
Answer
376.2k+ views
Hint: Find the equation of motion of the particle and use the conservation of mechanical energy at starting point and at the point of the balance condition. From there find the speed of the particle at the point where the tension in the string is equal to the weight of the particle.
Formula used:
Newton's second law is given by,
\[F = ma\]
where \[m\] is the mass of the particle and \[a\] is the acceleration of the particle.
The conservation of mechanical energy is given by,
\[{K_i} + {U_i} = {K_f} + {U_f}\]
where, \[{K_i}\] and \[{U_i}\] are the initial kinetic and potential energy and \[{K_f},{U_f}\] are the final kinetic and potential energy.
Complete step by step answer:
We have given here that the particle is given a velocity of \[\sqrt {gl} \] horizontally. Now, the particle will start swinging due to this. So, we have to find the velocity of the particle when the weight of the particle will be equal to the tension in the string. Now, there are two forces acting on the particle vertically one is the centrifugal force and another is the gravitational pull on the particle. Let, the mass of the particle is $m$.
So, the equation of motion of the particle along the wire can be written as, \[T = mg\cos \theta + \dfrac{{m{v^2}}}{l}\]
Now, according to the question this is equal to the weight of the particle hence we can write,
\[mg = mg\cos \theta + \dfrac{{m{v^2}}}{l}\]….(i)
Now, from conservation of mechanical energy we can write,
\[\dfrac{1}{2}m{(\sqrt {gl} )^2} = mgl' + \dfrac{1}{2}m{(v)^2}\]
Now, from the diagram we can see that, \[l' = l - l\cos \theta \].
So, putting this value we have,
\[\dfrac{1}{2}mgl = mgl(1 - \cos \theta ) + \dfrac{1}{2}m{(v)^2}\]
\[\Rightarrow \dfrac{1}{2}mgl - mgl = - mgl\cos \theta + \dfrac{1}{2}m{(v)^2}\]
Upon simplifying we have,
\[gl\cos \theta = \dfrac{1}{2}({v^2} + gl)\]
\[\Rightarrow \cos \theta = \dfrac{1}{{2gl}}({v^2} + gl)\]…….(ii)
Putting these value in equation (i) we have,
\[mg = mg\cos \theta + \dfrac{{m{v^2}}}{l}\]
\[\Rightarrow mg = mg\dfrac{1}{{2gl}}({v^2} + gl) + \dfrac{{m{v^2}}}{l}\]
\[\Rightarrow g = \dfrac{1}{{2l}}({v^2} + gl) + \dfrac{{{v^2}}}{l}\]
Upon simplifying we have,
\[g = \dfrac{{{v^2}}}{{2l}} + \dfrac{g}{2} + \dfrac{{{v^2}}}{l}\]
\[\Rightarrow g - \dfrac{g}{2} = \dfrac{{3{v^2}}}{{2l}}\]
\[\Rightarrow {v^2} = \dfrac{{gl}}{3}\]
\[\therefore v = \sqrt {\dfrac{{gl}}{3}} \]
Hence, the speed of a particle at the point where the tension in the string equals weight of the particle is \[\sqrt {\dfrac{{gl}}{3}} \].
Note: The point at which the particle starts swinging the weight of the particle may seem equal to the tension in the string. But, at the point of starting the particle has acceleration acting in the horizontal direction and since the tension and the weight of the particle is not equal it has some motion along the vertical along with the horizontal line also.
Formula used:
Newton's second law is given by,
\[F = ma\]
where \[m\] is the mass of the particle and \[a\] is the acceleration of the particle.
The conservation of mechanical energy is given by,
\[{K_i} + {U_i} = {K_f} + {U_f}\]
where, \[{K_i}\] and \[{U_i}\] are the initial kinetic and potential energy and \[{K_f},{U_f}\] are the final kinetic and potential energy.
Complete step by step answer:
We have given here that the particle is given a velocity of \[\sqrt {gl} \] horizontally. Now, the particle will start swinging due to this. So, we have to find the velocity of the particle when the weight of the particle will be equal to the tension in the string. Now, there are two forces acting on the particle vertically one is the centrifugal force and another is the gravitational pull on the particle. Let, the mass of the particle is $m$.

