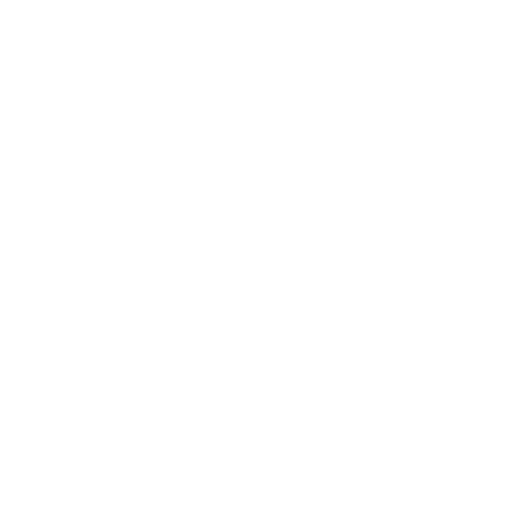

Introduction to Fine Structure
In atomic physics and quantum effects on atoms, the study of the hydrogen atom and their spectrum plays an important role. When the hydrogen spectrum was studied, physics noticed that the familiar red spectral line of the hydrogen atom consists of two closely spaced lines. That means the spectral line was split into two closely spaced lines or closely spaced doublet. The splitting of spectral lines is known as the fine structure or fine structure of spectral lines and it is considered one of the first pieces of experimental evidence for the electron spin.
Fine Structure of Hydrogen Atom
The fine structure of the hydrogen atom is also known as the hydrogen fine spectrum. We know that the hydrogen atom is one of the simplest forms of atom available, which consists of a single electron in its valence shell. Before we start with the fine structure of the hydrogen atom let us have a look at the spectrum of the hydrogen atom. The spectrum of a hydrogen atom consists of different series of spectral lines and these sets of spectral lines fall into a different region of the electromagnetic spectrum, for example, the Balmer series lies in the visible region of the electromagnetic spectrum.
Now, what is the fine structure of a hydrogen atom? When we examine the Balmer series of spectral lines we know that it consists of four different spectral lines corresponding to violet, blue, green, and red wavelengths. When spectral lines of the hydrogen spectrum were examined under a high-resolution spectrometer it was found that a single spectral line appears to be resolved into two pairs of closely spaced single lines such that these split lines will be having slightly different wavelengths. This splitting spectral line is known as the fine structure of a hydrogen atom.
When the red spectral line which is also known as The line is closely examined with high-resolution spectrometers, physicists found that it consists of two closely spaced doublet lines due to spin-orbit coupling. We know that the electrons are revolving around the nucleus in definite orbitals and due to the orbital motion of electrons a magnetic field is generated. When the spin electron magnetic moment interacts with the magnetic field, this interaction is familiarly known as spin-orbit coupling.
In atomic spectroscopy, the energy levels of electrons of an atom are given by the formula:
\[n^{2s+1}l_{j} ... (1)\]
Where,
n - The principal quantum number
s -The spin angular momentum quantum number
l -The orbital angular momentum quantum number
j -The total angular momentum quantum number (i.e., the sum of both spin and orbital angular momentum i.e., \[(l \pm s)\]
Depending upon the value of l different orbits or energy levels are designated, for example, for l =0 we have S-orbit, for l =1 we have P-orbit, and so on.
Fine Spectrum
The fine structure of the spectral line describes the splitting of spectral lines due to the electron spin and the relativistic correction to the total energy of the hydrogen atom electron. When electrons transit from lower energy levels to higher energy levels by absorbing the energy, they will be unstable and hence lose their energy in the form of photons of different wavelengths that further result in a spectrum.
The interaction between the magnetic field generated due to the relative motion of the nucleus and the electron spin angular momentum will result in the splitting of the energy of electrons into two energy levels.
