Answer
385.8k+ views
Hint: From the figure we can say that the current I get equally divided between AB and AC. With the help of these currents find the potential difference across AB and AC. From the potential difference across AB and AC you will get the potential difference and the current flowing across BC. Then, use the formula for magnetic field and find the magnetic field due to AC, AB and BC. Adding the magnetic fields due to AB, AC and BC will give the net magnetic field. This obtained value will be the magnetic field at the center O.
Complete answer:
Let the radius of the circular loop be r
It is given that the current entering from A is I.
The circuit is symmetric so the current flowing from A to B will be equal to the current flowing from A to C.
Current from A to B= Current from A to C= $\dfrac {I}{2}$
Let the potential at A be ${V}_{A}$
Similarly, at B and C be ${V}_{B}$ and ${V}_{C}$ respectively.
The potential difference between A and B can be given by,
${V}_{B}-{V}_{A}= \dfrac {I}{2}R$
$\Rightarrow {V}_{B}= {V}_{A}+ \dfrac {I}{2}R$ …(1)
Similarly, the potential difference between A and C can be given by,
${V}_{C}-{V}_{A}= \dfrac {I}{2}R$
$\Rightarrow {V}_{C}= {V}_{A}+ \dfrac {I}{2}R$ …(2)
From the equation. (1) and (2) we get,
${V}_{B}= {V}_{C}$
Thus, as the potential at B and C are the same, no current will flow from B to C.
We know, Magnetic field is given by,
$B= \dfrac {\theta}{360°}. \dfrac {{\mu}_{0}I}{2\pi r}$
Magnetic field due to AC by substituting the values in above equation is given by,
${B}_{AC}= \dfrac {120°}{360°}. \dfrac {{\mu}_{0}I}{4\pi r}$
$\Rightarrow {B}_{AC}= \dfrac {1}{3}. \dfrac {{\mu}_{0}I}{4\pi r}$
Now, applying the right hand thumb rule, the direction of the magnetic field is outwards.
Similarly, Magnetic field due to AB is given by,
${B}_{AB}= \dfrac {120°}{360°}. \dfrac {{\mu}_{0}I}{4\pi r}$
$\Rightarrow {B}_{AB}= \dfrac {1}{3}. \dfrac {{\mu}_{0}I}{4\pi r}$
Applying right hand thumb rule, the magnetic field due to AB is inwards.
Similarly, Magnetic field due to BC is given by,
${B}_{BC}= \dfrac {120°}{360°}. \dfrac {{\mu}_{0} \times 0}{2\pi r}$
$\Rightarrow {B}_{BC}= 0$
The magnitude of the magnetic field due to AB and AC are the same but their direction is opposite.
$\Rightarrow {B}_{AC}= -{B}_{AB}$ …(3)
Thus, the net magnetic field is given by,
${B}_{net}={B}_{AC}+{B}_{AB}+{B}_{BC}$
Substituting equation. (3) and value of ${B}_{BC}$ in above equation we get,
${B}_{net}=-{B}_{AB}+{B}_{AB}+0$
$\Rightarrow {B}_{net}= 0$
Thus, the magnetic field at the center O is zero.
So, the correct answer is option D i.e. zero.
Note:
For solving this question, we have used the right hand thumb rule. Students should not get confused between the right-hand thumb rule and Fleming’s right hand rule. According to Fleming’s right hand rule, the thumb indicates the direction of force, the forefinger indicates the direction of the magnetic field and the middle finger represents the direction of current. Whereas according to right hand thumb rule, the thumb indicates the direction of current and the direction in which the fingers wrap gives the direction of magnetic field.
Complete answer:
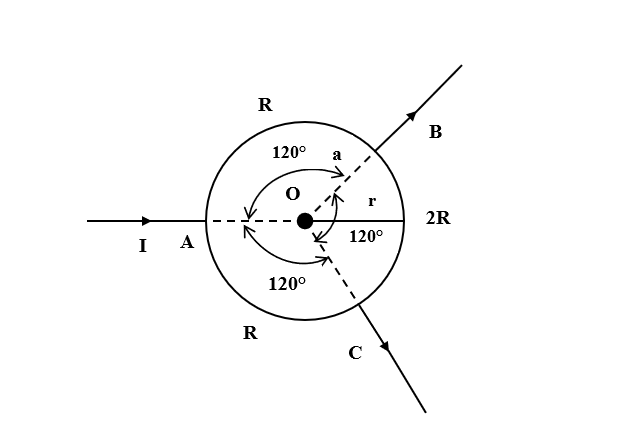
Let the radius of the circular loop be r
It is given that the current entering from A is I.
