Answer
405k+ views
Hint In this solution, we will use the law of conservation of energy which tells us that energy cannot be created or destroyed but can only be converted into one form to the other. We will also use the law of conservation of momentum which tells us that the net momentum of a system where the net force is zero, is also zero.
Complete step by step answer
We’ve been given that two bodies of mass 10 kg and 20 kg are kept at a distance of 1 m. At this point, the system of two masses will have only gravitational potential and no kinetic energy since they are not moving.
When the two masses are 0.5 m apart, they will be moving towards each other so they will have kinetic and gravitational potential energy. According to the law of conservation of energy, the net energy of the system remains constant. Also, since the net momentum of the two masses is zero when they are at rest, the net momentum will also be zero when they are moving towards each other. So, we can write
$\Rightarrow {M_1}{v_1} = {M_2}{v_2}$
Placing the value of two masses, we get
$\Rightarrow 10{v_1} = 20{v_2}$
$\Rightarrow {v_1} = 2{v_2}$
So, the net energy of the system in the case when they at 1m distance will be due to gravitational potential energy and will be:
$\Rightarrow {E_{initial}} = - \dfrac{{G{M_1}{M_2}}}{{{r_1}}}$ where ${M_1} = 10\,kg$ and ${M_2} = 20\,kg$, ${r_1} = 1\,m$
When they are 0.5 m apart, the energy of the system will be due to kinetic and potential energy and can be written as:
$\Rightarrow {E_{final}} = - \dfrac{{G{M_1}{M_2}}}{{{r_2}}} + \dfrac{1}{2}{M_1}{v_1}^2 + \dfrac{1}{2}{M_2}{v_2}^2$ where ${r_2} = 0.5\,m$
Since ${E_{initial}} = {E_{final}}$ from the law of conservation of energy, we have
$\Rightarrow - \dfrac{{G{M_1}{M_2}}}{{{r_1}}} = - \dfrac{{G{M_1}{M_2}}}{{{r_2}}} + \dfrac{1}{2}{M_1}{v_1}^2 + \dfrac{1}{2}{M_2}{v_2}^2$
Placing ${v_1} = 2{v_2}$ and $G = 6.67{\text{ }} \times {\text{ }}{10^ - }^{11}\;N/k{g^2}\;{m^2}$ in the above equation, we get
$\Rightarrow - \dfrac{{6.67 \times {{10}^{ - 11}} \times 10 \times 20}}{1} = - \dfrac{{6.67 \times {{10}^{ - 11}} \times 10 \times 20}}{{0.5}} + 20{v_2}^2 + 10{v_2}^2$
Simplifying the above equation, we get
$\Rightarrow - 13.34 \times {10^{ - 9}} = - 26.68 \times {10^{ - 9}} + 30{v_2}^2$
$\Rightarrow 13.34 \times {10^{ - 9}} = \,30{v_2}^2$
Solving for ${v_2}$, we get
$\Rightarrow {v_2} = 2.1 \times {10^{ - 5}}m/s$
Since ${v_1} = 2{v_2}$, we have
$\Rightarrow {v_1} = 4.2 \times {10^{ - 5}}\,m/s$.
Note
The trick to solving such a question is realizing that we only have to use the law of conservation of energy in the initial and the final point and not in between. Since the velocity of the two objects in question is very negligible, it shows why normal day to day objects don’t attract each other since the gravitational acceleration due to Earth which has a much higher mass overpowers the gravitational acceleration between objects.
Complete step by step answer
We’ve been given that two bodies of mass 10 kg and 20 kg are kept at a distance of 1 m. At this point, the system of two masses will have only gravitational potential and no kinetic energy since they are not moving.