So, the equation of motion of the particle along the wire can be written as, \[T = mg\cos \theta + \dfrac{{m{v^2}}}{l}\]
Now, according to the question this is equal to the weight of the particle hence we can write,
\[mg = mg\cos \theta + \dfrac{{m{v^2}}}{l}\]….(i)
Now, from conservation of mechanical energy we can write,
\[\dfrac{1}{2}m{(\sqrt {gl} )^2} = mgl' + \dfrac{1}{2}m{(v)^2}\]
Now, from the diagram we can see that, \[l' = l - l\cos \theta \].
So, putting this value we have,
\[\dfrac{1}{2}mgl = mgl(1 - \cos \theta ) + \dfrac{1}{2}m{(v)^2}\]
\[\Rightarrow \dfrac{1}{2}mgl - mgl = - mgl\cos \theta + \dfrac{1}{2}m{(v)^2}\]
Upon simplifying we have,
\[gl\cos \theta = \dfrac{1}{2}({v^2} + gl)\]
\[\Rightarrow \cos \theta = \dfrac{1}{{2gl}}({v^2} + gl)\]…….(ii)
Putting these value in equation (i) we have,
\[mg = mg\cos \theta + \dfrac{{m{v^2}}}{l}\]
\[\Rightarrow mg = mg\dfrac{1}{{2gl}}({v^2} + gl) + \dfrac{{m{v^2}}}{l}\]
\[\Rightarrow g = \dfrac{1}{{2l}}({v^2} + gl) + \dfrac{{{v^2}}}{l}\]
Upon simplifying we have,
\[g = \dfrac{{{v^2}}}{{2l}} + \dfrac{g}{2} + \dfrac{{{v^2}}}{l}\]
\[\Rightarrow g - \dfrac{g}{2} = \dfrac{{3{v^2}}}{{2l}}\]
\[\Rightarrow {v^2} = \dfrac{{gl}}{3}\]
\[\therefore v = \sqrt {\dfrac{{gl}}{3}} \]
Hence, the speed of a particle at the point where the tension in the string equals weight of the particle is \[\sqrt {\dfrac{{gl}}{3}} \].
