaction in which two or more nuclei of elements combine to produce a heavier and more stable nucleus. The initiation of the fusion process requires very high temperatures, which are obtained from nuclear fission reactions. Nuclear Fusion generates explosive amounts of energy, which is the source of power for the sun and all the stars. Examples: deuterium-deuterium (D-D) fusion, deuterium-tritium (D-T) fusion.
2 21H → 32He + 10n
21H + 31H → 42He + 10n
Nuclear Radiations
Nuclear radiation is the phenomenon of particles being emitted by atomic nuclei in the form of alpha rays, beta rays, gamma rays.
The particles emitted during a nuclear reaction are strong enough to ionize the electrons by removing them from atoms and molecules.
Types of Radiations
Alpha Radiations
Alpha rays are heavy particles and have a very short range, so they don't go that far. This means that alpha particles cannot penetrate even a piece of paper. Alpha particles outside the body do not even pass through the surface of the skin. However, inhaling or ingesting substances that emit alpha particles can expose delicate tissues such as the lungs. For this reason, high-level radon in your home is considered a problem.
Beta Radiations
Beta particles can travel a little further than alpha particles. You can use a relatively small shield to stop them. They can get into your body, but they can't penetrate it completely through it. To be useful in medical imaging, beta particles must be released through substances injected into the body. If radioactive substances can be introduced into tumors, they will also be very useful in treating cancer.
Gamma Radiations and X-rays
Gamma rays and X-rays are highly penetrating electromagnetic radiation that can penetrate through the body. They are proven to be useful in a medicine-to show if the bones are broken, where the cavities are, or to identify the tumor. Shields made of high-density materials such as concrete and lead are used to avoid exposure to sensitive internal organs and those who may handle this type of radiation.
Applications of Nuclear Chemistry
Agriculture
Plant mutation breeding to achieve improved nutrition and food security.
Management of fertilizer use through Radiolabelling.
Controlling insect populations.
Consumer products
Smoke detectors, non-stick materials, clocks, and watches utilize radioisotopes.
Food
Food irradiation with gamma rays to prevent spoilage and enhance shelf-life.
Pest control.
Industry
Radioactive tracers find use in industrial processes.
Inspection of instruments.
Carbon dating.
Nuclear desalination of water.
Medicine
MRI scans, CT scans, and X-rays for diagnosis.
Radioactive Iodine is used for the treatment of cancers.
Sterilization of medical instruments.
Transport
Nuclear-powered submarines and ships.
Radioisotope thermal generators for electricity production in space missions.
FAQs on Nuclear Chemistry
1. What are the work duties of the nuclear chemist?
There are various duties of a nuclear chemist, few includes-
Conducting research in laboratories of profit, non-governmental, universities, and industries.
Developing nuclear phenomenon stimulations on computer
Teaching and mentoring university students
Development of nuclear energy sources for utilities, submarines or satellites, and other spacecraft
Researching on the treatment of diseases where radiation are needed
2. What do you understand from Residual nuclear radiation?
Residual radiation from a nuclear explosion is primarily due to fallout that occurs from weapon debris, fission products, and, in the case of tremors, the radiated soil.
3. What are a few skills and abilities useful in the field of nuclear chemistry?
Few skills needed are-
Problem-solving skills
Critical and analytical thinking to quickly troubleshoot any issue
Good mathematical skills
Computer knowledge and coding
4. What are a few positive effects of radiation?
They are very useful in the medical field to detect cavities and tumors in a patient. Radiation treatments for cancer patients can prevent further cancer growth and even shrink the existing tumors.
5. How can radiation harm the human body?
Radiation can be very harmful if used incorrectly and for wrong purposes without the consultation of an expert. Radiation is given a bad public image because some people try to take advantage of the effects of radiation to harm others.
6. How are nuclear reactions different from chemical reactions?
In chemical reactions, bonds are broken and formed between two different atoms, whereas in nuclear reactions, the nuclei of a single atom emit particles or rays.
Atoms themselves remain unchanged but undergo rearrangements in chemical reactions. On the other hand, nuclear reactions involve a change in the nuclear composition and hence, a transformation of one atom to another type.
While chemical reactions involve valence electrons, nuclear reactions include electrons, protons, and neutrons.
Chemical reactions entail small energy changes. But nuclear reactions are accompanied by substantial changes in energy.
The factors influencing the rate of a chemical reaction include temperature, reactant, and product concentration, pressure, and the presence of catalysts. However, nuclear reactions are unaffected by any of these factors, and their rate can vary between a few milliseconds or millions of years.
7. What is transmutation in Nuclear Chemistry?
Nuclear transmutation is the conversion of an atom of one element into an atom of another element through nuclear reactions. Transmutation can occur in two ways – when the nucleus of an atom decays radioactively, or when the nucleus reacts with another particle. In induced nuclear transmutation, the nuclei are bombarded with high volume particles to induce transmutation. In 1919, Ernest Rutherford carried out the first nuclear transmutation. He used high-speed α particles from a natural isotope of radioactive radium to bombard nitrogen atoms. The reaction is:
147N + 42He →178O + 11H
The reaction resulted in the formation of protons and oxygen nuclei, which were stable, and thus, no further nuclear changes took place.
Particle accelerators are used to achieve the kinetic energy necessary for transmutation reactions. These devices utilize electric and magnetic fields to accelerate nuclear particles; the latter move in vacuum to avoid smashing with gas molecules.