When the two masses are 0.5 m apart, they will be moving towards each other so they will have kinetic and gravitational potential energy. According to the law of conservation of energy, the net energy of the system remains constant. Also, since the net momentum of the two masses is zero when they are at rest, the net momentum will also be zero when they are moving towards each other. So, we can write
$\Rightarrow {M_1}{v_1} = {M_2}{v_2}$
Placing the value of two masses, we get
$\Rightarrow 10{v_1} = 20{v_2}$
$\Rightarrow {v_1} = 2{v_2}$
So, the net energy of the system in the case when they at 1m distance will be due to gravitational potential energy and will be:
$\Rightarrow {E_{initial}} = - \dfrac{{G{M_1}{M_2}}}{{{r_1}}}$ where ${M_1} = 10\,kg$ and ${M_2} = 20\,kg$, ${r_1} = 1\,m$
When they are 0.5 m apart, the energy of the system will be due to kinetic and potential energy and can be written as:
$\Rightarrow {E_{final}} = - \dfrac{{G{M_1}{M_2}}}{{{r_2}}} + \dfrac{1}{2}{M_1}{v_1}^2 + \dfrac{1}{2}{M_2}{v_2}^2$ where ${r_2} = 0.5\,m$
Since ${E_{initial}} = {E_{final}}$ from the law of conservation of energy, we have
$\Rightarrow - \dfrac{{G{M_1}{M_2}}}{{{r_1}}} = - \dfrac{{G{M_1}{M_2}}}{{{r_2}}} + \dfrac{1}{2}{M_1}{v_1}^2 + \dfrac{1}{2}{M_2}{v_2}^2$
Placing ${v_1} = 2{v_2}$ and $G = 6.67{\text{ }} \times {\text{ }}{10^ - }^{11}\;N/k{g^2}\;{m^2}$ in the above equation, we get
$\Rightarrow - \dfrac{{6.67 \times {{10}^{ - 11}} \times 10 \times 20}}{1} = - \dfrac{{6.67 \times {{10}^{ - 11}} \times 10 \times 20}}{{0.5}} + 20{v_2}^2 + 10{v_2}^2$
Simplifying the above equation, we get
$\Rightarrow - 13.34 \times {10^{ - 9}} = - 26.68 \times {10^{ - 9}} + 30{v_2}^2$
$\Rightarrow 13.34 \times {10^{ - 9}} = \,30{v_2}^2$
Solving for ${v_2}$, we get
$\Rightarrow {v_2} = 2.1 \times {10^{ - 5}}m/s$
Since ${v_1} = 2{v_2}$, we have
$\Rightarrow {v_1} = 4.2 \times {10^{ - 5}}\,m/s$.
Note
