
Answer
480.9k+ views
Hint: Find vertices of parallelogram by intersection of tangents (sides of parallelogram) using parametric coordinates of point of contacts.
We have ellipse given;
$\dfrac{{{x}^{2}}}{{{a}^{2}}}+\dfrac{{{y}^{2}}}{{{b}^{2}}}=1................\left( i \right)$
As, given in question, two of the angular points of parallelogram is lying on ${{x}^{2}}={{h}^{2}}$.
$ABCD$is a parallelogram.
Let $AB,BC,CD,DA$ is touching ellipse at points $P,Q,R,S$ respectively. In other language, we can say that two tangents are drawn from points $P\text{ and }S,$ two tangents are from $P\text{ and }Q,$ two from $Q\text{ and }R;$ similarly, two tangents from $R\text{ and }S,$ which are intersecting at $A,B,C\And D$ respectively.
Let two points which are lying on ${{x}^{2}}={{h}^{2}}$are $B$and $D$.
Let point $P\text{ and }Q$ is $\left( a\cos \alpha ,b\sin \alpha \right)$ and $\left( a\cos \beta ,b\sin \beta \right)$ as parametric coordinates on ellipse $\dfrac{{{x}^{2}}}{{{a}^{2}}}+\dfrac{{{y}^{2}}}{{{b}^{2}}}=1.$
We know that tangent from any point $\left( {{x}_{1}},{{y}_{1}} \right)$ on ellipse is;
$T=0$
Or $\dfrac{x{{x}_{1}}}{{{a}_{2}}}+\dfrac{y{{y}_{1}}}{{{b}_{2}}}=1$
Hence, tangents through $P\left( a\cos \alpha ,b\sin \alpha \right)$ and $Q\left( a\cos \beta ,b\sin \beta \right)$;
$\dfrac{x\cos \alpha }{a}+\dfrac{y\sin \alpha }{b}=1\left( PB \right).............\left( ii \right)$
$\dfrac{x\cos \beta }{a}+\dfrac{y\sin \beta }{b}=1\left( BQ \right).............\left( iii \right)$
Let us find intersecting point of equation (ii) and (iii);
By cross multiplication method from equation (ii) and (iii);
$\begin{align}
& \dfrac{x}{\dfrac{\sin \alpha }{b}-\dfrac{\sin \beta }{b}}=\dfrac{-y}{\dfrac{\cos \alpha }{a}-\dfrac{\cos \beta }{a}}=\dfrac{-1}{\dfrac{\cos \alpha }{a}\dfrac{\sin \beta }{b}-\dfrac{\sin \alpha \cos \beta }{ab}} \\
& \dfrac{bx}{\left( \sin \alpha -\sin \beta \right)}=\dfrac{-ay}{\cos \alpha -\cos \beta }=\dfrac{-ab}{\cos \alpha \sin \beta -\sin \alpha \cos \beta } \\
\end{align}$
Simplifying the above relation to get $x$ and $y$
$x=\dfrac{a\left( \sin \alpha -\sin \beta \right)}{\sin \alpha \cos \beta -\cos \alpha \sin \beta },y=\dfrac{-b\left( \cos \alpha -\cos \beta \right)}{\sin \alpha \cos \beta -\cos \alpha \sin \beta }$
We have;
$\begin{align}
& \sin C-\sin D=2\sin \dfrac{C-D}{2}\cos \dfrac{C+D}{2} \\
& \cos C-\cos D=-2\sin \dfrac{C-D}{2}\sin \dfrac{C+D}{2} \\
& \sin C\cos D-\cos C\sin D=\sin \left( C-D \right) \\
\end{align}$
Therefore, we can rewrite $x$ and $y$as;
$x=\dfrac{2a\sin \left( \dfrac{\alpha -\beta }{2} \right)\cos \left( \dfrac{\alpha +\beta }{2} \right)}{\sin \left( \alpha -\beta \right)},y=\dfrac{2b\sin \dfrac{\alpha -\beta }{2}\sin \dfrac{\alpha +\beta }{2}}{-\sin \left( \alpha -\beta \right)}$
We have;
\[\begin{align}
& \sin 2\theta =2\sin \theta \cos \theta \\
& x=\dfrac{2a\sin \left( \dfrac{\alpha -\beta }{2} \right)\cos \left( \dfrac{\alpha +\beta }{2} \right)}{2\sin \left( \dfrac{\alpha -\beta }{2} \right)\cos \left( \dfrac{\alpha -\beta }{2} \right)}=\dfrac{a\cos \left( \dfrac{\alpha +\beta }{2} \right)}{\cos \left( \dfrac{\alpha -\beta }{2} \right)} \\
& y=\dfrac{2b\sin \left( \dfrac{\alpha -\beta }{2} \right)\sin \left( \dfrac{\alpha +\beta }{2} \right)}{2\sin \left( \dfrac{\alpha -\beta }{2} \right)\cos \left( \dfrac{\alpha -\beta }{2} \right)}=\dfrac{b\sin \left( \dfrac{\alpha +\beta }{2} \right)}{\cos \left( \dfrac{\alpha -\beta }{2} \right)} \\
& \left( x,y \right)=\left( \dfrac{a\cos \left( \dfrac{\alpha +\beta }{2} \right)}{\cos \left( \dfrac{\alpha -\beta }{2} \right)},\dfrac{b\sin \left( \dfrac{\alpha +\beta }{2} \right)}{\cos \left( \dfrac{\alpha -\beta }{2} \right)} \right)..........\left( iv \right) \\
\end{align}\]
Calculated $\left( x,y \right)$ in equation (iv) will lie on ${{x}^{2}}={{h}^{2}}$which is intersection of $PB$ and $BQ$ .
Let us find out points $A$ or $C$ of which we need to find locus.
Let us find out the intersection of $QC$ and $RC$ which is point $C$. Let's coordinate the point $C$ is $\left( {{x}_{_{1}}},{{y}_{1}} \right)$.
The parametric coordinate of point $Q$ is $\left( a\cos \beta ,b\sin \beta \right)$.