The circuit is symmetric so the current flowing from A to B will be equal to the current flowing from A to C.
Current from A to B= Current from A to C= $\dfrac {I}{2}$
Let the potential at A be ${V}_{A}$
Similarly, at B and C be ${V}_{B}$ and ${V}_{C}$ respectively.
The potential difference between A and B can be given by,
${V}_{B}-{V}_{A}= \dfrac {I}{2}R$
$\Rightarrow {V}_{B}= {V}_{A}+ \dfrac {I}{2}R$ …(1)
Similarly, the potential difference between A and C can be given by,
${V}_{C}-{V}_{A}= \dfrac {I}{2}R$
$\Rightarrow {V}_{C}= {V}_{A}+ \dfrac {I}{2}R$ …(2)
From the equation. (1) and (2) we get,
${V}_{B}= {V}_{C}$
Thus, as the potential at B and C are the same, no current will flow from B to C.
We know, Magnetic field is given by,
$B= \dfrac {\theta}{360°}. \dfrac {{\mu}_{0}I}{2\pi r}$
Magnetic field due to AC by substituting the values in above equation is given by,
${B}_{AC}= \dfrac {120°}{360°}. \dfrac {{\mu}_{0}I}{4\pi r}$
$\Rightarrow {B}_{AC}= \dfrac {1}{3}. \dfrac {{\mu}_{0}I}{4\pi r}$
Now, applying the right hand thumb rule, the direction of the magnetic field is outwards.
Similarly, Magnetic field due to AB is given by,
${B}_{AB}= \dfrac {120°}{360°}. \dfrac {{\mu}_{0}I}{4\pi r}$
$\Rightarrow {B}_{AB}= \dfrac {1}{3}. \dfrac {{\mu}_{0}I}{4\pi r}$
Applying right hand thumb rule, the magnetic field due to AB is inwards.
Similarly, Magnetic field due to BC is given by,
${B}_{BC}= \dfrac {120°}{360°}. \dfrac {{\mu}_{0} \times 0}{2\pi r}$
$\Rightarrow {B}_{BC}= 0$
The magnitude of the magnetic field due to AB and AC are the same but their direction is opposite.
$\Rightarrow {B}_{AC}= -{B}_{AB}$ …(3)
Thus, the net magnetic field is given by,
${B}_{net}={B}_{AC}+{B}_{AB}+{B}_{BC}$
Substituting equation. (3) and value of ${B}_{BC}$ in above equation we get,
${B}_{net}=-{B}_{AB}+{B}_{AB}+0$
$\Rightarrow {B}_{net}= 0$
Thus, the magnetic field at the center O is zero.
So, the correct answer is option D i.e. zero.
Note:
For solving this question, we have used the right hand thumb rule. Students should not get confused between the right-hand thumb rule and Fleming’s right hand rule. According to Fleming’s right hand rule, the thumb indicates the direction of force, the forefinger indicates the direction of the magnetic field and the middle finger represents the direction of current. Whereas according to right hand thumb rule, the thumb indicates the direction of current and the direction in which the fingers wrap gives the direction of magnetic field.
