Answer
405k+ views
Hint:After establishing a relationship between the two variables of pressure and volume using the equation of a straight line pertaining to the graph, we will equate it with the ideal gas equation. Using the boundary conditions for maximum temperature, we will be able to determine the maximum temperature.
Formulae used:
Equation of a straight line: $(x - {x_0}) = \dfrac{{{x_2} - {x_1}}}{{{y_2} - {y_1}}}(y - {y_0})$
Where $y,{y_1},{y_2}$ and $x,{x_1},{x_2}$ are the y and x intercepts of a graph.
Ideal gas equation: $PV = nRT$
Where $P$ is the pressure of the gas and is expressed in Bars $(B)$, $V$ is the volume of the gas and is expressed in meter cube $({m^3})$, $n$ is the number of moles of the gas, $R$ is the universal gas constant and $T$ is the temperature of the gas and is expressed in Celsius $(^\circ C)$.
Complete step by step answer:
From the graph we have the following data:
At A:
Pressure $ = 2{P_0}$
Volume $ = {V_0}$
At B:
Pressure $ = {P_0}$
Volume $ = 2{V_0}$
To establish a relationship between the two, we will use the equation of a line for the given graph.
We know that, $(y - {y_0}) = \dfrac{{{y_2} - {y_1}}}{{{x_2} - {x_1}}}(x - {x_0})$.
Substituting the intercepts according to our graph we get,
$(V - {V_0}) = \dfrac{{{V_2} - {V_1}}}{{{P_2} - {P_1}}}(P - {P_0})$
According to the graph, \[{V_2} = 2{V_0},{V_1} = {V_0},{P_2} = {P_0},{P_1} = 2{P_0}\]. Substituting the same we get,
$(V - {V_0}) = \dfrac{{2{V_0} - {V_0}}}{{{P_0} - 2{P_0}}}(P - {P_0})$
Simplifying the above equation we get,
\[
(V - {V_0}) = \dfrac{{{V_0}}}{{ - {P_0}}}(P - {P_0}) \\
\Rightarrow (P - {P_0}) = (V - {V_0})\dfrac{{ - {P_0}}}{{{V_0}}} \\
\Rightarrow (P - {P_0}) = \dfrac{{ - {P_0}V}}{{{V_0}}} + 2{P_0} \\
\Rightarrow P = \dfrac{{ - {P_0}V}}{{{V_0}}} + 2{P_0} + {P_0} \\
\Rightarrow P = \dfrac{{ - {P_0}}}{{{V_0}}}(V - 3{V_0}) \\
\]
Now, we know that for an ideal gas $PV = nRT$
Rearranging this we get,
$P = \dfrac{{nRT}}{V}$
Substituting the above in the relationship established between volume and pressure we get,
$\dfrac{{nRT}}{V} = \dfrac{{ - {P_0}}}{{{V_0}}}(V - 3{V_0})$
Upon rearrangement to arrive at an expression for temperature $T$ we get,
$T = \dfrac{{ - {P_0}}}{{nR{V_0}}}({V^2} - 3{V_0}V)$
We need to determine the value of $V$.
Now, we know that at maximum temperature, rate of change of temperature with respect to volume of an ideal gas is constant. That is $\dfrac{{dT}}{{dV}} = 0$.
Therefore, differentiating the expression for temperature with respect to volume we get,
$\dfrac{{dT}}{{dV}} = \dfrac{{d(\dfrac{{ - {P_0}}}{{nR{V_0}}}({V^2} - 3{V_0}V))}}{{dV}}$
Upon simplification and substitution of boundary condition $\dfrac{{dT}}{{dV}} = 0$ we will establish a relationship between volume and pressure only,
$
\dfrac{{dT}}{{dV}} = \dfrac{{d(\dfrac{{ - {P_0}}}{{nR{V_0}}}({V^2} - 3{V_0}V))}}{{dV}} \\
\Rightarrow 0 = \dfrac{{d(\dfrac{{ - {P_0}}}{{nR{V_0}}}({V^2} - 3{V_0}V))}}{{dV}} \\
\Rightarrow 0 = \dfrac{{ - {P_0}}}{{{V_0}}}({V^2} - 3{V_0}V) \\
\Rightarrow 3{P_0} = \dfrac{{{P_0}}}{{{V_0}}}2V \\
\Rightarrow 2V - 3{V_0} = 0 \\
\Rightarrow V = \dfrac{{3{V_0}}}{2} \\
$
Substituting this value in the equation for temperature we get,
$
T = \dfrac{{ - {P_0}}}{{nR{V_0}}}({V^2} - 3{V_0}V) \\
\Rightarrow T = \dfrac{{ -{P_0}}}{{nR{V_0}}}(\dfrac{9}{4}{V_0}^2 - \dfrac{9}{2}{V_0}^2) \\
\Rightarrow T = \dfrac{{ -{P_0}}}{{nR{V_0}}}\dfrac{{ - 9}}{4}{V_0}^2 \\
\therefore T = \dfrac{{9{P_0}{V_0}}}{{4nR}} \\
$
Therefore, The maximum temperature of the gas during the process will be $\dfrac{{9{P_0}{V_0}}}{{4nR}}$.