Now, by symmetry point $P$ and $R$ will lie exactly opposite to other as shown below;
Hence, parametric coordinate of point $R$is $a\cos \left( \pi +\alpha \right),b\sin \left( \pi +\alpha \right)\text{ or }-a\cos \alpha ,-b\sin \alpha .$
Now, let us write equation of tangents passing through $C$ i.e., $RC$ and $QC$ ;
$\begin{align}
& T=0 \\
& \dfrac{-xa\cos \alpha }{{{a}^{2}}}\dfrac{-yb\sin \alpha }{{{b}^{2}}}=1 \\
& \dfrac{x\cos \alpha }{a}+\dfrac{y\sin \alpha }{b}+1=0.............\left( v \right) \\
\end{align}$
Another tangent through $Q$ is;
$\dfrac{x\cos \beta }{a}+\dfrac{y\sin \beta }{b}-1=0.............\left( vi \right)$
Let us find out intersection of above two equations by cross multiplication method:
$\begin{align}
& \dfrac{x}{\dfrac{-\sin \alpha }{b}-\dfrac{\sin \beta }{b}}=\dfrac{-y}{\dfrac{-\cos \alpha }{a}-\dfrac{\cos \beta }{a}}=\dfrac{1}{\dfrac{\cos \alpha }{a}\dfrac{\sin \beta }{b}-\dfrac{\sin \alpha }{b}\dfrac{\cos \beta }{a}} \\
& \dfrac{-bx}{\sin \alpha +\sin \beta }=\dfrac{ay}{\cos \alpha +\cos \beta }=\dfrac{ab}{\cos \alpha \sin \beta -\sin \alpha \cos \beta } \\
\end{align}$
Now, we can write values of $x$ and $y$ as;
$\begin{align}
& x=\dfrac{a\left( \sin \alpha +\cos \beta \right)}{\sin \alpha \cos \beta -\cos \alpha \sin \beta } \\
& y=\dfrac{b\left( \cos \alpha +\cos \beta \right)}{\cos \alpha \sin \beta -\sin \alpha \cos \beta } \\
\end{align}$
We have,
$\begin{align}
& \sin C+\sin D=2\sin \dfrac{C+D}{2}\cos \dfrac{C-D}{2} \\
& \cos C+\cos D=-2\cos \dfrac{C+D}{2}\cos \dfrac{C-D}{2} \\
& \sin C\cos D-\cos C\sin D=\sin \left( C-D \right) \\
\end{align}$
Therefore, we can write $x$ and $y$ as;
$x=\dfrac{2a\sin \dfrac{\alpha +\beta }{2}\cos \dfrac{\alpha -\beta }{2}}{\sin \left( \alpha -\beta \right)}$
$\begin{align}
& x=\dfrac{2a\sin \dfrac{\alpha +\beta }{2}\cos \dfrac{\alpha -\beta }{2}}{2\sin \dfrac{\alpha -\beta }{2}\cos \dfrac{\alpha -\beta }{2}} \\
& x=\dfrac{a\sin \dfrac{\alpha +\beta }{2}}{\sin \dfrac{\alpha -\beta }{2}} \\
\end{align}$
And,
$\begin{align}
& y=\dfrac{2b\cos \dfrac{\alpha +\beta }{2}\cos \dfrac{\alpha -\beta }{2}}{\sin \left( \beta -\alpha \right)} \\
& y=\dfrac{2b\cos \dfrac{\alpha +\beta }{2}\cos \dfrac{\alpha -\beta }{2}}{-2\sin \dfrac{\alpha -\beta }{2}\cos \dfrac{\alpha -\beta }{2}} \\
& y=\dfrac{-b\cos \dfrac{\alpha +\beta }{2}}{\sin \dfrac{\alpha -\beta }{2}} \\
\end{align}$
Now, we have point $C\left( {{x}_{_{1}}},{{y}_{1}} \right)$as;
$\left( {{x}_{_{1}}},{{y}_{1}} \right)=\left( \dfrac{a\sin \dfrac{\alpha +\beta }{2}}{\sin \dfrac{\alpha -\beta }{2}},\dfrac{-b\cos \dfrac{\alpha +\beta }{2}}{\sin \dfrac{\alpha -\beta }{2}} \right).............\left( vii \right)$
As we have point $B(x,y)$ calculated in equation (iv) and will satisfy equation ${{x}^{2}}={{h}^{2}}$. Putting value of $x$ from equation (iv) to ${{x}^{2}}={{h}^{2}}$, we get;
$\dfrac{{{a}^{2}}{{\cos }^{2}}\dfrac{\alpha +\beta }{2}}{{{\cos }^{2}}\dfrac{\alpha -\beta }{2}}={{h}^{2}}...........\left( viii \right)$
Now, let us find out values of ${{\cos }^{2}}\dfrac{\alpha +\beta }{2}$,${{\cos }^{2}}\dfrac{\alpha -\beta }{2}$from equation (vii) in terms of ${{x}_{1}}\text{ and }{{y}_{1}}$, as;
${{x}_{_{1}}}=\dfrac{a\sin \dfrac{\alpha +\beta }{2}}{\sin \dfrac{\alpha -\beta }{2}},{{y}_{1}}=\dfrac{-b\cos \dfrac{\alpha +\beta }{2}}{\sin \dfrac{\alpha -\beta }{2}}$
Dividing ${{x}_{1}}\text{ and }{{y}_{1}}$, we get;
$\left( \dfrac{{{x}_{1}}}{{{y}_{1}}} \right)=\dfrac{-a}{b}\tan \left( \dfrac{\alpha +\beta }{2} \right)$
Squaring both sides, we get;
\[\left( \dfrac{{{x}_{1}}^{2}}{{{y}_{1}}^{2}} \right)=\dfrac{{{a}^{2}}}{{{b}^{2}}}{{\tan }^{2}}\left( \dfrac{\alpha +\beta }{2} \right)\]
We have relation $1+{{\tan }^{2}}\theta ={{\sec }^{2}}\theta $ or${{\tan }^{2}}\theta ={{\sec }^{2}}\theta -1,$using it in above equation, we get;
\[\begin{align}
& \dfrac{{{b}^{2}}{{x}_{1}}^{2}}{{{a}^{2}}{{y}_{1}}^{2}}={{\sec }^{2}}\dfrac{\alpha +\beta }{2}-1 \\
& {{\sec }^{2}}\dfrac{\alpha +\beta }{2}=1+\dfrac{{{b}^{2}}{{x}_{1}}^{2}}{{{a}^{2}}{{y}_{1}}^{2}} \\