Recently Updated Pages
How many sigma and pi bonds are present in HCequiv class 11 chemistry CBSE
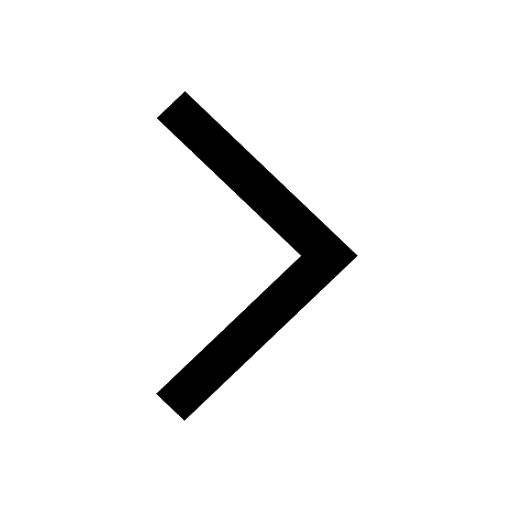
Why Are Noble Gases NonReactive class 11 chemistry CBSE
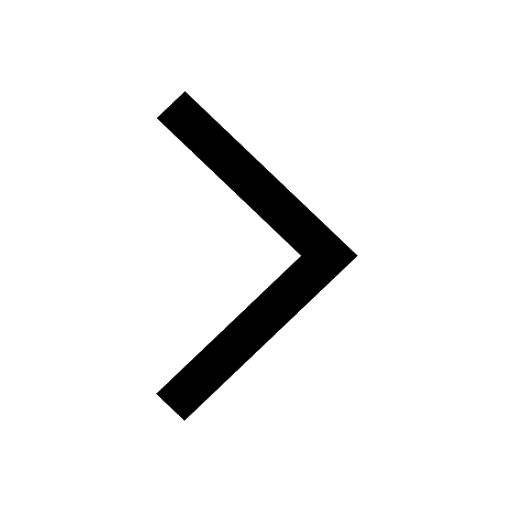
Let X and Y be the sets of all positive divisors of class 11 maths CBSE
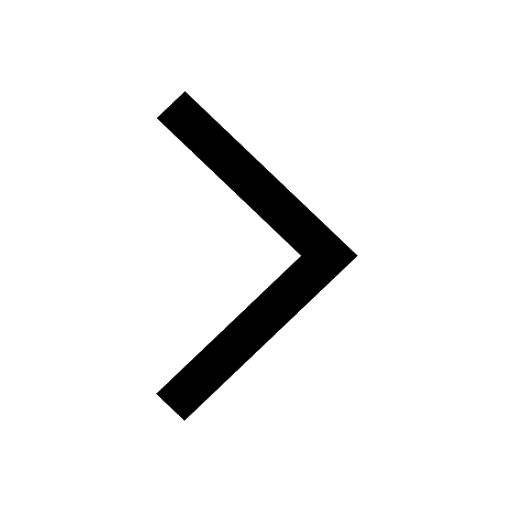
Let x and y be 2 real numbers which satisfy the equations class 11 maths CBSE
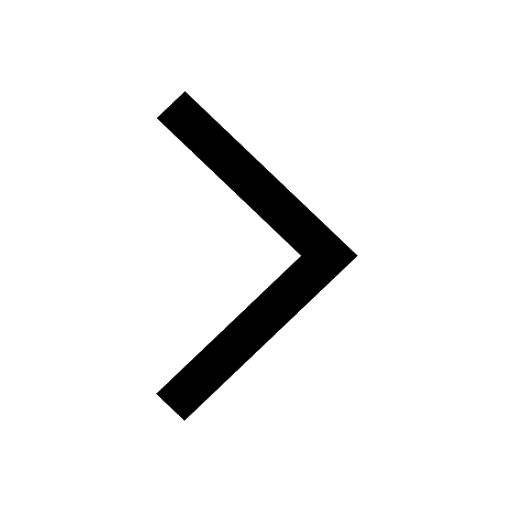
Let x 4log 2sqrt 9k 1 + 7 and y dfrac132log 2sqrt5 class 11 maths CBSE
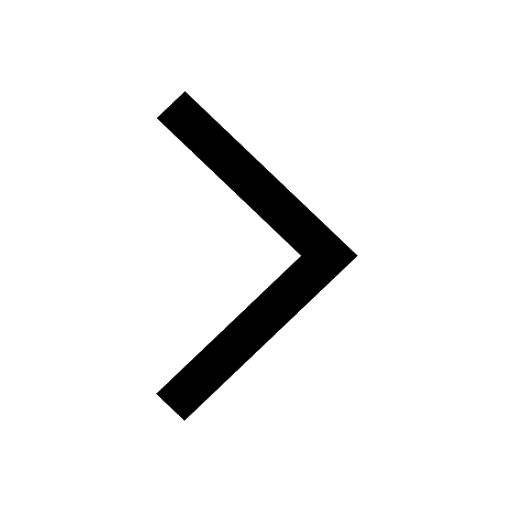