Hence, the correct option is D.
Note: All assumptions are made considering the gas in question as an ideal gas. For real gases equations and relations become more complex and difficult to calculate. Therefore assuming gas to be an ideal gas is the easiest way to solve the question.
Formulae used:
Equation of a straight line: $(x - {x_0}) = \dfrac{{{x_2} - {x_1}}}{{{y_2} - {y_1}}}(y - {y_0})$
Where $y,{y_1},{y_2}$ and $x,{x_1},{x_2}$ are the y and x intercepts of a graph.
Ideal gas equation: $PV = nRT$
Where $P$ is the pressure of the gas and is expressed in Bars $(B)$, $V$ is the volume of the gas and is expressed in meter cube $({m^3})$, $n$ is the number of moles of the gas, $R$ is the universal gas constant and $T$ is the temperature of the gas and is expressed in Celsius $(^\circ C)$.
Complete step by step answer:
From the graph we have the following data:
At A:
Pressure $ = 2{P_0}$
Volume $ = {V_0}$
At B:
Pressure $ = {P_0}$
Volume $ = 2{V_0}$
To establish a relationship between the two, we will use the equation of a line for the given graph.
We know that, $(y - {y_0}) = \dfrac{{{y_2} - {y_1}}}{{{x_2} - {x_1}}}(x - {x_0})$.
Substituting the intercepts according to our graph we get,
$(V - {V_0}) = \dfrac{{{V_2} - {V_1}}}{{{P_2} - {P_1}}}(P - {P_0})$
According to the graph, \[{V_2} = 2{V_0},{V_1} = {V_0},{P_2} = {P_0},{P_1} = 2{P_0}\]. Substituting the same we get,
$(V - {V_0}) = \dfrac{{2{V_0} - {V_0}}}{{{P_0} - 2{P_0}}}(P - {P_0})$
Simplifying the above equation we get,
\[
(V - {V_0}) = \dfrac{{{V_0}}}{{ - {P_0}}}(P - {P_0}) \\
\Rightarrow (P - {P_0}) = (V - {V_0})\dfrac{{ - {P_0}}}{{{V_0}}} \\
\Rightarrow (P - {P_0}) = \dfrac{{ - {P_0}V}}{{{V_0}}} + 2{P_0} \\
\Rightarrow P = \dfrac{{ - {P_0}V}}{{{V_0}}} + 2{P_0} + {P_0} \\
\Rightarrow P = \dfrac{{ - {P_0}}}{{{V_0}}}(V - 3{V_0}) \\
\]
Now, we know that for an ideal gas $PV = nRT$
Rearranging this we get,
$P = \dfrac{{nRT}}{V}$
Substituting the above in the relationship established between volume and pressure we get,
$\dfrac{{nRT}}{V} = \dfrac{{ - {P_0}}}{{{V_0}}}(V - 3{V_0})$
Upon rearrangement to arrive at an expression for temperature $T$ we get,
$T = \dfrac{{ - {P_0}}}{{nR{V_0}}}({V^2} - 3{V_0}V)$
We need to determine the value of $V$.
Now, we know that at maximum temperature, rate of change of temperature with respect to volume of an ideal gas is constant. That is $\dfrac{{dT}}{{dV}} = 0$.
