
Answer
376.8k+ views
Hint: First, we must reduce the expression ${}^{2}{{C}_{2}}+{}^{3}{{C}_{2}}+{}^{4}{{C}_{2}}+...+{}^{n}{{C}_{2}}$ by using the property ${}^{n}{{C}_{r}}+{}^{n}{{C}_{r+1}}={}^{n+1}{{C}_{r+1}}$ and the fact that ${}^{n}{{C}_{n}}=1$. Then, we can evaluate the given equation using the definition ${}^{n}{{C}_{r}}=\dfrac{n!}{r!\left( n-r \right)!}$. By comparing, we can find the value of k, m, p and h.
Complete step-by-step solution:
We all know very well that the combination of r distinct objects from a set of n distinct objects is defined as ${}^{n}{{C}_{r}}=\dfrac{n!}{r!\left( n-r \right)!}$.
For r = n, we can write that ${}^{n}{{C}_{n}}=\dfrac{n!}{n!\left( n-n \right)!}$.
Hence, we get ${}^{n}{{C}_{n}}=\dfrac{1}{0!}$.
We know that the factorial of 0 is defined as 1. Thus, we can write that
${}^{n}{{C}_{n}}=1$.
Thus, we now have ${}^{2}{{C}_{2}}={}^{3}{{C}_{3}}=1$.
Hence, we can write
${}^{2}{{C}_{2}}+{}^{3}{{C}_{2}}+{}^{4}{{C}_{2}}+...+{}^{n}{{C}_{2}}={}^{3}{{C}_{3}}+{}^{3}{{C}_{2}}+{}^{4}{{C}_{2}}+...+{}^{n}{{C}_{2}}...\left( i \right)$
We know the property that
${}^{n}{{C}_{r}}+{}^{n}{{C}_{r+1}}={}^{n+1}{{C}_{r+1}}$.
Hence, we can say that ${}^{3}{{C}_{2}}+{}^{3}{{C}_{3}}={}^{4}{{C}_{3}}$.
Thus, using the above value on the right hand side of equation (i), we get
${}^{2}{{C}_{2}}+{}^{3}{{C}_{2}}+{}^{4}{{C}_{2}}+...+{}^{n}{{C}_{2}}={}^{4}{{C}_{3}}+{}^{4}{{C}_{2}}+...+{}^{n}{{C}_{2}}...\left( ii \right)$
Using the same property again, we can write ${}^{4}{{C}_{2}}+{}^{4}{{C}_{3}}={}^{5}{{C}_{3}}$.
Thus, using the above value on the right hand side of equation (ii), we get
${}^{2}{{C}_{2}}+{}^{3}{{C}_{2}}+{}^{4}{{C}_{2}}+...+{}^{n}{{C}_{2}}={}^{5}{{C}_{3}}+{}^{5}{{C}_{2}}+...+{}^{n}{{C}_{2}}$
We can reduce the above equation in the same way, to get
${}^{2}{{C}_{2}}+{}^{3}{{C}_{2}}+{}^{4}{{C}_{2}}+...+{}^{n}{{C}_{2}}={}^{n}{{C}_{3}}+{}^{n}{{C}_{2}}$
And hence, we get
${}^{2}{{C}_{2}}+{}^{3}{{C}_{2}}+{}^{4}{{C}_{2}}+...+{}^{n}{{C}_{2}}={}^{n+1}{{C}_{3}}$.
So, we can write the given equation as
${}^{n+1}{{C}_{2}}+2\left[ {}^{n+1}{{C}_{3}} \right]=\dfrac{n\left( n+k \right)\left( pn+m \right)}{h}$
We can write the above equation as
${}^{n+1}{{C}_{2}}+{}^{n+1}{{C}_{3}}+{}^{n+1}{{C}_{3}}=\dfrac{n\left( n+k \right)\left( pn+m \right)}{h}$
Using the property ${}^{n}{{C}_{r}}+{}^{n}{{C}_{r+1}}={}^{n+1}{{C}_{r+1}}$ on the left hand side of above equation, we can write
${}^{n+2}{{C}_{3}}+{}^{n+1}{{C}_{3}}=\dfrac{n\left( n+k \right)\left( pn+m \right)}{h}$.