& {{\sec }^{2}}\dfrac{\alpha +\beta }{2}=\dfrac{{{a}^{2}}{{y}_{1}}^{2}+{{b}^{2}}{{x}_{1}}^{2}}{{{a}^{2}}{{y}_{1}}^{2}} \\
& Or\text{ co}{{\text{s}}^{2}}\dfrac{\alpha +\beta }{2}=\dfrac{{{a}^{2}}{{y}_{1}}^{2}}{{{a}^{2}}{{y}_{1}}^{2}+{{b}^{2}}{{x}_{1}}^{2}}............\left( ix \right) \\
\end{align}\]
Now, let us calculate square of ${{x}_{1}}$;
${{x}_{_{1}}}^{2}=\dfrac{{{a}^{2}}{{\sin }^{2}}\dfrac{\alpha +\beta }{2}}{{{\sin }^{2}}\left( \dfrac{\alpha -\beta }{2} \right)}$
We have${{\sin }^{2}}\theta +{{\cos }^{2}}\theta =1$; Hence;
$\begin{align}
& {{x}_{_{1}}}^{2}=\dfrac{{{a}^{2}}\left( 1-{{\cos }^{2}}\dfrac{\alpha +\beta }{2} \right)}{{{\sin }^{2}}\left( \dfrac{\alpha -\beta }{2} \right)} \\
& {{\sin }^{2}}\dfrac{\alpha -\beta }{2}=\dfrac{{{a}^{2}}}{{{x}_{_{1}}}^{2}}\left( 1-\dfrac{{{a}^{2}}{{y}_{1}}^{2}}{{{a}^{2}}{{y}_{1}}^{2}+{{b}^{2}}{{x}_{1}}^{2}} \right) \\
& {{\sin }^{2}}\dfrac{\alpha -\beta }{2}=\dfrac{{{a}^{2}}}{{{x}_{_{1}}}^{2}}\left( \dfrac{{{b}^{2}}{{x}_{1}}^{2}}{{{a}^{2}}{{y}_{1}}^{2}+{{b}^{2}}{{x}_{1}}^{2}} \right) \\
& {{\sin }^{2}}\dfrac{\alpha -\beta }{2}=\dfrac{{{a}^{2}}{{b}^{2}}}{{{a}^{2}}{{y}_{1}}^{2}+{{b}^{2}}{{x}_{1}}^{2}} \\
& Or\text{ }1-{{\cos }^{2}}\dfrac{\alpha -\beta }{2}=\dfrac{{{a}^{2}}{{b}^{2}}}{{{a}^{2}}{{y}_{1}}^{2}+{{b}^{2}}{{x}_{1}}^{2}} \\
& {{\cos }^{2}}\dfrac{\alpha -\beta }{2}=\dfrac{{{a}^{2}}{{y}_{1}}^{2}+{{b}^{2}}{{x}_{1}}^{2}-{{a}^{2}}{{b}^{2}}}{{{a}^{2}}{{y}_{1}}^{2}+{{b}^{2}}{{x}_{1}}^{2}}...........\left( x \right) \\
\end{align}$
Putting values of ${{\cos }^{2}}\dfrac{\alpha +\beta }{2},{{\cos }^{2}}\dfrac{\alpha -\beta }{2}$from equation (ix) and (x) in equation (viii), we get;
$\begin{align}
& \dfrac{{{a}^{2}}\left( \dfrac{{{a}^{2}}{{y}_{1}}^{2}}{{{a}^{2}}{{y}_{1}}^{2}+{{b}^{2}}{{x}_{1}}^{2}} \right)}{\left( \dfrac{{{a}^{2}}{{y}_{1}}^{2}+{{b}^{2}}{{x}_{1}}^{2}-{{a}^{2}}{{b}^{2}}}{{{a}^{2}}{{y}_{1}}^{2}+{{b}^{2}}{{x}_{1}}^{2}} \right)}={{h}^{2}} \\
& \dfrac{{{a}^{4}}{{y}_{1}}^{2}}{{{a}^{2}}{{y}_{1}}^{2}+{{b}^{2}}{{x}_{1}}^{2}-{{a}^{2}}{{b}^{2}}}={{h}^{2}} \\
& \dfrac{{{a}^{4}}{{y}_{1}}^{2}}{{{h}^{2}}}={{a}^{2}}{{y}_{1}}^{2}+{{b}^{2}}{{x}_{1}}^{2}-{{a}^{2}}{{b}^{2}} \\
& {{a}^{2}}{{b}^{2}}={{y}_{1}}^{2}\left( {{a}^{2}}-\dfrac{{{a}^{2}}}{{{h}^{2}}} \right)+{{b}^{2}}{{x}_{1}}^{2} \\
\end{align}$
Dividing both sides by ${{a}^{2}}{{b}^{2}}$, we get;
$1=\dfrac{{{y}_{1}}^{2}}{{{b}^{2}}}\left( 1-\dfrac{{{a}^{2}}}{{{h}^{2}}} \right)+\dfrac{{{x}_{1}}^{2}}{{{a}^{2}}}$
Replacing $\left( {{x}_{1}},{{y}_{1}} \right)$ by $\left( x,y \right)$ to get the required locus, we get;
$\dfrac{{{x}_{1}}^{2}}{{{a}^{2}}}+\dfrac{{{y}_{1}}^{2}}{{{b}^{2}}}\left( 1-\dfrac{{{a}^{2}}}{{{h}^{2}}} \right)=1$
Hence, proved.
Note: One can calculate intersection of tangent $PA$ and $AS$ to get point $A$ where point $P\text{ and }S$ has parametric coordinates as $\left( a\cos \alpha ,b\sin \alpha \right),\left( a\cos \left( \pi +\beta \right),b\sin \left( \pi +\beta \right) \right)$. Solution will be the same. Calculation is the important part of these kinds of questions.
One can use a substitution and elimination approach to find intersecting points of tangents but that will be a longer process than cross – multiplication which gives $\left( x,y \right)$ in one line.
By symmetry of ellipse points $\left( P,R \right)\text{ and }\left( Q,S \right)$ will be opposite to each other at a difference of $180{}^\circ $ eccentric angle which is the key point of this question..
We have ellipse given;
$\dfrac{{{x}^{2}}}{{{a}^{2}}}+\dfrac{{{y}^{2}}}{{{b}^{2}}}=1................\left( i \right)$
As, given in question, two of the angular points of parallelogram is lying on ${{x}^{2}}={{h}^{2}}$.
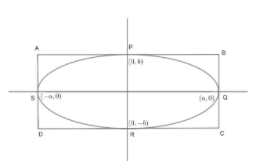
$ABCD$is a parallelogram.
Let $AB,BC,CD,DA$ is touching ellipse at points $P,Q,R,S$ respectively. In other language, we can say that two tangents are drawn from points $P\text{ and }S,$ two tangents are from $P\text{ and }Q,$ two from $Q\text{ and }R;$ similarly, two tangents from $R\text{ and }S,$ which are intersecting at $A,B,C\And D$ respectively.
Let two points which are lying on ${{x}^{2}}={{h}^{2}}$are $B$and $D$.