Note: The point at which the particle starts swinging the weight of the particle may seem equal to the tension in the string. But, at the point of starting the particle has acceleration acting in the horizontal direction and since the tension and the weight of the particle is not equal it has some motion along the vertical along with the horizontal line also.
Recently Updated Pages
How many sigma and pi bonds are present in HCequiv class 11 chemistry CBSE
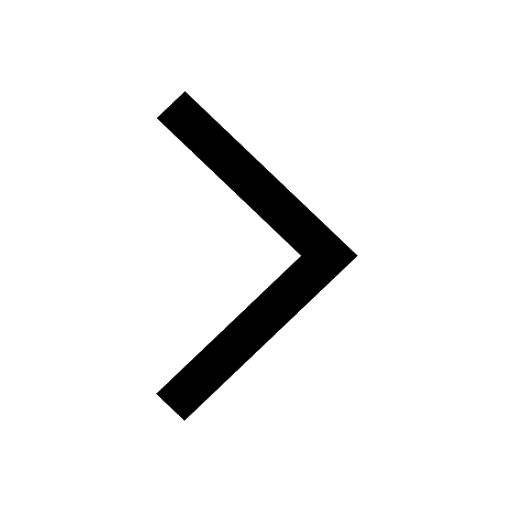
Mark and label the given geoinformation on the outline class 11 social science CBSE
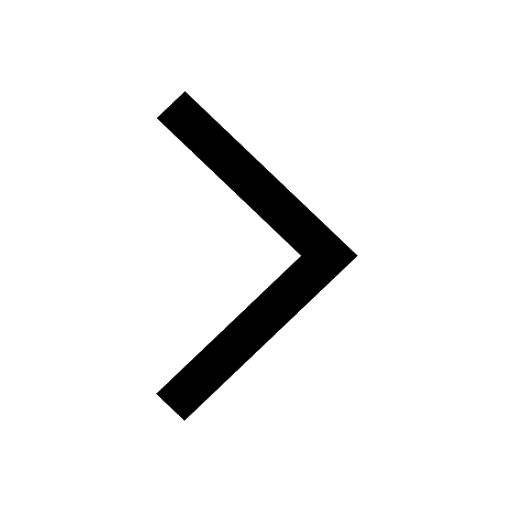
When people say No pun intended what does that mea class 8 english CBSE
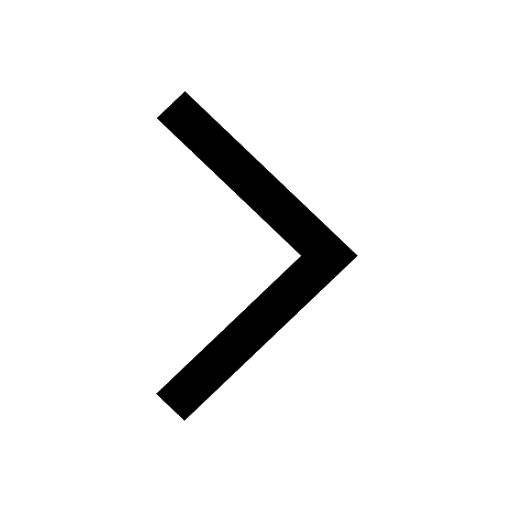
Name the states which share their boundary with Indias class 9 social science CBSE
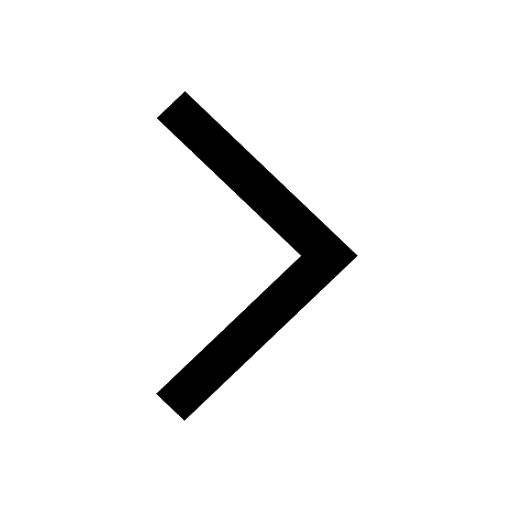
Give an account of the Northern Plains of India class 9 social science CBSE
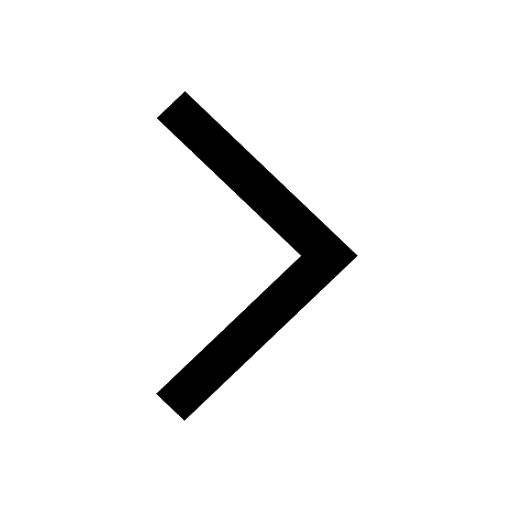
Change the following sentences into negative and interrogative class 10 english CBSE
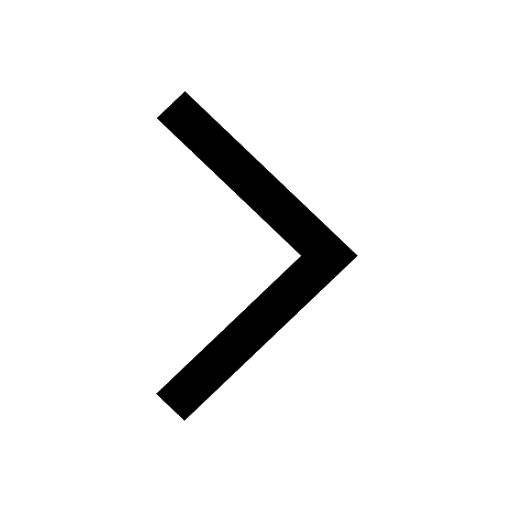
Trending doubts
Fill the blanks with the suitable prepositions 1 The class 9 english CBSE
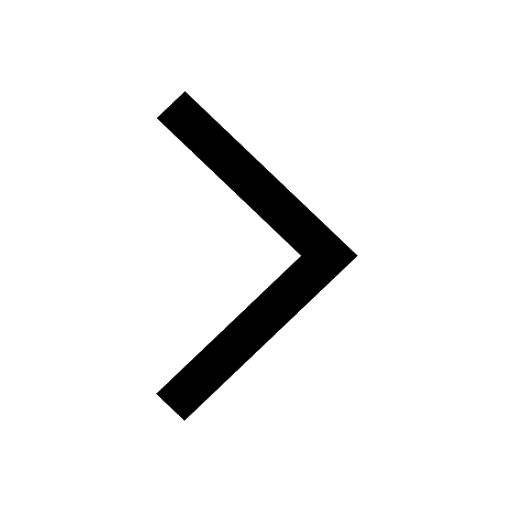
Which are the Top 10 Largest Countries of the World?