Using the definition of combinations, we can write
$\dfrac{\left( n+2 \right)!}{3!\left( n+2-3 \right)!}+\dfrac{\left( n+1 \right)!}{3!\left( n+1-3 \right)!}=\dfrac{n\left( n+k \right)\left( pn+m \right)}{h}$.
On simplification, we get
$\dfrac{\left( n+2 \right)!}{3!\left( n-1 \right)!}+\dfrac{\left( n+1 \right)!}{3!\left( n-2 \right)!}=\dfrac{n\left( n+k \right)\left( pn+m \right)}{h}$.
Hence, we can now write
$\dfrac{\left( n+2 \right)\left( n+1 \right)n}{6}+\dfrac{\left( n+1 \right)n\left( n-1 \right)}{6}=\dfrac{n\left( n+k \right)\left( pn+m \right)}{h}$.
We can simplify this equation as
$\dfrac{n\left( n+1 \right)\left( 2n+1 \right)}{6}=\dfrac{n\left( n+k \right)\left( pn+m \right)}{h}$
On comparing, we can write
$\begin{align}
& k=1 \\
& m=1 \\
& p=2 \\
& h=6 \\
\end{align}$
Thus, $k+m+p+h=1+1+2+6$.
Hence, the value of $k+m+p+h$ is 10.
Note: We must remember the property of combination, ${}^{n}{{C}_{r}}+{}^{n}{{C}_{r+1}}={}^{n+1}{{C}_{r+1}}$ by heart, as it is very easy to make a mistake in writing this property. Also, we must not try to simplify the given equation by solving each combination separately, as this will make the equation much more complex.
Complete step-by-step solution:
We all know very well that the combination of r distinct objects from a set of n distinct objects is defined as ${}^{n}{{C}_{r}}=\dfrac{n!}{r!\left( n-r \right)!}$.
For r = n, we can write that ${}^{n}{{C}_{n}}=\dfrac{n!}{n!\left( n-n \right)!}$.
Hence, we get ${}^{n}{{C}_{n}}=\dfrac{1}{0!}$.
We know that the factorial of 0 is defined as 1. Thus, we can write that
${}^{n}{{C}_{n}}=1$.
Thus, we now have ${}^{2}{{C}_{2}}={}^{3}{{C}_{3}}=1$.
Hence, we can write
${}^{2}{{C}_{2}}+{}^{3}{{C}_{2}}+{}^{4}{{C}_{2}}+...+{}^{n}{{C}_{2}}={}^{3}{{C}_{3}}+{}^{3}{{C}_{2}}+{}^{4}{{C}_{2}}+...+{}^{n}{{C}_{2}}...\left( i \right)$
We know the property that
${}^{n}{{C}_{r}}+{}^{n}{{C}_{r+1}}={}^{n+1}{{C}_{r+1}}$.
Hence, we can say that ${}^{3}{{C}_{2}}+{}^{3}{{C}_{3}}={}^{4}{{C}_{3}}$.
Thus, using the above value on the right hand side of equation (i), we get
${}^{2}{{C}_{2}}+{}^{3}{{C}_{2}}+{}^{4}{{C}_{2}}+...+{}^{n}{{C}_{2}}={}^{4}{{C}_{3}}+{}^{4}{{C}_{2}}+...+{}^{n}{{C}_{2}}...\left( ii \right)$
Using the same property again, we can write ${}^{4}{{C}_{2}}+{}^{4}{{C}_{3}}={}^{5}{{C}_{3}}$.
Thus, using the above value on the right hand side of equation (ii), we get
${}^{2}{{C}_{2}}+{}^{3}{{C}_{2}}+{}^{4}{{C}_{2}}+...+{}^{n}{{C}_{2}}={}^{5}{{C}_{3}}+{}^{5}{{C}_{2}}+...+{}^{n}{{C}_{2}}$
We can reduce the above equation in the same way, to get
${}^{2}{{C}_{2}}+{}^{3}{{C}_{2}}+{}^{4}{{C}_{2}}+...+{}^{n}{{C}_{2}}={}^{n}{{C}_{3}}+{}^{n}{{C}_{2}}$
And hence, we get
${}^{2}{{C}_{2}}+{}^{3}{{C}_{2}}+{}^{4}{{C}_{2}}+...+{}^{n}{{C}_{2}}={}^{n+1}{{C}_{3}}$.