Let point $P\text{ and }Q$ is $\left( a\cos \alpha ,b\sin \alpha \right)$ and $\left( a\cos \beta ,b\sin \beta \right)$ as parametric coordinates on ellipse $\dfrac{{{x}^{2}}}{{{a}^{2}}}+\dfrac{{{y}^{2}}}{{{b}^{2}}}=1.$
We know that tangent from any point $\left( {{x}_{1}},{{y}_{1}} \right)$ on ellipse is;
$T=0$
Or $\dfrac{x{{x}_{1}}}{{{a}_{2}}}+\dfrac{y{{y}_{1}}}{{{b}_{2}}}=1$
Hence, tangents through $P\left( a\cos \alpha ,b\sin \alpha \right)$ and $Q\left( a\cos \beta ,b\sin \beta \right)$;
$\dfrac{x\cos \alpha }{a}+\dfrac{y\sin \alpha }{b}=1\left( PB \right).............\left( ii \right)$
$\dfrac{x\cos \beta }{a}+\dfrac{y\sin \beta }{b}=1\left( BQ \right).............\left( iii \right)$
Let us find intersecting point of equation (ii) and (iii);
By cross multiplication method from equation (ii) and (iii);
$\begin{align}
& \dfrac{x}{\dfrac{\sin \alpha }{b}-\dfrac{\sin \beta }{b}}=\dfrac{-y}{\dfrac{\cos \alpha }{a}-\dfrac{\cos \beta }{a}}=\dfrac{-1}{\dfrac{\cos \alpha }{a}\dfrac{\sin \beta }{b}-\dfrac{\sin \alpha \cos \beta }{ab}} \\
& \dfrac{bx}{\left( \sin \alpha -\sin \beta \right)}=\dfrac{-ay}{\cos \alpha -\cos \beta }=\dfrac{-ab}{\cos \alpha \sin \beta -\sin \alpha \cos \beta } \\
\end{align}$
Simplifying the above relation to get $x$ and $y$
$x=\dfrac{a\left( \sin \alpha -\sin \beta \right)}{\sin \alpha \cos \beta -\cos \alpha \sin \beta },y=\dfrac{-b\left( \cos \alpha -\cos \beta \right)}{\sin \alpha \cos \beta -\cos \alpha \sin \beta }$
We have;
$\begin{align}
& \sin C-\sin D=2\sin \dfrac{C-D}{2}\cos \dfrac{C+D}{2} \\
& \cos C-\cos D=-2\sin \dfrac{C-D}{2}\sin \dfrac{C+D}{2} \\
& \sin C\cos D-\cos C\sin D=\sin \left( C-D \right) \\
\end{align}$
Therefore, we can rewrite $x$ and $y$as;
$x=\dfrac{2a\sin \left( \dfrac{\alpha -\beta }{2} \right)\cos \left( \dfrac{\alpha +\beta }{2} \right)}{\sin \left( \alpha -\beta \right)},y=\dfrac{2b\sin \dfrac{\alpha -\beta }{2}\sin \dfrac{\alpha +\beta }{2}}{-\sin \left( \alpha -\beta \right)}$
We have;
\[\begin{align}
& \sin 2\theta =2\sin \theta \cos \theta \\
& x=\dfrac{2a\sin \left( \dfrac{\alpha -\beta }{2} \right)\cos \left( \dfrac{\alpha +\beta }{2} \right)}{2\sin \left( \dfrac{\alpha -\beta }{2} \right)\cos \left( \dfrac{\alpha -\beta }{2} \right)}=\dfrac{a\cos \left( \dfrac{\alpha +\beta }{2} \right)}{\cos \left( \dfrac{\alpha -\beta }{2} \right)} \\
& y=\dfrac{2b\sin \left( \dfrac{\alpha -\beta }{2} \right)\sin \left( \dfrac{\alpha +\beta }{2} \right)}{2\sin \left( \dfrac{\alpha -\beta }{2} \right)\cos \left( \dfrac{\alpha -\beta }{2} \right)}=\dfrac{b\sin \left( \dfrac{\alpha +\beta }{2} \right)}{\cos \left( \dfrac{\alpha -\beta }{2} \right)} \\
& \left( x,y \right)=\left( \dfrac{a\cos \left( \dfrac{\alpha +\beta }{2} \right)}{\cos \left( \dfrac{\alpha -\beta }{2} \right)},\dfrac{b\sin \left( \dfrac{\alpha +\beta }{2} \right)}{\cos \left( \dfrac{\alpha -\beta }{2} \right)} \right)..........\left( iv \right) \\
\end{align}\]
Calculated $\left( x,y \right)$ in equation (iv) will lie on ${{x}^{2}}={{h}^{2}}$which is intersection of $PB$ and $BQ$ .
Let us find out points $A$ or $C$ of which we need to find locus.
Let us find out the intersection of $QC$ and $RC$ which is point $C$. Let's coordinate the point $C$ is $\left( {{x}_{_{1}}},{{y}_{1}} \right)$.
The parametric coordinate of point $Q$ is $\left( a\cos \beta ,b\sin \beta \right)$.