The electron with \[+\frac{1}{2}\] will have a magnetic spin momentum and experience a torque due to the presence of a magnetic field and hence it will rotate it, at the same time, the electron with \[-\frac{1}{2}\] will also have some magnetic spin momentum and experience a torque due to the presence of magnetic field and hence it will rotate it in opposite direction. As the electron rotates, there will be a change in its internal energy and it is given by:
\[\Rightarrow U = -\mu B\]
(Note: since they rotate by a different amount, hence they will also have a different amount of energy)
Suppose that the electron in hydrogen atom transit from 1s level to 2P level, we know that the motion of the electron is associated with the orbital quantum number and the spin quantum number. When the electron is in the 1S state it is in its orbit and hence a single energy level is obtained, whereas the 2p state due to spin-orbit interaction splits into two levels. Mathematically, we write:
\[\Rightarrow j = (1\pm s) ...(1)\]
For P-orbit the value of l is 1and we know that the spin quantum number of the electron is 12. substituting, these values in equation (1) we get,
\[\Rightarrow j = \frac{3}{2} , \frac{1}{2}\]
(Image will be Uploaded Soon)
Thus 2P level is split into two energy levels. Thus, when the transition of an electron from 1S to 2P is observed we notice only a single spectral line, when it is observed through a high-resolution spectrometer, we notice that there are two closely spaced spectral lines with slightly different wavelengths, and this splitting of spectral lines is known as the fine structure of hydrogen atom or the fine spectrum.
Fine Structure of H Alpha Line
The H-alpha(H)line is a specific deep-red visible spectral line found in the Balmer series and the wavelength of the H-alpha is around 656 nm. The H-alpha line originates when the electron transit from its third to second lowest energy level. The H-alpha line is one of the brightest spectral lines in the Balmer series.
(Image will be Uploaded Soon)
More About Fine Structure:
The fine structure is the breaking of an atom's primary spectral lines into two or more components, each indicating a slightly different wavelength, in spectroscopy. When an atom transitions from one energy state to another, it produces light, which creates a fine structure. The split lines, also known as the fine structure of the main lines, result from the interaction of an electron's orbital motion with its quantum mechanical "spin." An electron may be compared to an electrically charged spinning top, and as a result, it behaves like a little bar magnet. The fine structure is created when a spinning electron interacts with the magnetic field created by the electron's revolution around the atomic nucleus.
The fine-structure constant is a dimensionless constant that describes the amount of splitting.
Alkali metal atoms, such as sodium and potassium, have two fine structure components (called doublets), whereas alkaline earth atoms have three fine structure components (called triplets) (triplets). This is because alkali metal atoms have only one electron outside of a closed core, or shell, of electrons, whereas alkaline earth atoms have two. With increasing atomic number, doublet separation for matching lines rises; therefore, a doublet with lithium (atomic number 3) may not be resolved by an average spectroscope, but a doublet with rubidium (atomic number 37) may be widely separated.
When a light source is put in a magnetic field, the Zeeman effect occurs, dividing a spectral line into two or more components of slightly different frequencies. Pieter Zeeman, a Dutch scientist, first noticed it in 1896 as a thickening of the yellow D-lines of sodium in a flame held between strong magnetic poles. Later, it was discovered that the widening was caused by a distinct splitting of spectral lines into up to 15 components.
Zeeman's Discovery
Zeeman's discovery won him the Nobel Prize in Physics in 1902, which he shared with fellow Dutch physicist, Hendrik Antoon Lorentz. Lorentz theorized that the oscillations of electrons inside an atom generate light and that a magnetic field would impact the oscillations and hence the frequency of the light emitted. Lorentz had previously devised a hypothesis concerning the effect of magnetism on the light. This idea was validated by Zeeman's study and later updated by quantum mechanics, which states that as electrons transition from one discrete energy level to another, spectral lines of light are emitted. In a magnetic field, each of the levels, which is defined by its angular momentum (a quantity related to mass and spin), is divided into substates of equal energy. The ensuing patterns of spectral line components reflect these energy substrates.
The Zeeman effect has aided physicists in determining and identifying the energy levels of atoms in terms of angular momenta. It also makes it possible to investigate atomic nuclei and phenomena like electron paramagnetic resonance. The pattern and quantity of splitting indicate the presence of a magnetic field as well as its intensity. The quantum number L can have non-negative integer values. The magnetic field splitting in terms of levels may be determined using the formula 2* L+1.
The Balmer series lines we observe are creatively referred to as alpha, beta, and gamma.