The trick to solving such a question is realizing that we only have to use the law of conservation of energy in the initial and the final point and not in between. Since the velocity of the two objects in question is very negligible, it shows why normal day to day objects don’t attract each other since the gravitational acceleration due to Earth which has a much higher mass overpowers the gravitational acceleration between objects.
Recently Updated Pages
How many sigma and pi bonds are present in HCequiv class 11 chemistry CBSE
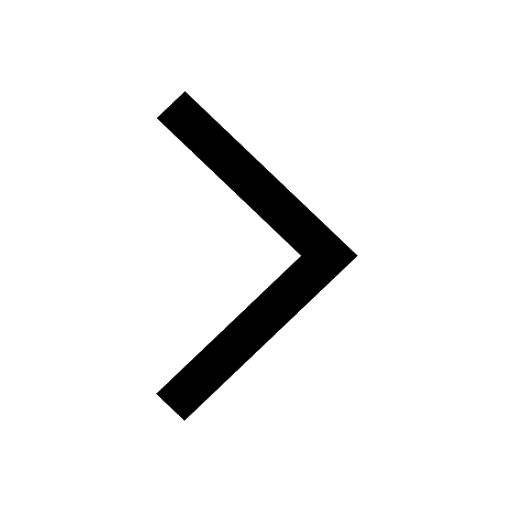
Why Are Noble Gases NonReactive class 11 chemistry CBSE
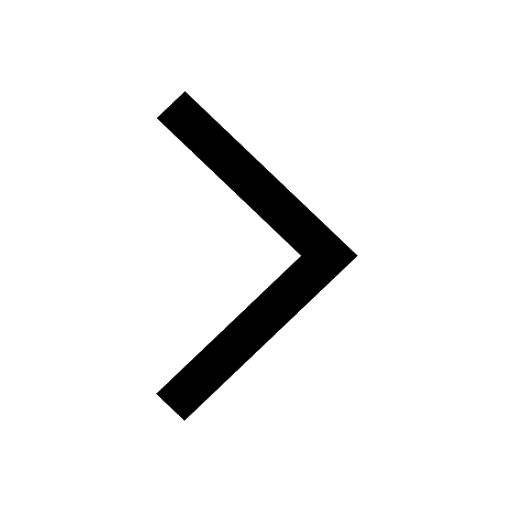
Let X and Y be the sets of all positive divisors of class 11 maths CBSE
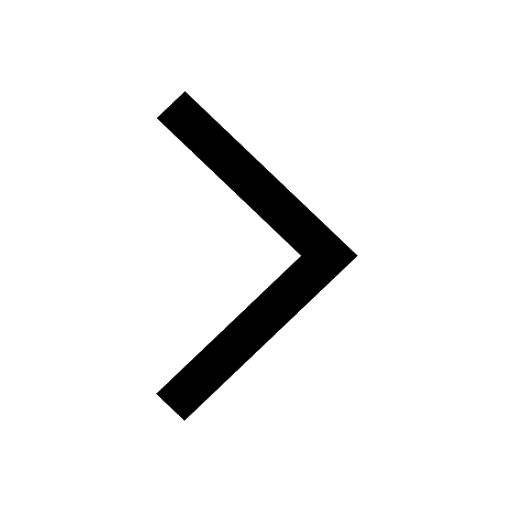
Let x and y be 2 real numbers which satisfy the equations class 11 maths CBSE
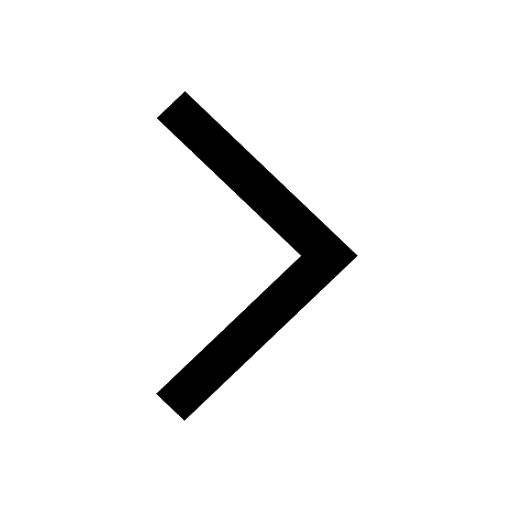
Let x 4log 2sqrt 9k 1 + 7 and y dfrac132log 2sqrt5 class 11 maths CBSE
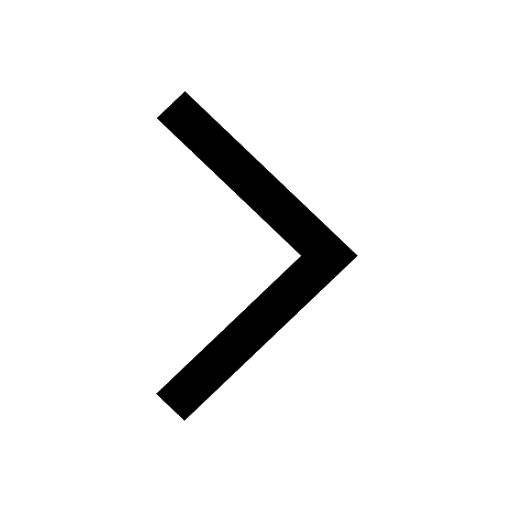
Let x22ax+b20 and x22bx+a20 be two equations Then the class 11 maths CBSE
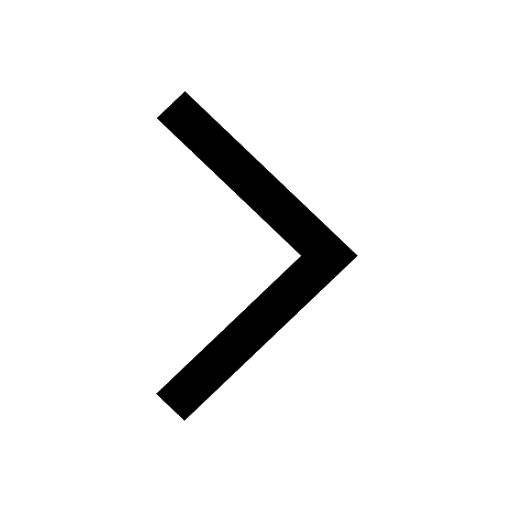