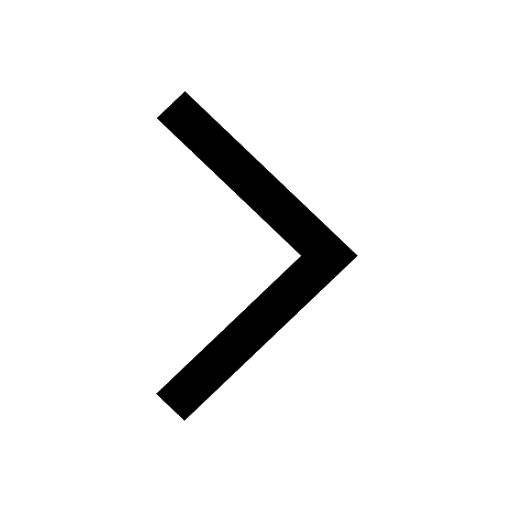
Give 10 examples for herbs , shrubs , climbers , creepers
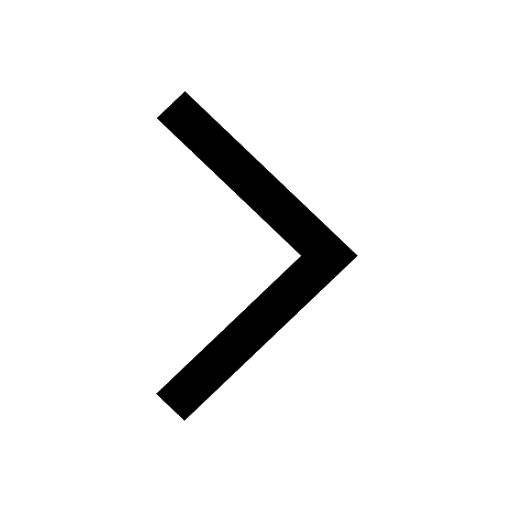
Difference Between Plant Cell and Animal Cell
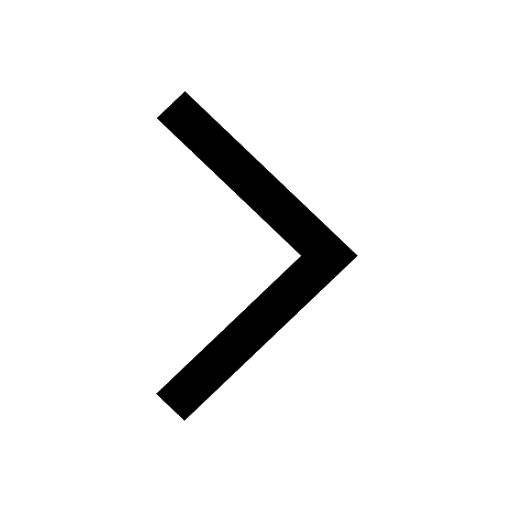
Difference between Prokaryotic cell and Eukaryotic class 11 biology CBSE
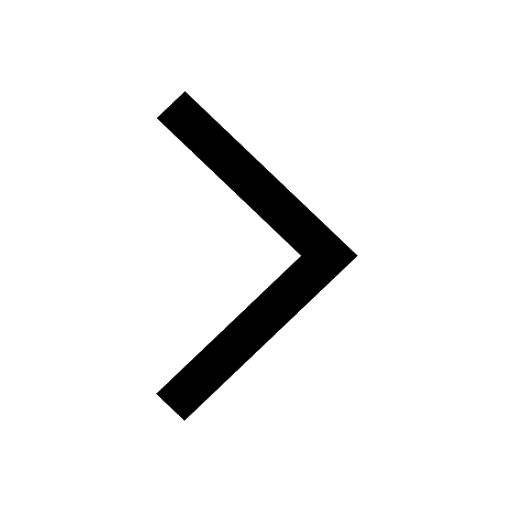
The Equation xxx + 2 is Satisfied when x is Equal to Class 10 Maths