Therefore, differentiating the expression for temperature with respect to volume we get,
$\dfrac{{dT}}{{dV}} = \dfrac{{d(\dfrac{{ - {P_0}}}{{nR{V_0}}}({V^2} - 3{V_0}V))}}{{dV}}$
Upon simplification and substitution of boundary condition $\dfrac{{dT}}{{dV}} = 0$ we will establish a relationship between volume and pressure only,
$
\dfrac{{dT}}{{dV}} = \dfrac{{d(\dfrac{{ - {P_0}}}{{nR{V_0}}}({V^2} - 3{V_0}V))}}{{dV}} \\
\Rightarrow 0 = \dfrac{{d(\dfrac{{ - {P_0}}}{{nR{V_0}}}({V^2} - 3{V_0}V))}}{{dV}} \\
\Rightarrow 0 = \dfrac{{ - {P_0}}}{{{V_0}}}({V^2} - 3{V_0}V) \\
\Rightarrow 3{P_0} = \dfrac{{{P_0}}}{{{V_0}}}2V \\
\Rightarrow 2V - 3{V_0} = 0 \\
\Rightarrow V = \dfrac{{3{V_0}}}{2} \\
$
Substituting this value in the equation for temperature we get,
$
T = \dfrac{{ - {P_0}}}{{nR{V_0}}}({V^2} - 3{V_0}V) \\
\Rightarrow T = \dfrac{{ -{P_0}}}{{nR{V_0}}}(\dfrac{9}{4}{V_0}^2 - \dfrac{9}{2}{V_0}^2) \\
\Rightarrow T = \dfrac{{ -{P_0}}}{{nR{V_0}}}\dfrac{{ - 9}}{4}{V_0}^2 \\
\therefore T = \dfrac{{9{P_0}{V_0}}}{{4nR}} \\
$
Therefore, The maximum temperature of the gas during the process will be $\dfrac{{9{P_0}{V_0}}}{{4nR}}$.
Hence, the correct option is D.
Note: All assumptions are made considering the gas in question as an ideal gas. For real gases equations and relations become more complex and difficult to calculate. Therefore assuming gas to be an ideal gas is the easiest way to solve the question.
Recently Updated Pages
How many sigma and pi bonds are present in HCequiv class 11 chemistry CBSE
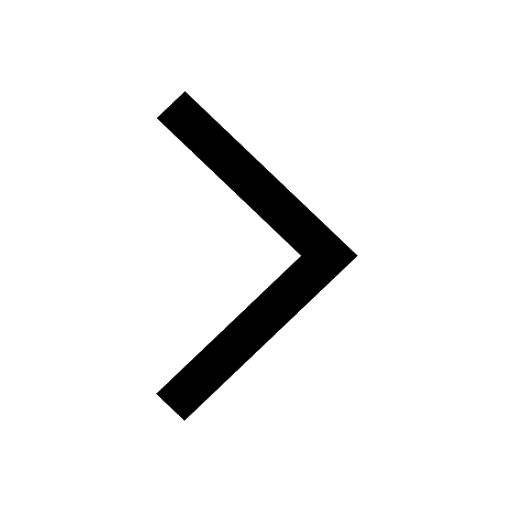