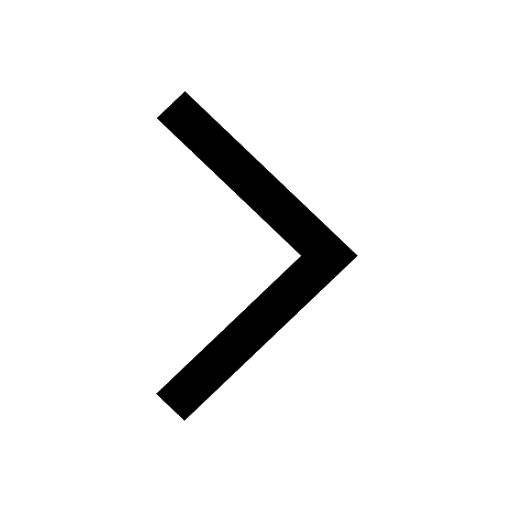
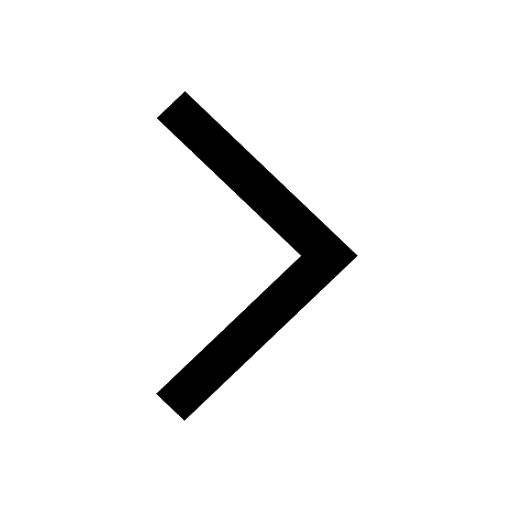
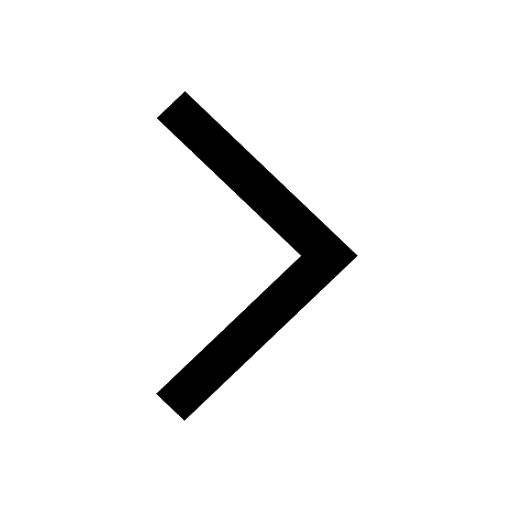
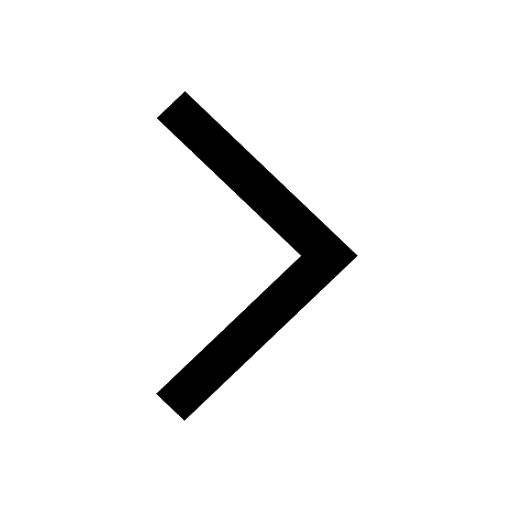
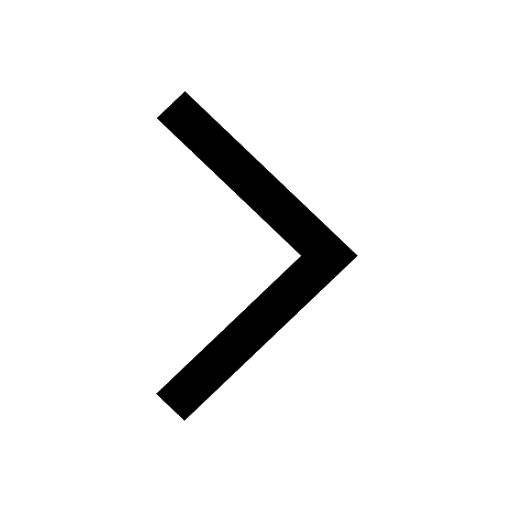
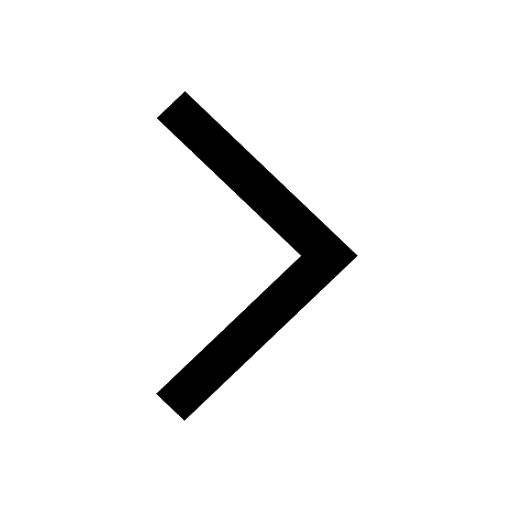