So, we can write the given equation as
${}^{n+1}{{C}_{2}}+2\left[ {}^{n+1}{{C}_{3}} \right]=\dfrac{n\left( n+k \right)\left( pn+m \right)}{h}$
We can write the above equation as
${}^{n+1}{{C}_{2}}+{}^{n+1}{{C}_{3}}+{}^{n+1}{{C}_{3}}=\dfrac{n\left( n+k \right)\left( pn+m \right)}{h}$
Using the property ${}^{n}{{C}_{r}}+{}^{n}{{C}_{r+1}}={}^{n+1}{{C}_{r+1}}$ on the left hand side of above equation, we can write
${}^{n+2}{{C}_{3}}+{}^{n+1}{{C}_{3}}=\dfrac{n\left( n+k \right)\left( pn+m \right)}{h}$.
Using the definition of combinations, we can write
$\dfrac{\left( n+2 \right)!}{3!\left( n+2-3 \right)!}+\dfrac{\left( n+1 \right)!}{3!\left( n+1-3 \right)!}=\dfrac{n\left( n+k \right)\left( pn+m \right)}{h}$.
On simplification, we get
$\dfrac{\left( n+2 \right)!}{3!\left( n-1 \right)!}+\dfrac{\left( n+1 \right)!}{3!\left( n-2 \right)!}=\dfrac{n\left( n+k \right)\left( pn+m \right)}{h}$.