Now, by symmetry point $P$ and $R$ will lie exactly opposite to other as shown below;
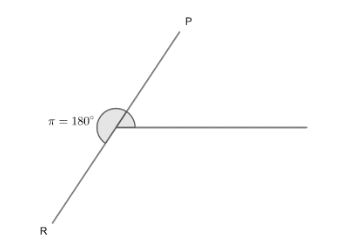
Hence, parametric coordinate of point $R$is $a\cos \left( \pi +\alpha \right),b\sin \left( \pi +\alpha \right)\text{ or }-a\cos \alpha ,-b\sin \alpha .$
Now, let us write equation of tangents passing through $C$ i.e., $RC$ and $QC$ ;
$\begin{align}
& T=0 \\
& \dfrac{-xa\cos \alpha }{{{a}^{2}}}\dfrac{-yb\sin \alpha }{{{b}^{2}}}=1 \\
& \dfrac{x\cos \alpha }{a}+\dfrac{y\sin \alpha }{b}+1=0.............\left( v \right) \\
\end{align}$
Another tangent through $Q$ is;
$\dfrac{x\cos \beta }{a}+\dfrac{y\sin \beta }{b}-1=0.............\left( vi \right)$
Let us find out intersection of above two equations by cross multiplication method:
$\begin{align}
& \dfrac{x}{\dfrac{-\sin \alpha }{b}-\dfrac{\sin \beta }{b}}=\dfrac{-y}{\dfrac{-\cos \alpha }{a}-\dfrac{\cos \beta }{a}}=\dfrac{1}{\dfrac{\cos \alpha }{a}\dfrac{\sin \beta }{b}-\dfrac{\sin \alpha }{b}\dfrac{\cos \beta }{a}} \\
& \dfrac{-bx}{\sin \alpha +\sin \beta }=\dfrac{ay}{\cos \alpha +\cos \beta }=\dfrac{ab}{\cos \alpha \sin \beta -\sin \alpha \cos \beta } \\
\end{align}$
Now, we can write values of $x$ and $y$ as;
$\begin{align}
& x=\dfrac{a\left( \sin \alpha +\cos \beta \right)}{\sin \alpha \cos \beta -\cos \alpha \sin \beta } \\
& y=\dfrac{b\left( \cos \alpha +\cos \beta \right)}{\cos \alpha \sin \beta -\sin \alpha \cos \beta } \\
\end{align}$
We have,
$\begin{align}
& \sin C+\sin D=2\sin \dfrac{C+D}{2}\cos \dfrac{C-D}{2} \\
& \cos C+\cos D=-2\cos \dfrac{C+D}{2}\cos \dfrac{C-D}{2} \\
& \sin C\cos D-\cos C\sin D=\sin \left( C-D \right) \\
\end{align}$
Therefore, we can write $x$ and $y$ as;
$x=\dfrac{2a\sin \dfrac{\alpha +\beta }{2}\cos \dfrac{\alpha -\beta }{2}}{\sin \left( \alpha -\beta \right)}$
$\begin{align}
& x=\dfrac{2a\sin \dfrac{\alpha +\beta }{2}\cos \dfrac{\alpha -\beta }{2}}{2\sin \dfrac{\alpha -\beta }{2}\cos \dfrac{\alpha -\beta }{2}} \\
& x=\dfrac{a\sin \dfrac{\alpha +\beta }{2}}{\sin \dfrac{\alpha -\beta }{2}} \\
\end{align}$
And,
$\begin{align}
& y=\dfrac{2b\cos \dfrac{\alpha +\beta }{2}\cos \dfrac{\alpha -\beta }{2}}{\sin \left( \beta -\alpha \right)} \\
& y=\dfrac{2b\cos \dfrac{\alpha +\beta }{2}\cos \dfrac{\alpha -\beta }{2}}{-2\sin \dfrac{\alpha -\beta }{2}\cos \dfrac{\alpha -\beta }{2}} \\
& y=\dfrac{-b\cos \dfrac{\alpha +\beta }{2}}{\sin \dfrac{\alpha -\beta }{2}} \\
\end{align}$
Now, we have point $C\left( {{x}_{_{1}}},{{y}_{1}} \right)$as;
$\left( {{x}_{_{1}}},{{y}_{1}} \right)=\left( \dfrac{a\sin \dfrac{\alpha +\beta }{2}}{\sin \dfrac{\alpha -\beta }{2}},\dfrac{-b\cos \dfrac{\alpha +\beta }{2}}{\sin \dfrac{\alpha -\beta }{2}} \right).............\left( vii \right)$
As we have point $B(x,y)$ calculated in equation (iv) and will satisfy equation ${{x}^{2}}={{h}^{2}}$. Putting value of $x$ from equation (iv) to ${{x}^{2}}={{h}^{2}}$, we get;
$\dfrac{{{a}^{2}}{{\cos }^{2}}\dfrac{\alpha +\beta }{2}}{{{\cos }^{2}}\dfrac{\alpha -\beta }{2}}={{h}^{2}}...........\left( viii \right)$
Now, let us find out values of ${{\cos }^{2}}\dfrac{\alpha +\beta }{2}$,${{\cos }^{2}}\dfrac{\alpha -\beta }{2}$from equation (vii) in terms of ${{x}_{1}}\text{ and }{{y}_{1}}$, as;
${{x}_{_{1}}}=\dfrac{a\sin \dfrac{\alpha +\beta }{2}}{\sin \dfrac{\alpha -\beta }{2}},{{y}_{1}}=\dfrac{-b\cos \dfrac{\alpha +\beta }{2}}{\sin \dfrac{\alpha -\beta }{2}}$
Dividing ${{x}_{1}}\text{ and }{{y}_{1}}$, we get;
$\left( \dfrac{{{x}_{1}}}{{{y}_{1}}} \right)=\dfrac{-a}{b}\tan \left( \dfrac{\alpha +\beta }{2} \right)$
Squaring both sides, we get;
\[\left( \dfrac{{{x}_{1}}^{2}}{{{y}_{1}}^{2}} \right)=\dfrac{{{a}^{2}}}{{{b}^{2}}}{{\tan }^{2}}\left( \dfrac{\alpha +\beta }{2} \right)\]