Why Are Noble Gases NonReactive class 11 chemistry CBSE
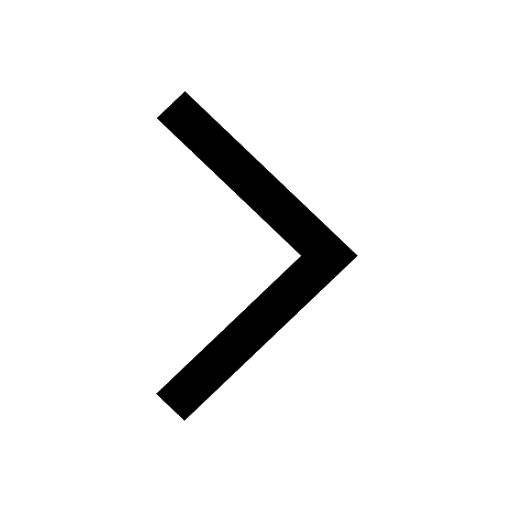
Let X and Y be the sets of all positive divisors of class 11 maths CBSE
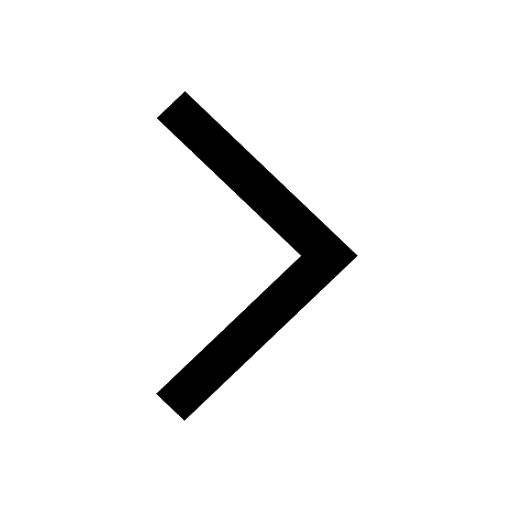
Let x and y be 2 real numbers which satisfy the equations class 11 maths CBSE
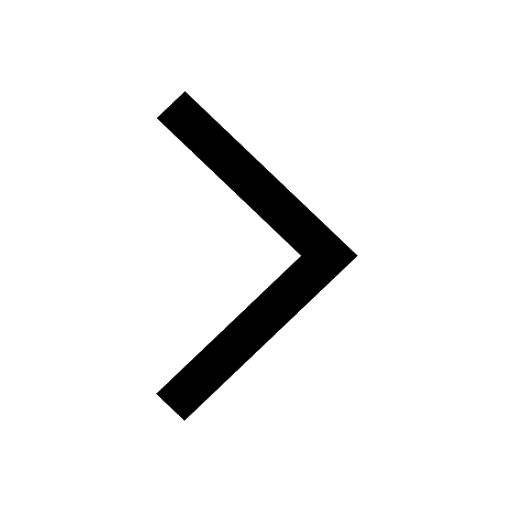
Let x 4log 2sqrt 9k 1 + 7 and y dfrac132log 2sqrt5 class 11 maths CBSE
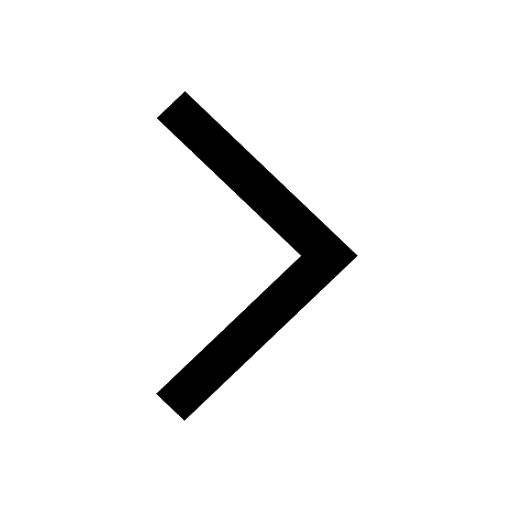
Let x22ax+b20 and x22bx+a20 be two equations Then the class 11 maths CBSE
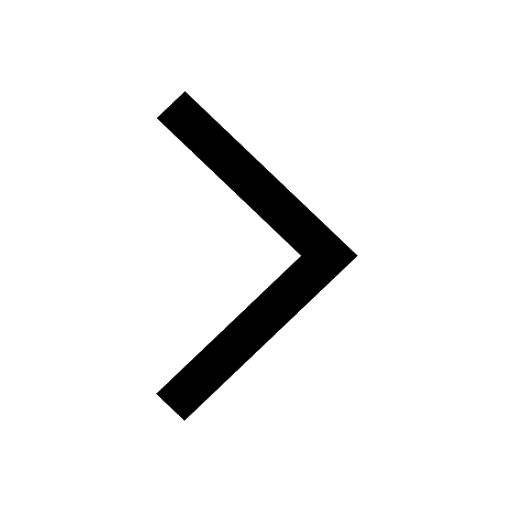
Trending doubts
Fill the blanks with the suitable prepositions 1 The class 9 english CBSE
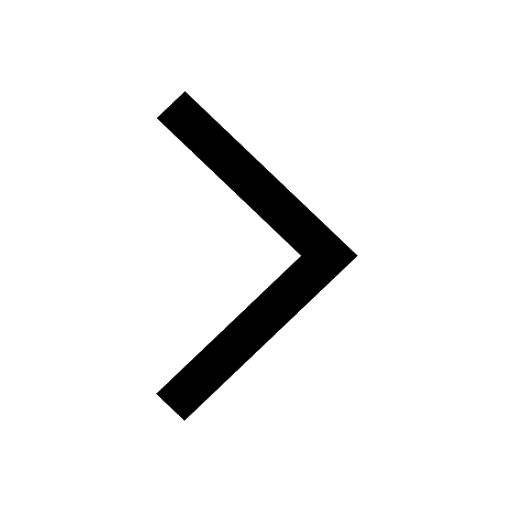
At which age domestication of animals started A Neolithic class 11 social science CBSE