Let x22ax+b20 and x22bx+a20 be two equations Then the class 11 maths CBSE
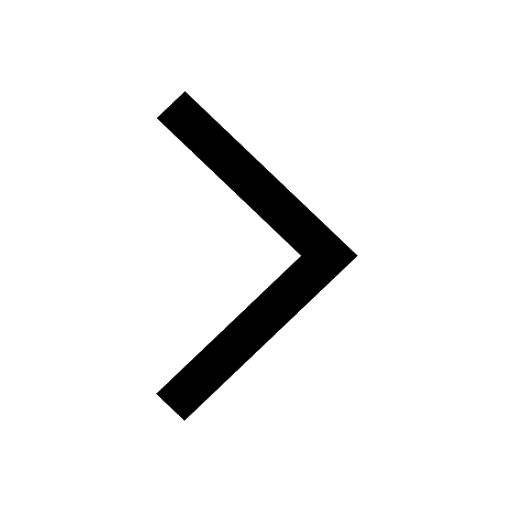
Trending doubts
Fill the blanks with the suitable prepositions 1 The class 9 english CBSE
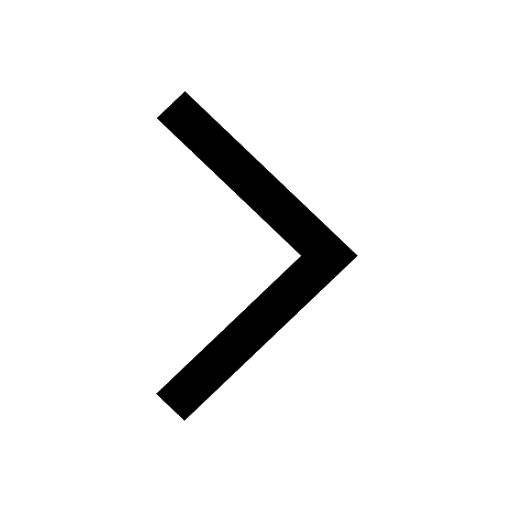
At which age domestication of animals started A Neolithic class 11 social science CBSE
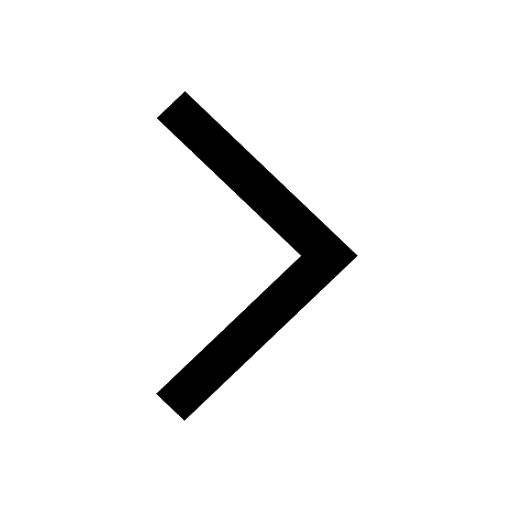
Which are the Top 10 Largest Countries of the World?
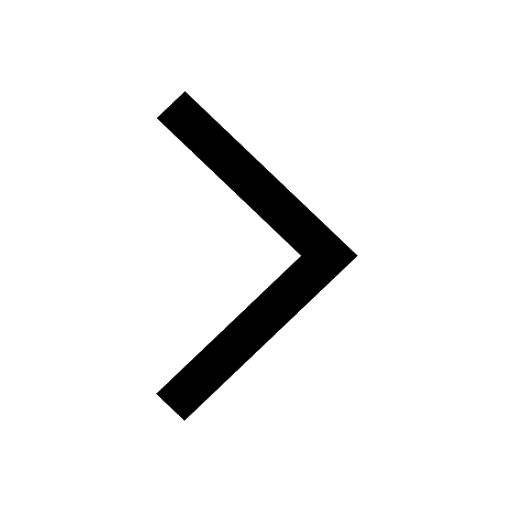
Give 10 examples for herbs , shrubs , climbers , creepers
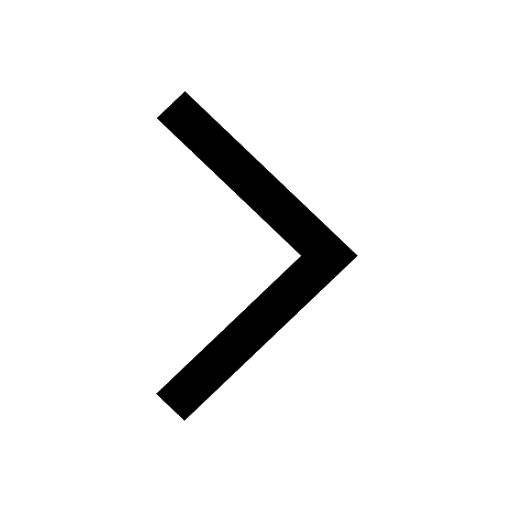