When an electron travels between the second and third orbits (N=2 and N=3), a line forms in the red section of the spectrum, and the wavelength at which this happens is 656nm. The Hydrogen-alpha line is named after it, and hydrogen alpha filters are designed to block out as much of the spectrum as possible, leaving just a very small bandwidth for light with the H-alpha frequency to pass through.
Did You know?
Spectral lines give information on the nucleus. The main effects are isotope shift and hyperfine structure.
The study of the hyperfine structure of the H alpha line is of importance in many fields of science. The emission of the H alpha line determines many features of the solar atmosphere including prominences and the chromosphere.
FAQs on Fine Structure
1. What is the Fine Structure of the H Alpha Line?
On examining the Balmer series of spectral lines of hydrogen atoms we know that it consists of four different spectral lines corresponding to different wavelengths. The H-alpha(H)line is a specific deep-red visible spectral line found in the Balmer series and the wavelength of the H-alpha is around 656 nm. The H-alpha line originates when the electron transit from its third to second lowest energy level.
2. What is the Reason for the Splitting of Spectral Lines?
The main reason for the splitting spectral lines is due to spin-orbit interactions. Thus the reason for the splitting of spectral lines is only due to the electron motion resulting in spin-orbit coupling. The interaction of the spin electron magnetic moment with the magnetic field is familiarly known as spin-orbit coupling.
3. Define the Normal Zeeman Effect.
The Normal Zeeman effect is one of three different types of Zeeman effects. The Normal Zeeman Effect is the splitting of spectral lines in an atomic spectrum caused by the interaction between the momentum of the orbital magnetic field and the external magnetic field. In the absence of electron spins, this phenomenon can be observed.