We have relation $1+{{\tan }^{2}}\theta ={{\sec }^{2}}\theta $ or${{\tan }^{2}}\theta ={{\sec }^{2}}\theta -1,$using it in above equation, we get;
\[\begin{align}
& \dfrac{{{b}^{2}}{{x}_{1}}^{2}}{{{a}^{2}}{{y}_{1}}^{2}}={{\sec }^{2}}\dfrac{\alpha +\beta }{2}-1 \\
& {{\sec }^{2}}\dfrac{\alpha +\beta }{2}=1+\dfrac{{{b}^{2}}{{x}_{1}}^{2}}{{{a}^{2}}{{y}_{1}}^{2}} \\
& {{\sec }^{2}}\dfrac{\alpha +\beta }{2}=\dfrac{{{a}^{2}}{{y}_{1}}^{2}+{{b}^{2}}{{x}_{1}}^{2}}{{{a}^{2}}{{y}_{1}}^{2}} \\
& Or\text{ co}{{\text{s}}^{2}}\dfrac{\alpha +\beta }{2}=\dfrac{{{a}^{2}}{{y}_{1}}^{2}}{{{a}^{2}}{{y}_{1}}^{2}+{{b}^{2}}{{x}_{1}}^{2}}............\left( ix \right) \\
\end{align}\]
Now, let us calculate square of ${{x}_{1}}$;
${{x}_{_{1}}}^{2}=\dfrac{{{a}^{2}}{{\sin }^{2}}\dfrac{\alpha +\beta }{2}}{{{\sin }^{2}}\left( \dfrac{\alpha -\beta }{2} \right)}$
We have${{\sin }^{2}}\theta +{{\cos }^{2}}\theta =1$; Hence;
$\begin{align}
& {{x}_{_{1}}}^{2}=\dfrac{{{a}^{2}}\left( 1-{{\cos }^{2}}\dfrac{\alpha +\beta }{2} \right)}{{{\sin }^{2}}\left( \dfrac{\alpha -\beta }{2} \right)} \\
& {{\sin }^{2}}\dfrac{\alpha -\beta }{2}=\dfrac{{{a}^{2}}}{{{x}_{_{1}}}^{2}}\left( 1-\dfrac{{{a}^{2}}{{y}_{1}}^{2}}{{{a}^{2}}{{y}_{1}}^{2}+{{b}^{2}}{{x}_{1}}^{2}} \right) \\
& {{\sin }^{2}}\dfrac{\alpha -\beta }{2}=\dfrac{{{a}^{2}}}{{{x}_{_{1}}}^{2}}\left( \dfrac{{{b}^{2}}{{x}_{1}}^{2}}{{{a}^{2}}{{y}_{1}}^{2}+{{b}^{2}}{{x}_{1}}^{2}} \right) \\
& {{\sin }^{2}}\dfrac{\alpha -\beta }{2}=\dfrac{{{a}^{2}}{{b}^{2}}}{{{a}^{2}}{{y}_{1}}^{2}+{{b}^{2}}{{x}_{1}}^{2}} \\
& Or\text{ }1-{{\cos }^{2}}\dfrac{\alpha -\beta }{2}=\dfrac{{{a}^{2}}{{b}^{2}}}{{{a}^{2}}{{y}_{1}}^{2}+{{b}^{2}}{{x}_{1}}^{2}} \\
& {{\cos }^{2}}\dfrac{\alpha -\beta }{2}=\dfrac{{{a}^{2}}{{y}_{1}}^{2}+{{b}^{2}}{{x}_{1}}^{2}-{{a}^{2}}{{b}^{2}}}{{{a}^{2}}{{y}_{1}}^{2}+{{b}^{2}}{{x}_{1}}^{2}}...........\left( x \right) \\
\end{align}$
Putting values of ${{\cos }^{2}}\dfrac{\alpha +\beta }{2},{{\cos }^{2}}\dfrac{\alpha -\beta }{2}$from equation (ix) and (x) in equation (viii), we get;
$\begin{align}
& \dfrac{{{a}^{2}}\left( \dfrac{{{a}^{2}}{{y}_{1}}^{2}}{{{a}^{2}}{{y}_{1}}^{2}+{{b}^{2}}{{x}_{1}}^{2}} \right)}{\left( \dfrac{{{a}^{2}}{{y}_{1}}^{2}+{{b}^{2}}{{x}_{1}}^{2}-{{a}^{2}}{{b}^{2}}}{{{a}^{2}}{{y}_{1}}^{2}+{{b}^{2}}{{x}_{1}}^{2}} \right)}={{h}^{2}} \\
& \dfrac{{{a}^{4}}{{y}_{1}}^{2}}{{{a}^{2}}{{y}_{1}}^{2}+{{b}^{2}}{{x}_{1}}^{2}-{{a}^{2}}{{b}^{2}}}={{h}^{2}} \\
& \dfrac{{{a}^{4}}{{y}_{1}}^{2}}{{{h}^{2}}}={{a}^{2}}{{y}_{1}}^{2}+{{b}^{2}}{{x}_{1}}^{2}-{{a}^{2}}{{b}^{2}} \\
& {{a}^{2}}{{b}^{2}}={{y}_{1}}^{2}\left( {{a}^{2}}-\dfrac{{{a}^{2}}}{{{h}^{2}}} \right)+{{b}^{2}}{{x}_{1}}^{2} \\
\end{align}$
Dividing both sides by ${{a}^{2}}{{b}^{2}}$, we get;
$1=\dfrac{{{y}_{1}}^{2}}{{{b}^{2}}}\left( 1-\dfrac{{{a}^{2}}}{{{h}^{2}}} \right)+\dfrac{{{x}_{1}}^{2}}{{{a}^{2}}}$
Replacing $\left( {{x}_{1}},{{y}_{1}} \right)$ by $\left( x,y \right)$ to get the required locus, we get;
$\dfrac{{{x}_{1}}^{2}}{{{a}^{2}}}+\dfrac{{{y}_{1}}^{2}}{{{b}^{2}}}\left( 1-\dfrac{{{a}^{2}}}{{{h}^{2}}} \right)=1$
Hence, proved.
Note: One can calculate intersection of tangent $PA$ and $AS$ to get point $A$ where point $P\text{ and }S$ has parametric coordinates as $\left( a\cos \alpha ,b\sin \alpha \right),\left( a\cos \left( \pi +\beta \right),b\sin \left( \pi +\beta \right) \right)$. Solution will be the same. Calculation is the important part of these kinds of questions.
One can use a substitution and elimination approach to find intersecting points of tangents but that will be a longer process than cross – multiplication which gives $\left( x,y \right)$ in one line.
By symmetry of ellipse points $\left( P,R \right)\text{ and }\left( Q,S \right)$ will be opposite to each other at a difference of $180{}^\circ $ eccentric angle which is the key point of this question..