Trending doubts
Fill the blanks with the suitable prepositions 1 The class 9 english CBSE
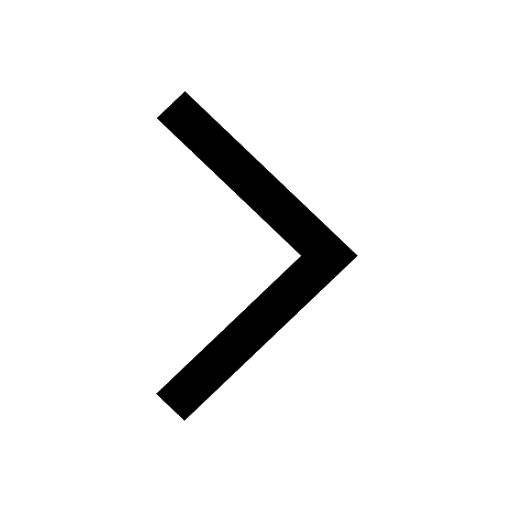
At which age domestication of animals started A Neolithic class 11 social science CBSE
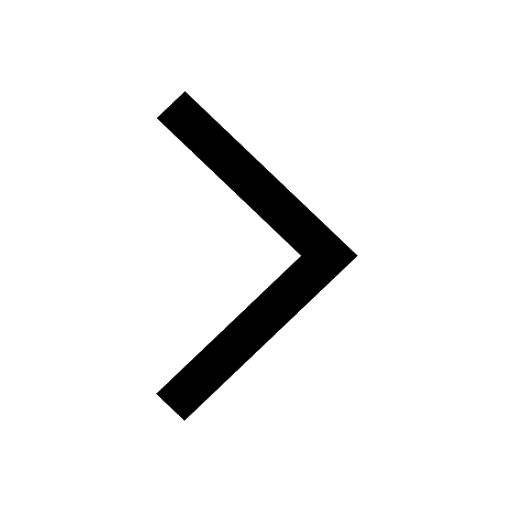
Which are the Top 10 Largest Countries of the World?
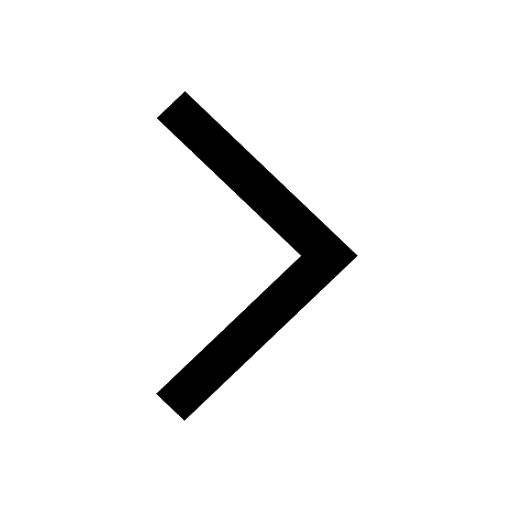
Give 10 examples for herbs , shrubs , climbers , creepers
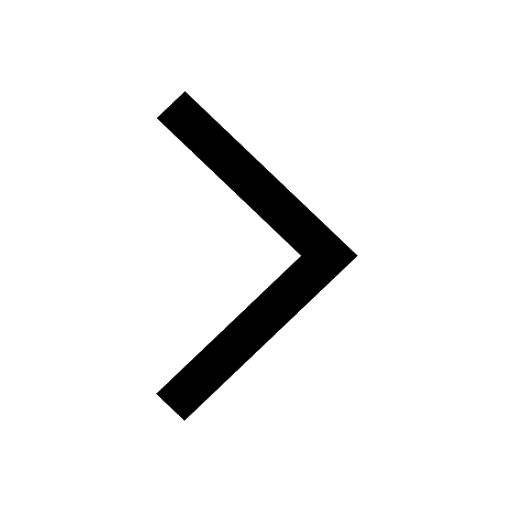
Difference between Prokaryotic cell and Eukaryotic class 11 biology CBSE
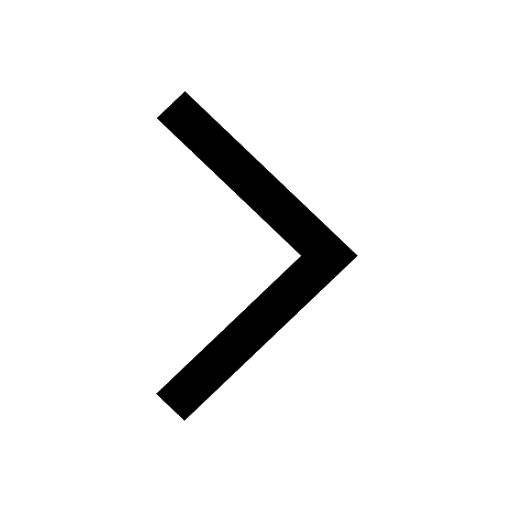
Difference Between Plant Cell and Animal Cell
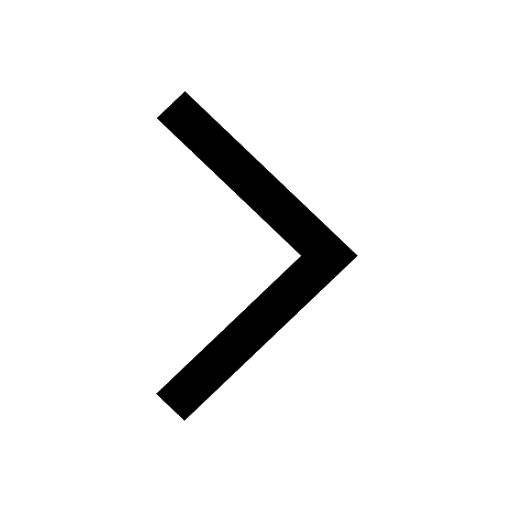
Write a letter to the principal requesting him to grant class 10 english CBSE
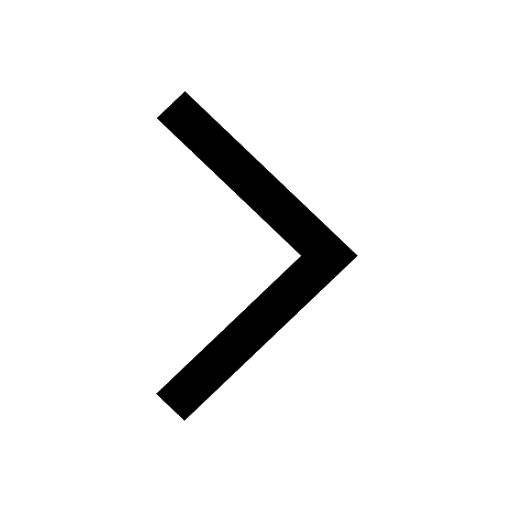
Change the following sentences into negative and interrogative class 10 english CBSE
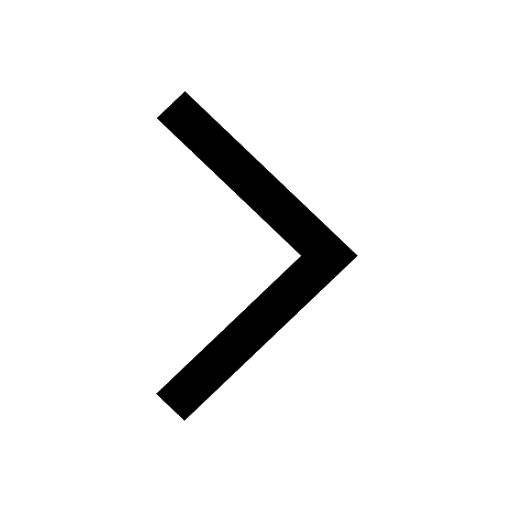
Fill in the blanks A 1 lakh ten thousand B 1 million class 9 maths CBSE
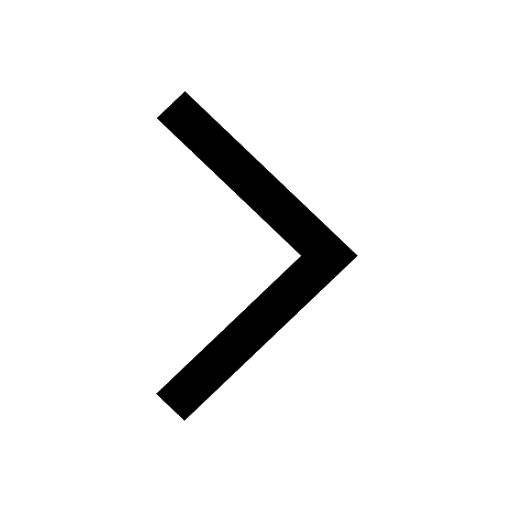