When energy is transferred to an atom, the atom becomes excited. That atom's electrons can consume energy and migrate to a higher energy level. Similarly, all of that atom's electrons can absorb energy and migrate to higher energy levels. This gives the atom's absorption spectrum. It can also be seen in the measured spectrum as a triplet rather than a single spectral line in the predicted scope.
4. What do you mean by the Paschen back effect?
The energy levels of atoms are broken apart in the presence of a substantial external magnetic field. The Zeeman effect defines this splitting well if the splitting is small relative to the energy variance between the unaffected groups, i.e. for sufficiently weak magnetic fields. If the magnetic field is large enough, it disrupts orbital coupling and spins angular momentum, resulting in a new Zeeman splitting pattern. The Paschen-Back effect is the name given to this phenomenon. The Paschen-Back effect has astronomical relevance since it is detected in some lithium spectra on the Sun.
5. What do you mean by the H alpha line?
In the air, H-alpha is a deep-red visible spectral line in the Balmer series with a wavelength of 656.28 nm that occurs when a hydrogen electron falls from the third to the second-lowest energy level. In the visible region of the hydrogen spectrum, the H - line is the first line. It's the line that came out of the Balmer series. When an electron goes from its third-lowest to its second-lowest energy state, this happens.
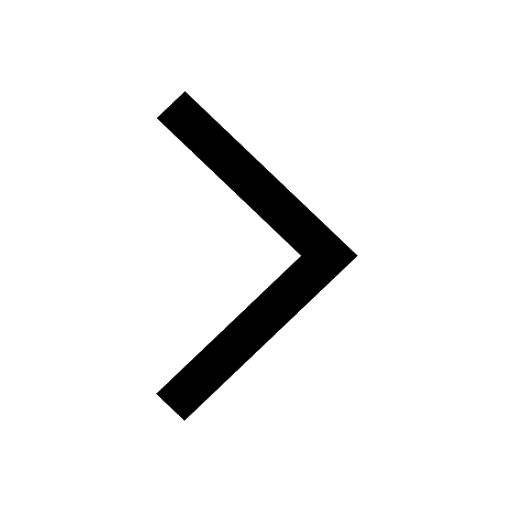
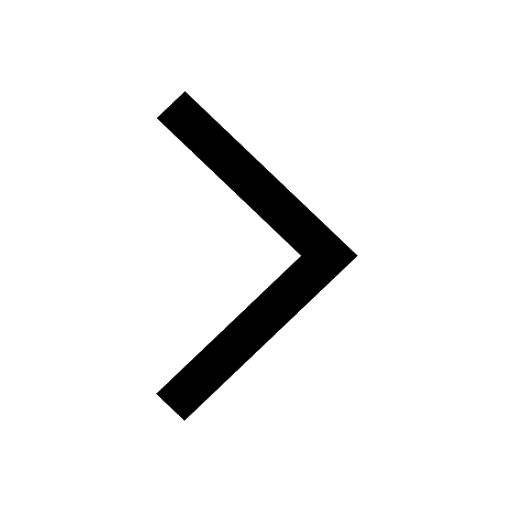
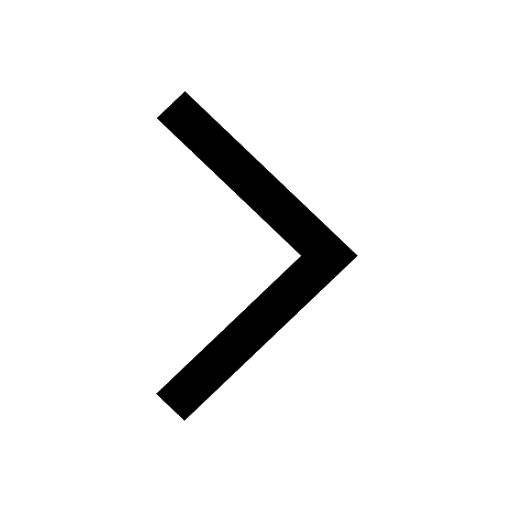
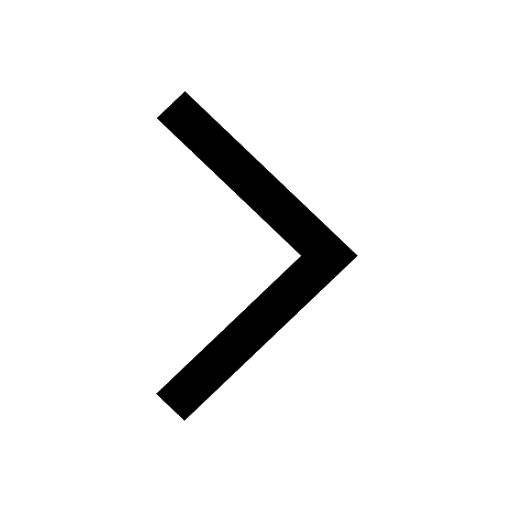
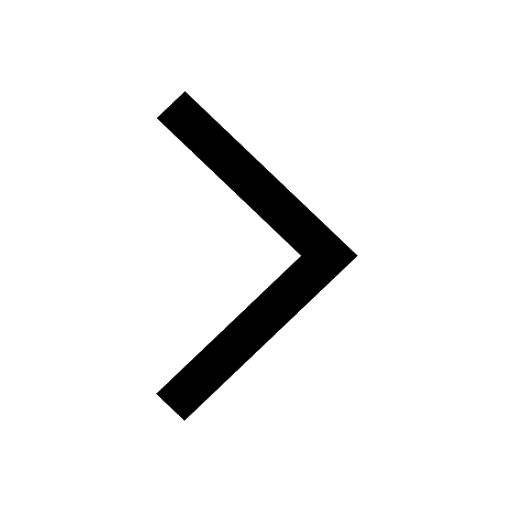
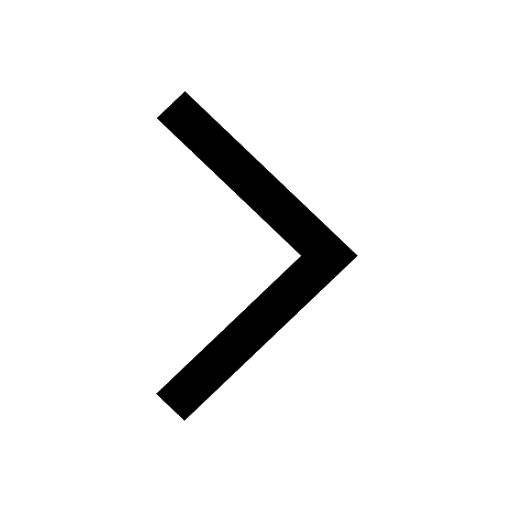