Difference between Prokaryotic cell and Eukaryotic class 11 biology CBSE
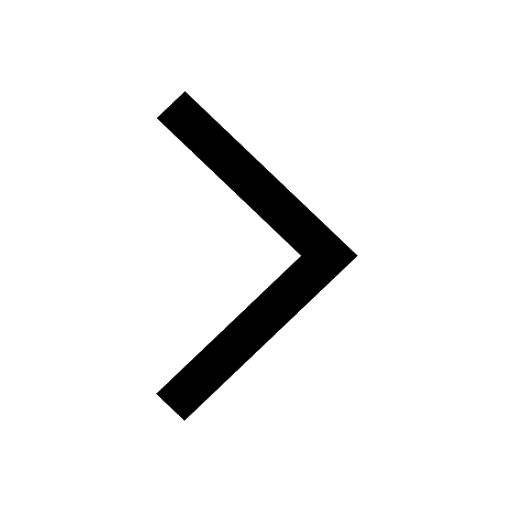
Difference Between Plant Cell and Animal Cell
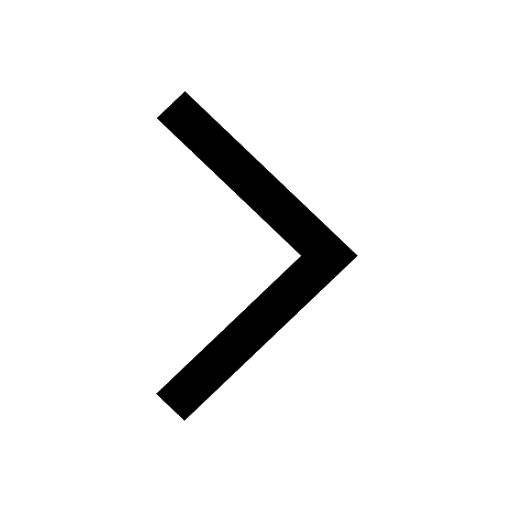
Write a letter to the principal requesting him to grant class 10 english CBSE
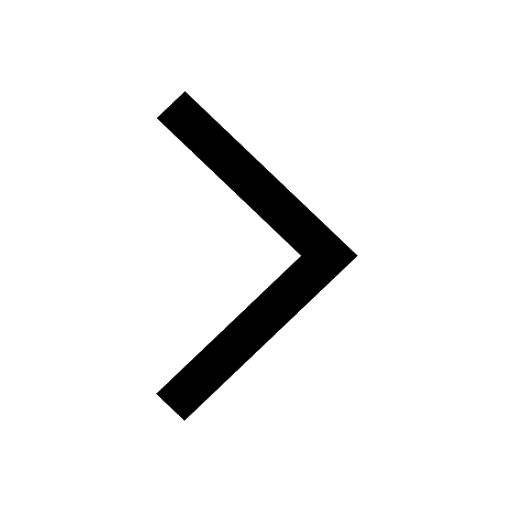
Change the following sentences into negative and interrogative class 10 english CBSE
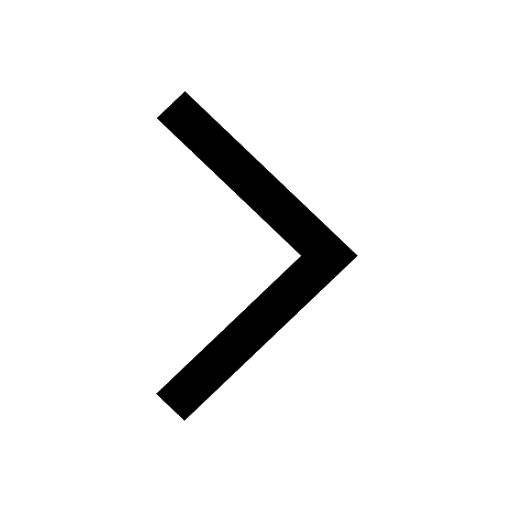
Fill in the blanks A 1 lakh ten thousand B 1 million class 9 maths CBSE
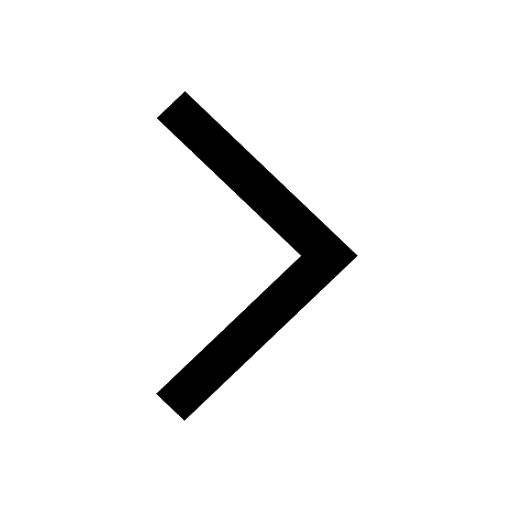