Hence, we can now write
$\dfrac{\left( n+2 \right)\left( n+1 \right)n}{6}+\dfrac{\left( n+1 \right)n\left( n-1 \right)}{6}=\dfrac{n\left( n+k \right)\left( pn+m \right)}{h}$.
We can simplify this equation as
$\dfrac{n\left( n+1 \right)\left( 2n+1 \right)}{6}=\dfrac{n\left( n+k \right)\left( pn+m \right)}{h}$
On comparing, we can write
$\begin{align}
& k=1 \\
& m=1 \\
& p=2 \\
& h=6 \\
\end{align}$
Thus, $k+m+p+h=1+1+2+6$.
Hence, the value of $k+m+p+h$ is 10.
Note: We must remember the property of combination, ${}^{n}{{C}_{r}}+{}^{n}{{C}_{r+1}}={}^{n+1}{{C}_{r+1}}$ by heart, as it is very easy to make a mistake in writing this property. Also, we must not try to simplify the given equation by solving each combination separately, as this will make the equation much more complex.
Recently Updated Pages
How many sigma and pi bonds are present in HCequiv class 11 chemistry CBSE
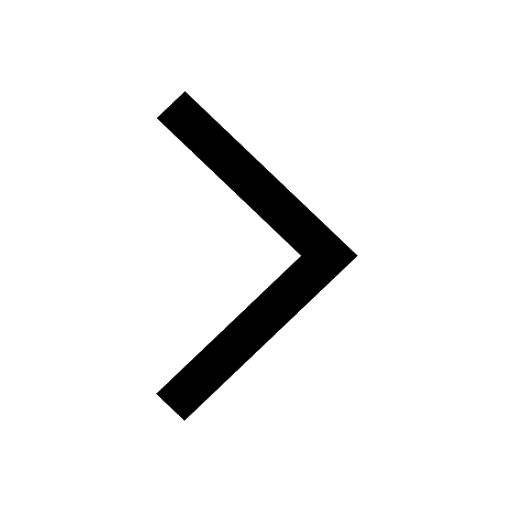
Mark and label the given geoinformation on the outline class 11 social science CBSE
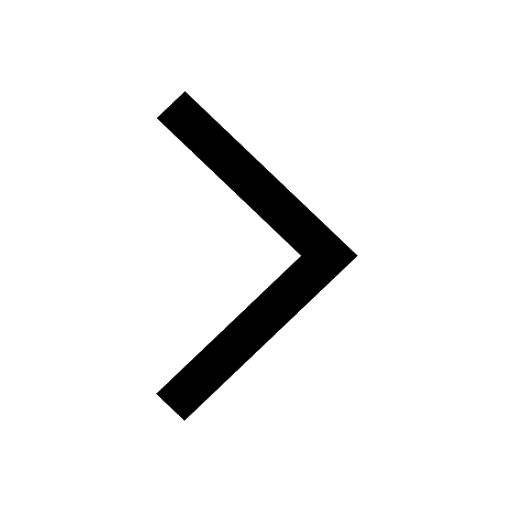
When people say No pun intended what does that mea class 8 english CBSE
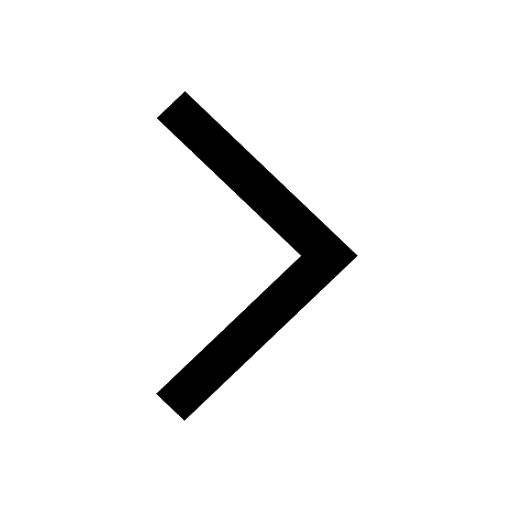
Name the states which share their boundary with Indias class 9 social science CBSE
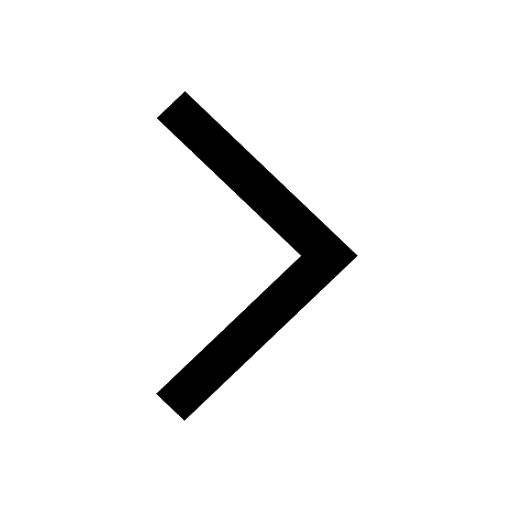
Give an account of the Northern Plains of India class 9 social science CBSE
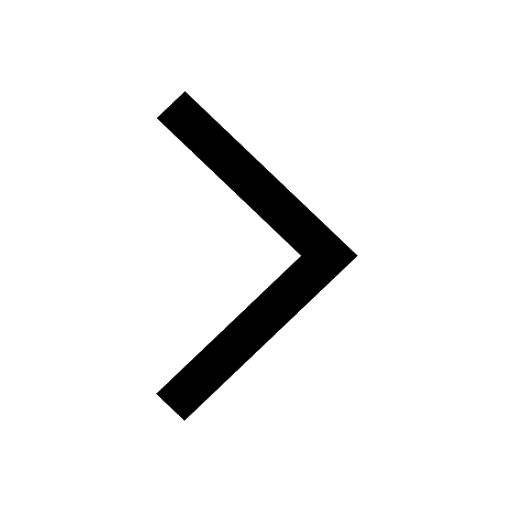
Change the following sentences into negative and interrogative class 10 english CBSE
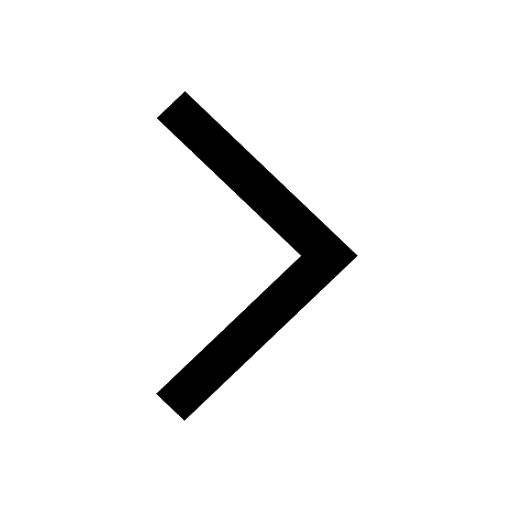
Trending doubts
Fill the blanks with the suitable prepositions 1 The class 9 english CBSE
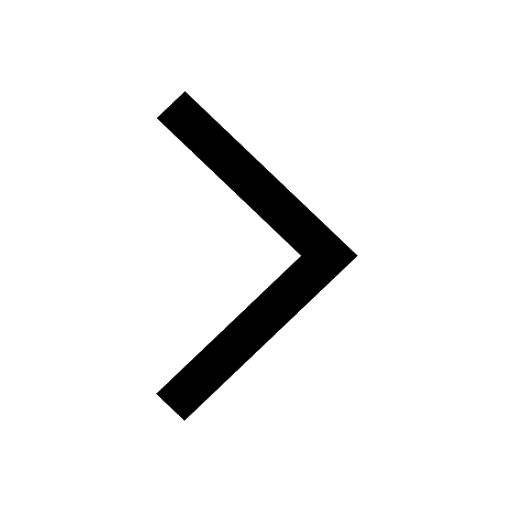