Recently Updated Pages
How many sigma and pi bonds are present in HCequiv class 11 chemistry CBSE
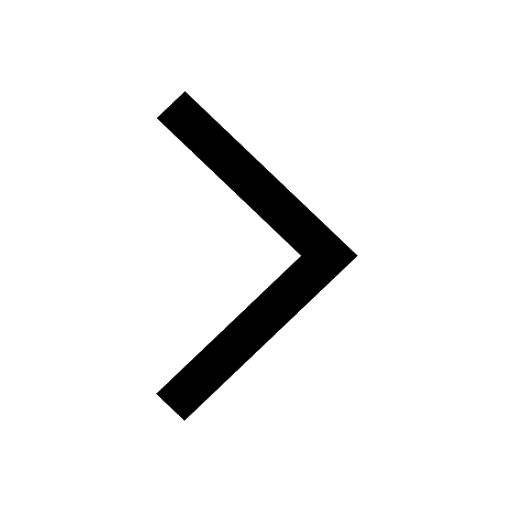
Mark and label the given geoinformation on the outline class 11 social science CBSE
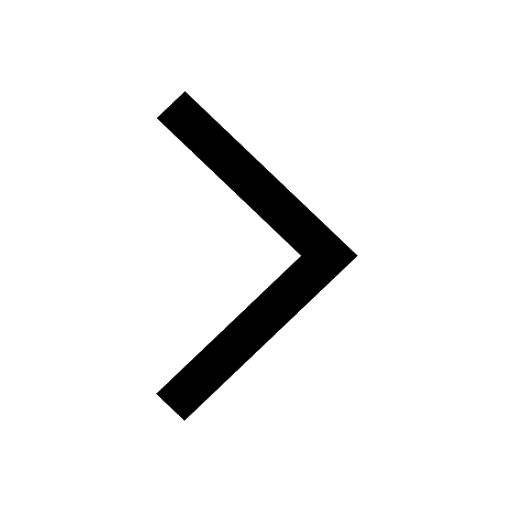
When people say No pun intended what does that mea class 8 english CBSE
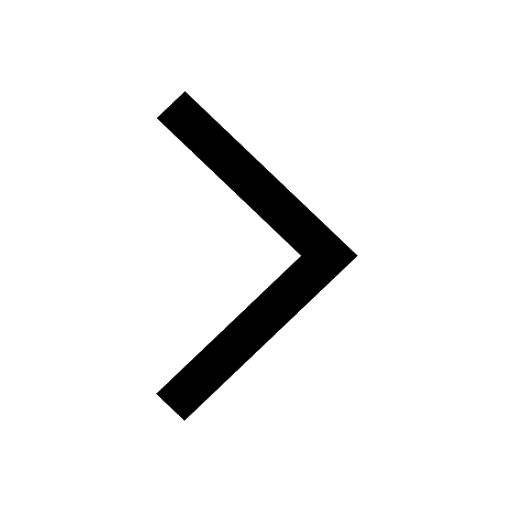
Name the states which share their boundary with Indias class 9 social science CBSE
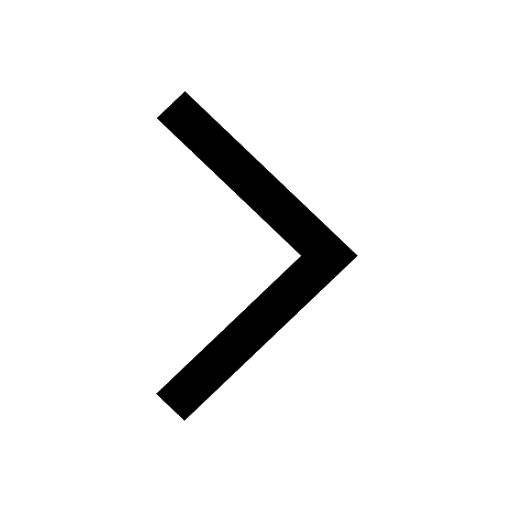
Give an account of the Northern Plains of India class 9 social science CBSE
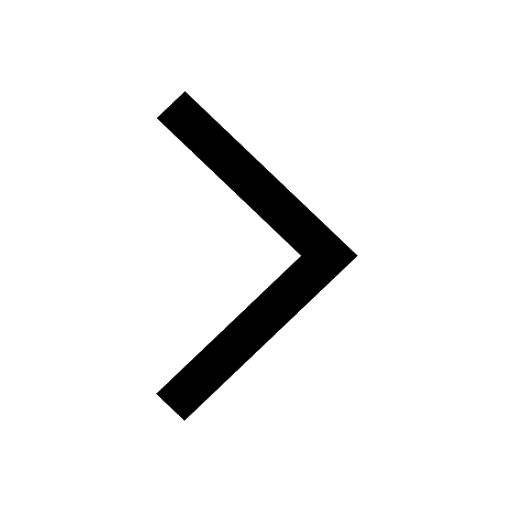
Change the following sentences into negative and interrogative class 10 english CBSE
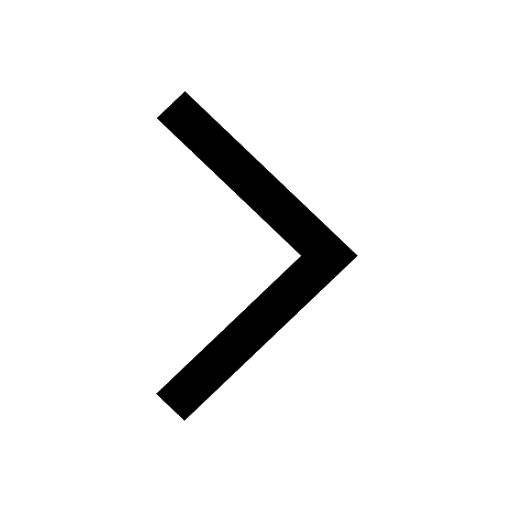
Trending doubts
Fill the blanks with the suitable prepositions 1 The class 9 english CBSE
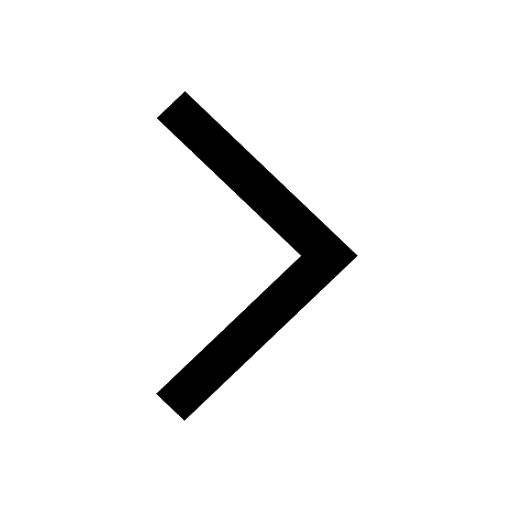
Which are the Top 10 Largest Countries of the World?