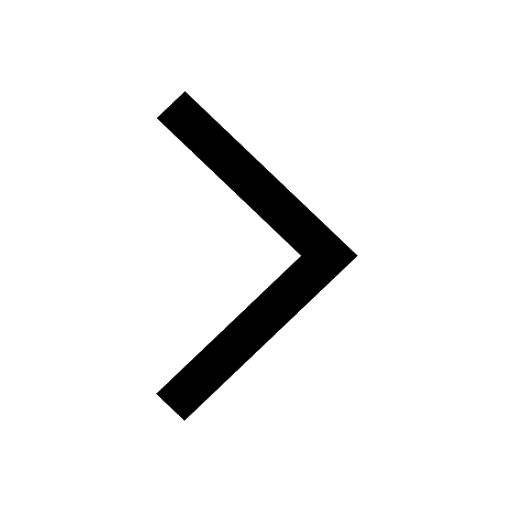
Which are the Top 10 Largest Countries of the World?
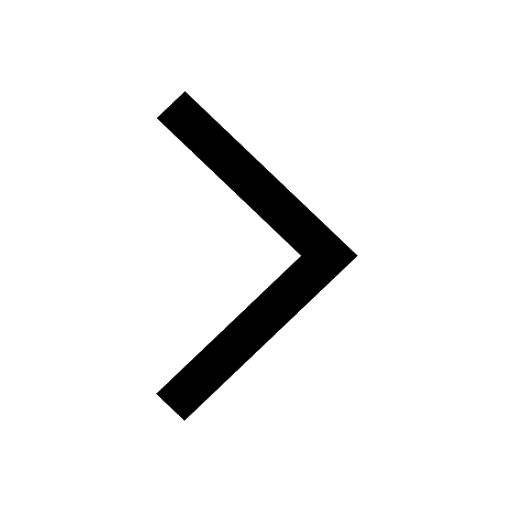
Give 10 examples for herbs , shrubs , climbers , creepers
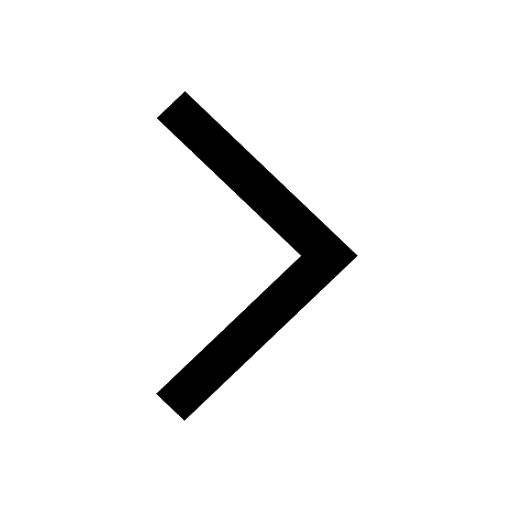
Difference between Prokaryotic cell and Eukaryotic class 11 biology CBSE
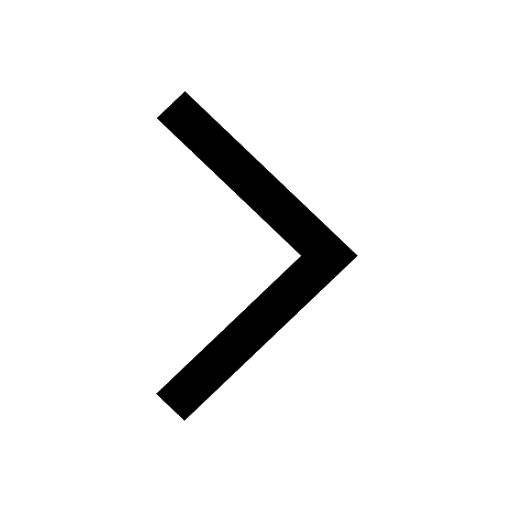
Difference Between Plant Cell and Animal Cell
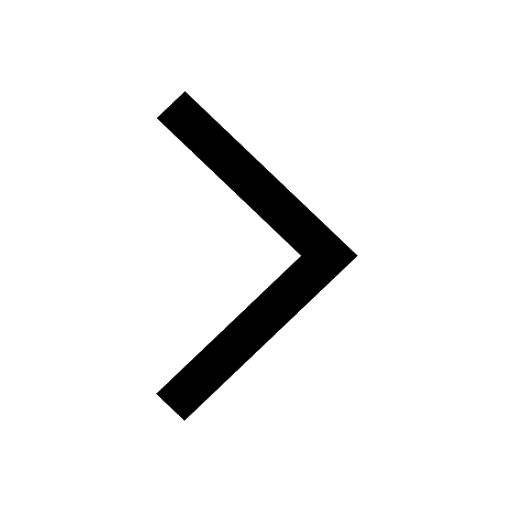
Write a letter to the principal requesting him to grant class 10 english CBSE
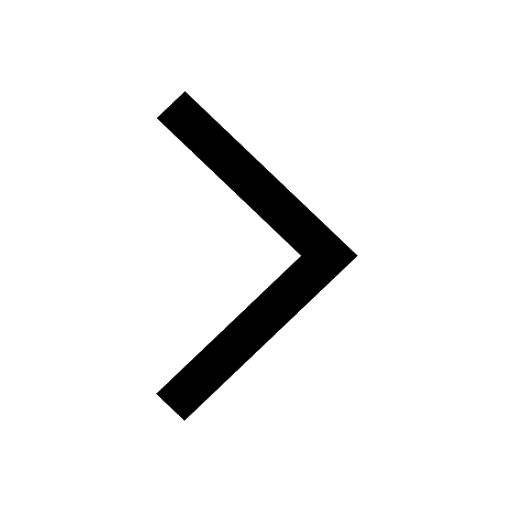
Change the following sentences into negative and interrogative class 10 english CBSE
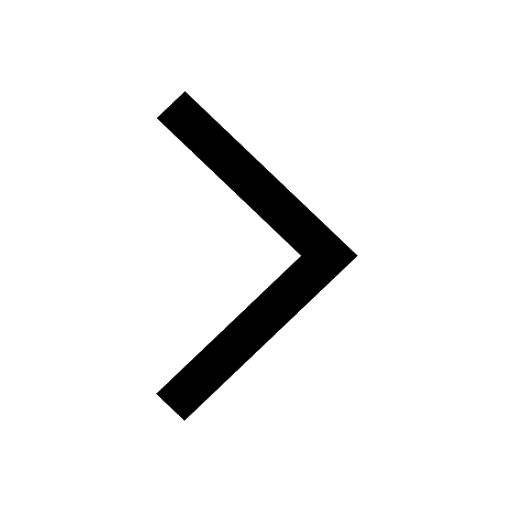
Fill in the blanks A 1 lakh ten thousand B 1 million class 9 maths CBSE
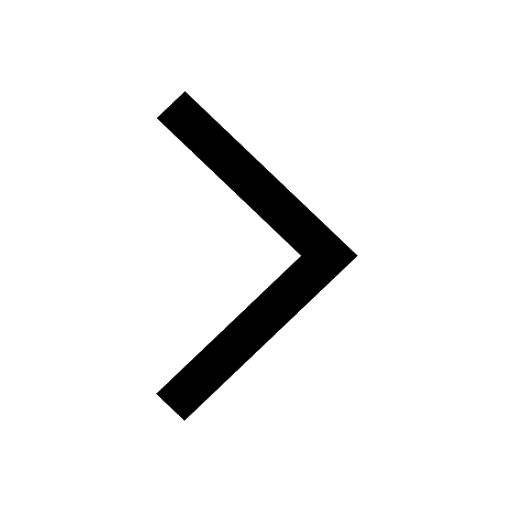