The Equation xxx + 2 is Satisfied when x is Equal to Class 10 Maths
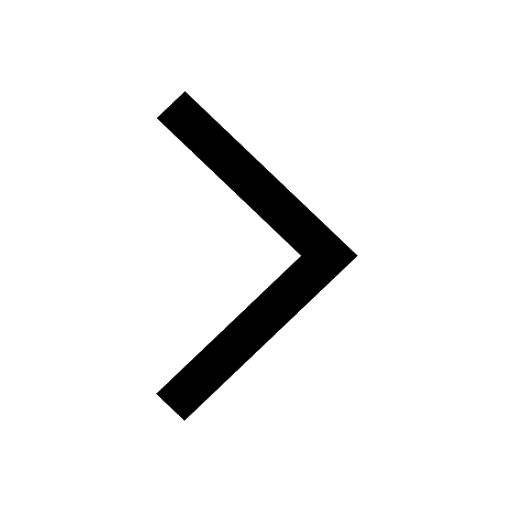
In Indian rupees 1 trillion is equal to how many c class 8 maths CBSE
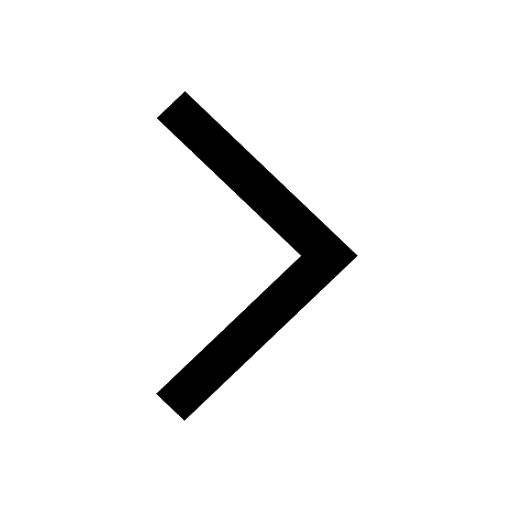
Which are the Top 10 Largest Countries of the World?
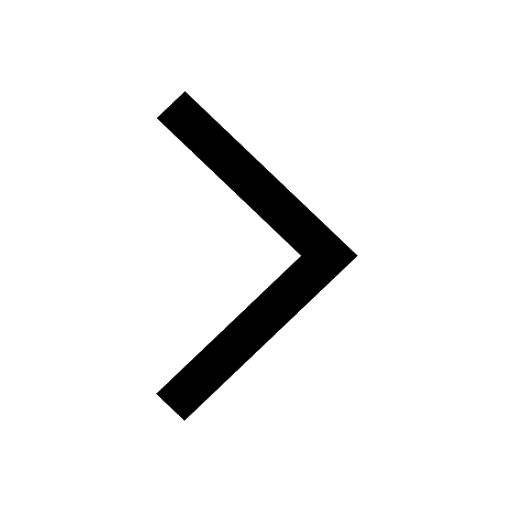
How do you graph the function fx 4x class 9 maths CBSE
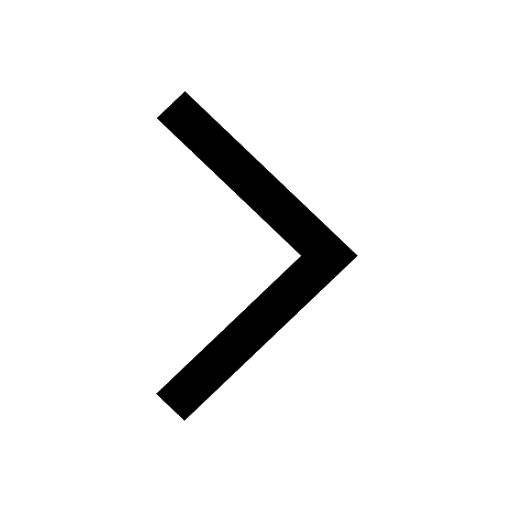
Give 10 examples for herbs , shrubs , climbers , creepers
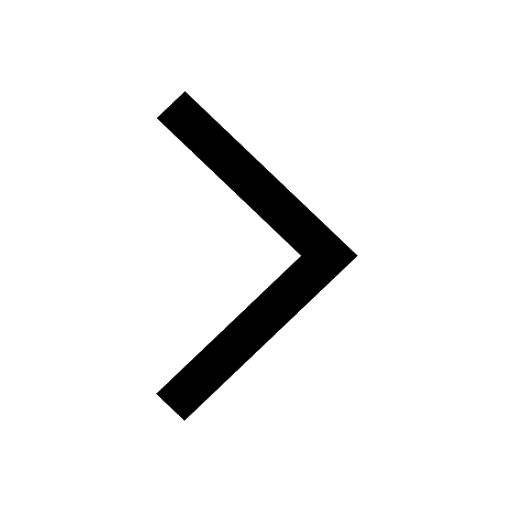
Difference Between Plant Cell and Animal Cell
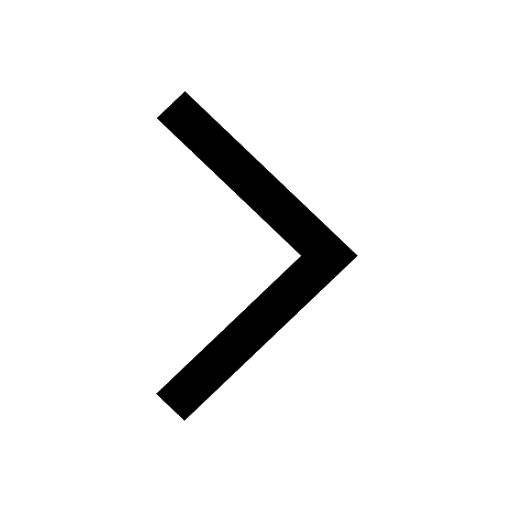
Difference between Prokaryotic cell and Eukaryotic class 11 biology CBSE
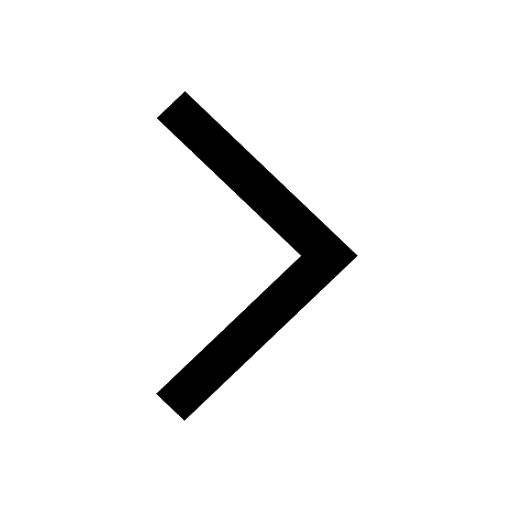
Why is there a time difference of about 5 hours between class 10 social science CBSE
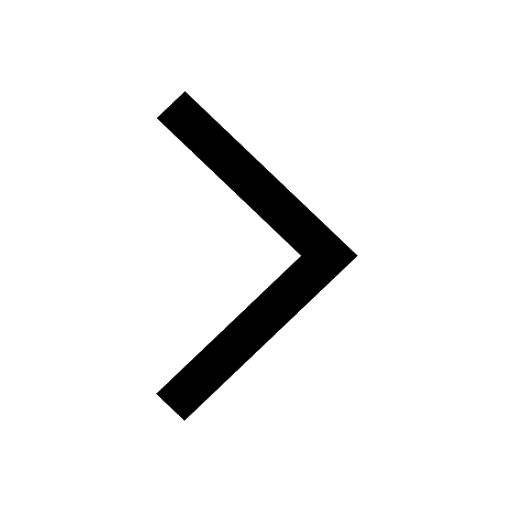