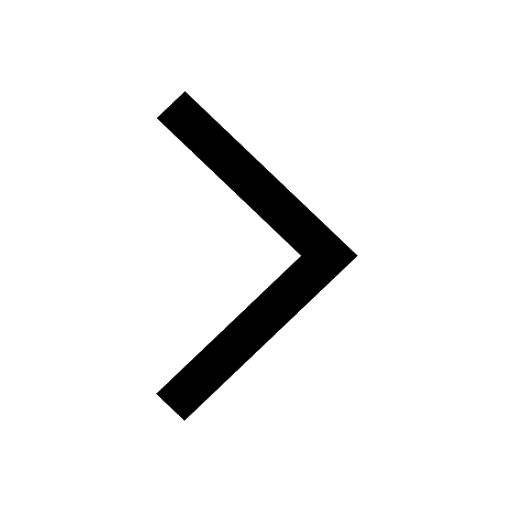
Change the following sentences into negative and interrogative class 10 english CBSE
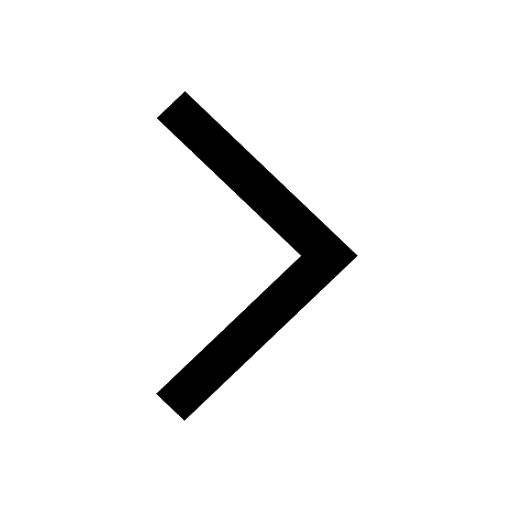
How do you graph the function fx 4x class 9 maths CBSE
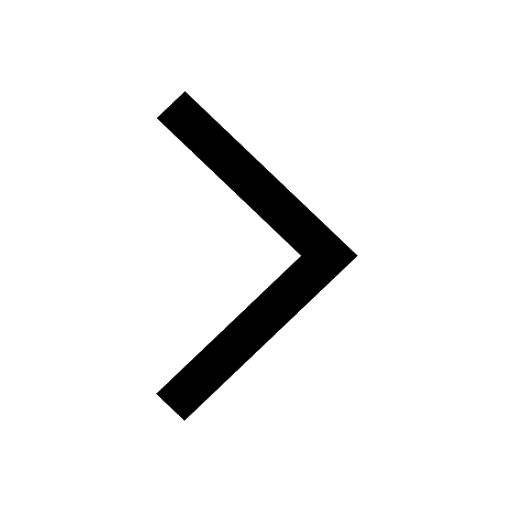
Write a letter to the principal requesting him to grant class 10 english CBSE
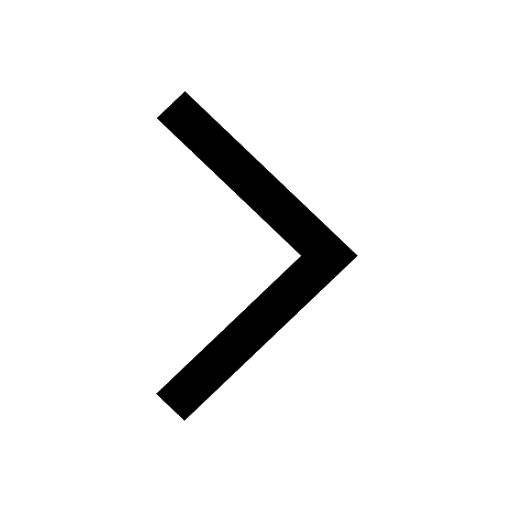