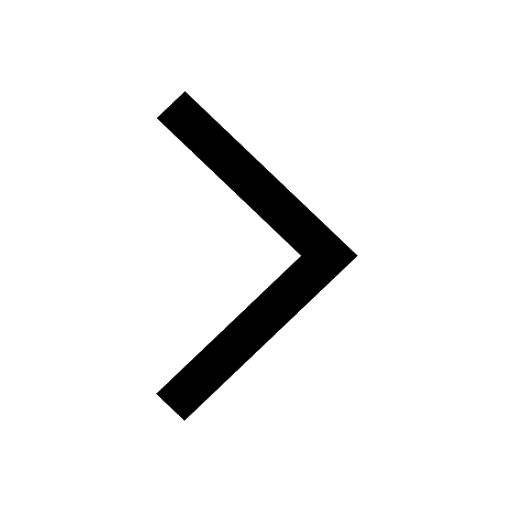
Give 10 examples for herbs , shrubs , climbers , creepers
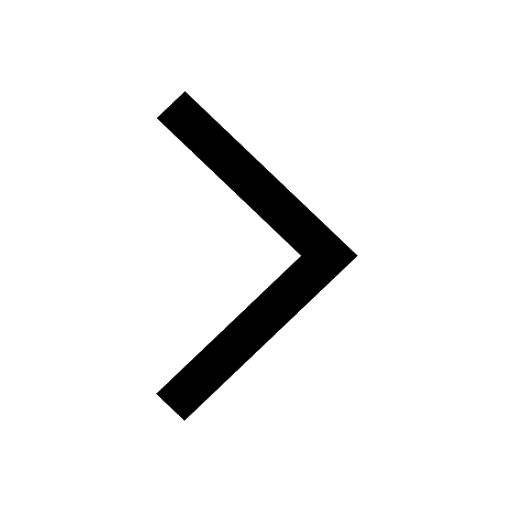
Difference Between Plant Cell and Animal Cell
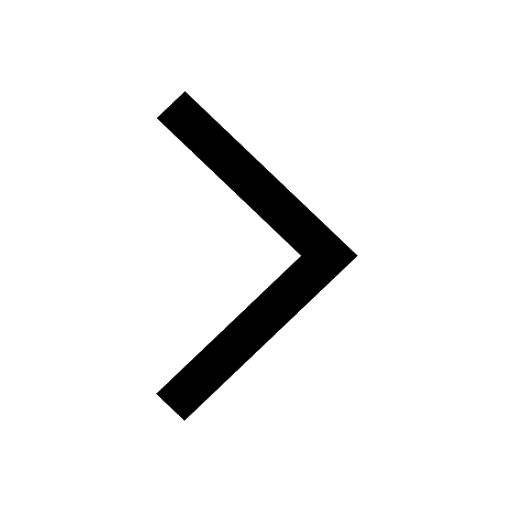
Difference between Prokaryotic cell and Eukaryotic class 11 biology CBSE
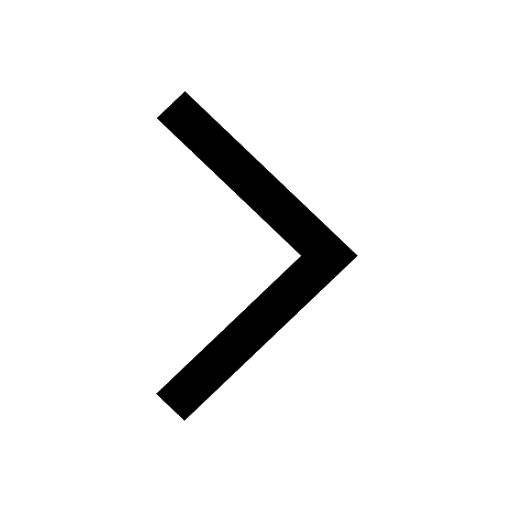
The Equation xxx + 2 is Satisfied when x is Equal to Class 10 Maths
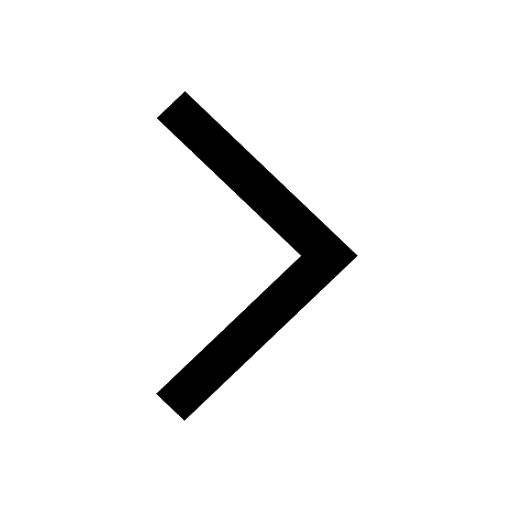
Change the following sentences into negative and interrogative class 10 english CBSE
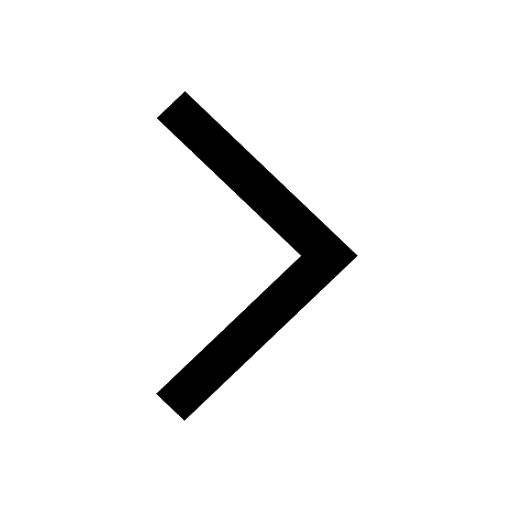
How do you graph the function fx 4x class 9 maths CBSE
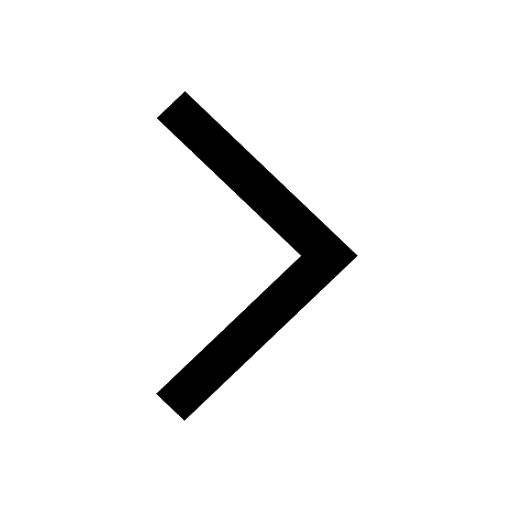
Write a letter to the principal requesting him to grant class 10 english CBSE
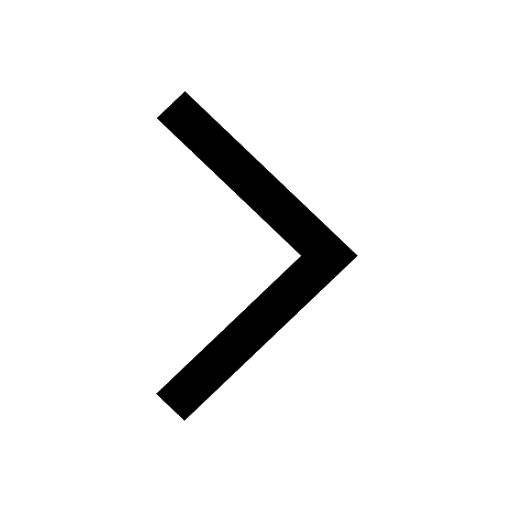